Cultivating our urban forest future: a value-chain perspective
Abstract
For cities to grow their urban forest canopy the formula appears rather straightforward: the right trees, plus the right conditions, plus the right care equals success. These simplified “tree chain of custody” steps, however, represent activities within a complex value-chain in Canada. Given that there is heightened demand for urban tree planting as natural climate solutions become the norm, how can we prepare the value-chain to meet these demands? To answer this question, we outline the pathways by which trees presently go from nurseries into urban and peri-urban areas. Delineating the actors, roles, and present barriers to success exposes the complexity of the process and relationships in the value-chain, as there are distinct phases with multiple actor groups involved who influence, and are influenced, by one another. We explore the issues that pose prominent challenges to, as well as opportunities for, the value-chain. Emergent themes include communication, forecasting demand and timing, underpricing and undervaluing tree establishment, lack of awareness on the importance of soils, juvenile tree health, species selection, and gaps in evidence-based decision support tools. The touchstones of science and innovation, collaboration, and knowledge mobilization are pertinent for the value-chain in Canada to draw upon to navigate the future.
Introduction
Urban forests are of immense value and are often highlighted as a key component of “green infrastructure”. Green infrastructure refers to “an interconnected network of green space that conserves natural ecosystem values and functions and provides associated benefits to human populations” (Benedict and McMahon 2002, p. 12). Trees reduce air pollution and provide oxygen (Zupancic et al. 2015; Leff 2016; Nowak et al. 2016). Trees’ hydrological functions have upsides of improving water filtration, storing water, and lowering stormwater runoff (Bartens et al. 2008; Berland et al. 2017; Leff 2016). Moreover, habitat is provided for wildlife and biodiversity is promoted (Threlfall et al. 2015; Leff 2016; Aronson et al. 2017). Trees also help cities adapt to climate change (Rahman et al. 2015; Brandt et al. 2016; Leff 2016; Sinnett 2020). In addition to the ecological benefits, urban forests offer several advantages to the well-being of individuals who live in cities and peri-urban areas. Urban forests enable an active lifestyle (Mytton et al. 2012; Richardson et al. 2013; Leff 2016)—promoting mental well-being while reducing physical conditions such as blood pressure, obesity, and diabetes (Jiang et al. 2014; Kardan et al. 2015; Ulmer et al. 2016).
At the same time, urban forests in Canada are confronting many challenges, and these will intensify in the future. The 2020 Canadian City Parks Survey reveals that 70% of cities are reporting increased demands for more naturalized spaces and projects, plus there is a growing demand for green infrastructure (Park People 2020). This increasing demand is and will continue to be exacerbated by climate change; heat waves, heavy rainfall, and other extreme weather events are expected to increase in frequency and intensity with negative impacts on urban forests (Johnston 2004; Safford et al. 2013). It is anticipated that climatic changes will also increase susceptibility to insects and disease (Tubby and Webber 2010; Dale and Frank 2017) as well as favor many populations of tree pests and pathogen species normally kept at low levels by cold winter temperatures (Tubby and Webber 2010). None of the above challenges operate in isolation as urban forests are complex systems characterized by interconnections and interdependencies and their basic requirements are different compared with rural conditions (Czaja et al. 2020).
Aggressive tree planting goals to achieve canopy cover growth targets are a common manifestation to overcome the above challenges. As with many other issues of importance to Canadians, the impetus for tree planting comes from governments and nongovernment organizations through a mix of mechanisms. The 2019 mandate letter from Canada’s Prime Minister included three priorities related to urban forestry: (i) operationalize the plan to plant 2 billion trees over the next 10 years, (ii) help cities to expand and diversify urban forests, and (iii) increase the resilience of Canadian urban forests by supporting research and providing access to domestic sources of climate-resilient and genetically diverse trees (Trudeau 2019). It builds upon provincial tree planting programs, such as Forests Ontario’s 50 Million Tree Program (Forests Ontario 2020). Forest management in cities is largely the purview of municipal governments. Considerable variability exists in municipalities throughout Canada in terms of their capacity for forest management, policies, and by-laws. Notwithstanding efforts by governments, the Canadian Urban Forest Strategy (CUFS) sets forth a vision of sustainable, biodiverse, and healthy urban forests that protect and enhance the well-being and prosperity of Canadian communities (Tree Canada 2019a). Most recently, the Government of Canada announced CAN$200 million in investments into the “Natural Infrastructure Fund” that recognizes the importance of local parks, green spaces, and waterfronts as part of our natural infrastructure (Department of Finance Canada 2021).
The burgeoning interest in planting trees and increasing canopy cover is much needed, timely, and laudable. However, it focuses attention on the ultimate end goal and neglects the means to achieve it. We argue for a value-chain approach to urban forestry in Canada and discuss three essential questions to advancing this perspective in scholarship and practice. First, what are the pathways by which trees presently go from nurseries to being planted in urban and peri-urban areas? Second, what issues pose prominent challenges and opportunities to the value-chain? Third, what touchstones can the urban tree value-chain in Canada draw upon to navigate the future?
Pathways
Understanding the urban tree value-chain is essential to meeting growing demand for planting trees in North American cities (Conway and Vander Vecht 2015). The urban tree value-chain (also referred to as a market, supply, or commodity) employs the analogy of a chain to symbolize interconnections among the actors involved in bringing a product from its source to end (Kaplinsky and Morris 2000). Value-chains are networks that operate across levels, encompass the range of activities implemented by individuals and (or) organizations, and concern different divisions and relationships among actors (Kaplinsky and Morris 2000; Ingram et al. 2014; Khalili and Alinezhad 2018). We ground our perspective in recent works like Kapoor (2018), who demonstrated that value-chains are in fact complex interdependent networks that function more like ecosystems rather than simple, linear (linked) chains. While “existing urban forest literature is strongest in its quantification and qualification of the benefits and care of trees…” there is considerably less information available on the value-chain (Vogt et al. 2015, p. 293). Comprehending the decisions made by those engaged in the urban tree value-chain is needed to illuminate if, and how, municipalities are meeting their goals, the ways urban forests may be changing, and broader socio-ecological dynamics (Conway and Vander Vecht 2015).
As urban forestry evolved into a field of professional practice, researchers have started exploring some portions and (or) aspects of the value-chain. Sydnor et al. (2010), for example, sought to better understand the value-chain/interaction between nurseries (suppliers) and urban foresters (planters) regarding desired tree species for planting. Their research revealed differences between these two actor groups in terms of the desired tree species for planting as well as awareness of species availability, largely due to lack of communication and (or) miscommunication. Research by Whittet et al. (2016) revealed similar results in relation to tree/seed supply and demand challenges between suppliers, planters, and government. Increasing attention (see Campbell et al. 2016; Jim 2019) is being directed to knowledge co-production and information sharing. Jim (2019) characterized the connections between value-chain actors as weak and found little interaction or knowledge sharing among researchers, policy-makers and practitioners, which severely impedes progress in urban forestry. Whereas most scholarship addresses a portion and (or) aspect of the value-chain, a notable exception is by Conway and Vander Vecht (2015) who used mixed methods to comprehensively explore the value-chain regarding selecting tree species.
Advancement in understanding the urban forest value-chain comes from Bowen and Jenkins (2021) who used tree planting by municipalities as a departure point and illustrated (Fig. 1) the urban tree value-chain in Ontario in terms of the main actor groups, actions required, and pathways by which they interact. Bowen and Jenkins (2021) highlighted that urban tree planting is comprised of multiple actions for tree planting and maintenance, which are both imperative for successful tree establishment. It is also comprised of multiple pathways: one based on the flow of products and one based on the flow of knowledge and communication. Distinguishing these pathways is necessary, according to Bowen and Jenkins (2021), to recognize their distinct flows. The pathway of products is linear and clearly delineates what each actor is contributing to urban forest establishment. However, the knowledge and communication pathway is not that simple. There are many more connections, they are bidirectional, and may occur separately or in conjunction with the pathway of products. A total of 13 established paths of communication were identified, with many additional paths identified by actor groups to be integral, beneficial, or of interest.
Fig. 1.

Governance of urban forest in Canada is fragmented, multi-faceted, and not comprehensively reflected in value-chain studies to date. Fragmentation largely stems from the dearth of federal or provincial government involvement (Kenney 2003) and largely resides with municipalities (Barker and Kenney 2012). Institutional diversity is observed at the municipal level as exhibited in documents such as “…street tree regulations (called ordinances, bylaws, or other terms, depending on context; Mincey et al. 2013), as well as policies, strategies, and management plans (Davies et al. 2017) and can be complemented by other municipal planning instruments, such as zoning and urban growth regulations (Hill et al. 2010; Mincey et al. 2013)” (Ordóñez et al. 2020, p. 137).
While enabling legislation grows (see Bardekjian 2018) and many cities have developed climate change and (or) urban forest policies to enhance climate resilience that support urban livability (Cheng et al. 2021), the extent of policy effectiveness remains unclear (Cheng et al. 2021). In effort to combat tree/vegetation loss and protect urban greening, local governments have initiated enforcement strategies or programs to protect urban green space, for example, tree planting programs (Phelan et al. 2019) as well as mechanisms to protect trees on private property (Phelan et al. 2019). Conversely, municipal efforts to protect urban trees can also be problematic. For example, municipalities may impose size mandates for trees at planting, despite empirical evidence showing that transplanting large trees are likely to have reduced growth for many years (e.g., Watson 1985). In comparison, “smaller 1- to 3-inch trees, transplanted at the same time, will often equal in size or surpass them before larger trees regain their pre transplanting vigor. In spite of these difficulties, larger trees continue to be transplanted for the immediate advantages they can provide in landscape design” (Watson 1985, p. 37). Restricting plant lists by origin poses another considerable challenge by municipalities and cities. There is a preponderance on native tree species (Conway and Vander Vecht 2015), while only planting these species is often problematic and limits the diversity of plantings (Sjöman et al. 2016). Studies throughout the world (e.g., Sjöman et al. 2010, 2012, 2016; Lévesque et al. 2013, 2014; Hilbert et al. 2019) have found many non-native, noninvasive species are adapted to poor site conditions characteristic of urban settings and underrepresented in urban plantings.
Relatedly, development practices set by municipalities shape urban forests. Land-use planning policies promote intensification within existing urban areas (Jim 2001; Phelan et al. 2019). For example, development is often the source of soil issues that negatively impact the establishment and growth of trees in urban areas (Jim 2013). The cultivation and management of urban forests are influenced by space restrictions on urban sites, resulting in inadequate soil volume needed to reach tree maturity (Jim 2001; Tree Canada 2015). This can result in developers as well as planners ignoring the soil needs of the trees and ultimately shortening their lifespan (Tree Canada 2015). Minimum requirements for soil and tree spacing (e.g., Tree Canada 2015; Cheng et al. 2021) are an example of how this issue is being tackled in Canada. At the same time, significant gaps and discrepancies have been found to exist within Canadian cities, with only three cities (15%) having developed aligned climate change and urban forest policies (Cheng et al. 2021).
Private landowners are another critical linkage in the urban forestry chain, especially as the majority of lands available for new plantings are privately owned (Green Infrastructure Ontario 2015; Conway 2016). Landowners collectively manage a significant portion of the urban forest (Conway 2016), emphasizing the centrality of their role in the management of urban forests. Most trees on private property have been planted, rather than naturally existing through regeneration (Nowak 2012; Pearce et al. 2013; Conway 2016). Reasons why landowners may want trees to be planted include aesthetics, climate change mitigation, shade benefits, and social factors (Conway 2016; Avolio et al. 2018; MacDonald et al. 2020). At the same time, owners of private property have declined trees even when they are free. For example, research by Carmichael and McDonough (2018) revealed nearly one-quarter of eligible people in Detroit declined free street trees due to historical lack of maintenance. Similarly, “in Ontario, Canada, residential property owners reported factoring in maintenance concerns as well as aesthetics in tree planting decisions (Conway 2016)” (MacDonald et al. 2020, p. 2). This demonstrates the need to engage private landowners in tree planting programs in a way that is responsive to their motivations (MacDonald et al. 2020).
This illustrates the need to closely consider interactions between/among value-chain actors. Previously it was assumed that regulations by local authorities did not extend to gardens and trees on private property (Daniel et al. 2016). However, recent studies clarify that “private tree cover is affected not just by the attitudes of individual owners or residents (Kirkpatrick et al. 2013; Pearce et al. 2013), but also are influenced by a range of direct and indirect governance mechanisms (Nasar et al. 2007; Hall 2010a, 2010b; Kirkpatrick et al. 2011)” (Daniel et al. 2016). For example, Daniel et al. (2016) investigated Brisbane’s (Australia) contemporary planning and environment policy and found “urban consolidation” to be a common theme in response to rapid population growth with the scope of direct and indirect regulation of urban trees on private property.
Equally important is consideration of actors absent from the urban forest value-chain. Indigenous Peoples in urban forestry are noticeably absent, despite being recognized as key actors, and the need to work as true partners in shared governance, joint decision-making, collaborative stewardship, and land-use planning is highlighted (Harland 2019). A positive signal in this regard comes from Tree Canada (2019b), which “… established an Aboriginal Engagement Committee to ensure that the organization’s work would include appropriate acknowledgement and increased participation of Indigenous peoples in Tree Canada programs, projects and activities”. Natural Resources Canada’s Canadian Forest Service (CFS) and Tree Canada has subsequently (2019) conducted a research study to better understand the needs and interests of Indigenous communities in relation to urban forestry. Results provide insight into how Indigenous communities perceive and engage in urban forestry projects and how these projects create opportunities for community engagement. Despite these initiatives, there is an apprehensible lack of meaningful engagement with Indigenous people and clear need to learn about and learn with Indigenous sovereignties and ontologies (Cooke 2020).
Prominent challenges and opportunities
With a more fulsome picture of the actors involved as well as the pathways by which they interact, we turn our attention to gleaning insights into the contemporary situation of the urban forest value-chain in Canada. The entry point for this section stems from the recent study by McGrath (2021), who asked actors from across the Canadian value-chain about present challenges and opportunities. Eight salient issues were identified, and we will use this section to discuss how they present both challenges and (or) opportunities drawing upon scholarship and experiences from Canada and beyond.
Geography and size of cities influences urban forestry
In Canada there are four size classes of cities based on population: large urban population centres (100 000 or more), medium population centres (between 30 000 and 99 000), small population centres (between 1000 and 29 000), and rural areas (less than 1000; Statistics Canada 2017). While the practice of urban forestry has largely focused on large centres, small municipalities provide many of the same benefits (e.g., environmental, associated amenities, etc.; Groninger et al. 2002). Small cities also experience similar challenges to those in urban centres, but without the same access to resources, including invasive species, effects of climate change, budget constraints, etc. (Barker and Kenney 2012). Smaller population centres may also have fewer resources (e.g., knowledge of tree maintenance, financial resources, tree inventories, social capital, etc.; Groninger et al. 2002). This lack of resources may worsen challenges and negative effects on small municipalities (Barker and Kenney 2012). Inadequate resources often compel small municipalities to manage urban forests reactively, thus addressing problems as they arise rather than resolving them pre-emptively (Groninger et al. 2002). This can include hazard tree removal, cleanup following storms, disease outbreak, etc. Consistent monitoring is integral to the safety and vitality of a community’s urban forest, but many small municipalities cannot commit a continuous flow of financial and human resources (Maco and McPherson 2003).
Bowen and Jenkins (2021) uncovered many disparities between large and small municipalities in their study in Ontario that corroborates the findings from others, which suggest that smaller cities are limited by their budget and resources. Participants from municipal actor groups highlighted that larger cities have greater ability to ensure tree quality. For instance, they will go to the nursery to inspect and tag trees to be planted by contractor, they inspect stock after purchase by contractor—only quality stock is received—and staff can visit planting sites to delineate planting areas and allocate tree species and size. Large cities also indicated that they require a two-year guarantee by the contactor that they will replace tree if it dies. Comparatively, respondents for smaller municipalities have stated they lack the resources to ensure quality detailed by larger cities and cannot enforce the replacement of trees by contractors if they die within the two-year period.
In Canada, urban forestry is undertaken by municipalities with little involvement from upper-level governments (Barker and Kenney 2012; Tree Canada 2019a). The effectiveness of urban forestry may be harder to gauge, as it is inconsistent and sporadic between municipalities: “a network of relationships, resources, and environmental factors defines urban forests, but urban forestry overall is implemented in an inconsistent manner without significant involvement from a central guiding entity (Kenney 2003)” (Barker and Kenney 2012, p. 119). Therefore, how urban forests are planted and managed is also geographically distinct. Canada’s climate alone makes urban forestry distinctly different across the country resulting in different challenges faced by actor groups. This poses a challenge to diversification and leaves trees and urban forests vulnerable to devastating insect and disease infestations. A particularly salient example is Ophiostoma ulmi or Ceratocystis ulmi, Dutch elm disease, which first hit Eastern Canada, killing off 80%–90% of elms. As the disease moved west, the impact in Prairie provinces, cities, and towns were far greater due to the large prevalence of elms in the west (Tree Canada 2019a).
Communication
Ineffective and (or) nonexistent communication is foundational to several present difficulties identified by actors (e.g., municipalities, nurseries, consultants, landscape contractors, landscape architects etc.) across the Canadian value-chain (Bowen and Jenkins 2021). Jim (2019) identified a disconnection between available and digestible information across research and practice, which has the two-fold implication: researchers exert little influence over the end value-chain/product (e.g., loss of productivity, diminished effectiveness/results, increase costs, etc.) and practitioners are not able to make good use of translating knowledge into practice. A lack of coordination and not sharing information are often the cause of poor performance (Arshinder and Deshmuk 2008), distortions (Simatupang et al. 2002), and greater uncertainty (Yu et al. 2001).
Current urban forestry challenges are complex and cannot be solved by managerial expertise/practitioners alone; similar to other “wicked problems”, communication is paramount to knowledge sharing and understanding across numerous stakeholders and disciplinary boundaries (Ludwig 2001; Campbell et al. 2016). An exciting opportunity exists to bridge the knowledge–practice gap by fostering interactions among researchers and practitioners through communication, mutual learning, and understanding (Sydnor et al. 2010; Campbell et al. 2016; Jim 2019). Information sharing and communication among actors will lead to better decisions and optimization of value-chain dynamics.
Forecasting demand and timing
Actors throughout the Canadian urban forest value-chain identified the challenge of forecasting demand. Municipal budgetary approval processes allow for a lead time consisting of months, which does not align with the several years it takes to finish a tree in the nursery to meet size requirements for municipal contracts. To meet demand, nurseries must take on financial risk through the initiation of contracts with other growers or grow trees on speculation, with no advance contractual obligation or deposit. This risk is exacerbated when growing unproven material as it can lead to wasted time and wasted product, limiting the amount of new material that is introduced into the landscape. Disparities between what urban foresters wish to plant as compared to available stock is a clear and persistent problem in the value-chain (Cutting-Decelle et al. 2007). Sydnor et al.’s (2010) survey of urban foresters in Ohio illustrated the extent of this mismatch, as desirable species were not available from nurseries and other species were in excess; a similar pattern has been found in many other countries (see Moreira da Silva et al. 2016). Cleary, numerous potential constraints exist in the value-chain be it natural, economic, or practical (Moreira da Silva et al. 2016; Whittet et al. 2016).
Tree establishment is misunderstood, underpriced, and undervalued
Tree establishment was repeatedly identified as a challenge and opportunity by actors throughout the Canadian urban forest value-chain (Bowen and Jenkins 2021). Tree establishment concerns the installation and care (maintenance) of trees, which ultimately influences their well-being and success. A systematic review conducted by Roy et al. (2012) revealed that only 15.6% (18 of 115) of papers discussed the problems or costs of urban trees. More recently, Vogt et al. (2015) observed that the cost of maintenance/management (i.e., planting, pruning, removal and treatment), are not well understood particularly with respect to how mismanagement may reduce the benefits that could be gained from trees that receive more optimal care. Unhealthy trees have reduced crown growth and (or) increased mortality, and the economic, social, environmental, and ecological benefits potentially provided, are lost. It is unfortunate that tree care budgets are frequently considered nonessential or less-essential city services compared with other services (police/fire departments, road projects, schools, etc.) (Vogt et al. 2015). However, urban forestry professionals broadly recognize that ongoing maintenance costs are essential to support urban forest health, ecological functions, and associated ecosystem services (Vogt et al. 2015). Unmaintained trees may even become a disservice, such as when heavy/breaking limbs cause safety concerns or accidents (Roman et al. 2021). Current literature on the cost of not maintaining trees is lacking, especially in terms of specific maintenance activities. Except for specific utility arboriculture or pruning cycles, costs of not pruning are rare (see Ryder and Moore 2013 for a noteworthy exception) (Vogt et al. 2015). Evidence is required to determine the true cost and benefits of proper care from installation onward to meet urban forestry goals (Vogt et al. 2015).
Opportunities for the Canadian value-chain here are multi-faceted. There is a need to understanding the true cost as well as the benefits gained from proper installation and care of trees in urban settings. Changing the mindset and expectations of people, from tree planting to establishment, is a simultaneous requirement. Together, this shift in understanding and expectations provides a logical basis to support tree establishment. This idea came forth in recent work by McGrath (2021, p. 5) on the Canadian value-chain in which regular investment through “itemized, multi-year maintenance contracts built into municipal budgets” was expressed as a clear need. This need is not hypothetical as the integration of urban forests into municipal asset management plans is now being explored by some cities (e.g., Regional Municipality of York).
Soils matter for tree establishment and performance
Attention to soils is very important to the Canadian value-chain, further, it was consistently identified as a high-priority research need by the value-chain (McGrath 2021). Developing soil standards for tree establishment and performance, including geographically relevant soil research and specifications were highlighted as pressing needs. While it is well understood that tree establishment and performance in urban settings is often challenging because of poor soil quality (Day and Bassuk 1994; Conlin and van den Driessche 1996; Jim 1998b; De Lucia et al. 2013; Watson et al. 2014; Layman et al. 2016), it is often neglected (Haan et al. 2012; McGrath et al. 2020) as well as understudied. Major gaps in knowledge include soil structure, aggregate stability, bulk density, porosity (Jim 1998a; Puskás and Farsang 2009), soil volume restriction, and soil sealing (Jim 2017; Just et al. 2018). There is also a need to understand long-term effectiveness and consequences related to tree performance in poor-quality soils (Layman et al. 2016). Although research examining the relationship between soil properties and urban tree performance are limited, Scharenbroch and Catania (2012) found soil organic matter, pH, and texture to relate to urban tree performance and Layman et al. (2016) highlighted the connection between healthy soil and improved tree performance. Such research remains generally disconnected from practice, in which “common tree growth limitations have remained entrenched. For decades, they have escaped the attention of managers and continued to suppress tree performance. They account for many cases of poor tree performance, premature decline, tree hazards and wasteful use of resources” (Jim 2019, p. 51).
Efforts by Scharenbroch et al. (2017) to develop a rapid urban site index for assessing the quality of street tree planting sites, to match species tolerances and site qualities, and assess the efficacy of soil management actions are examples of tools being advanced for managers. They explore these factors to understand the connection between urban soil quality and tree performance and provide practitioners with a rapid-assessment tool.
Nurturing juvenile tree health
Actors across the Canadian value-chain expressed concerns regarding the effects of nursery production practices and quality of nursery stock (McGrath 2021). There are multiple dimensions of this challenge and much more research is needed to specifically understand how different tree nursery soil conditions impact transplanting/establishment (Day et al. 2009). Poor root environment negatively influences transplant survival (Hirons and Percival 2011) and quality root systems have been found to lead to failure due to girdling or poor anchorage (Gilman 2013; Hirons and Percival 2011). The influences of container designs on tree root quality while growing in nurseries and the relationship with tree establishment/survival is gaining attention (Day et al. 2009; Gilman et al. 2016; Allen et al. 2017; McGrath et al. 2021). Gilman (2013) also demonstrated that poor tree form can lead to tree damage in storm events and be costlier for cities to manage either through replacements or increased pruning costs.
Opportunities here are several. It is clear that “a number of nursery production practices can influence the establishment of trees. Perhaps of greatest significance is the extent to which the root system can be diminished during transplanting” (Hirons and Percival 2011, p. 54). Root structure/quality needs to be considered early on, during nursery production, to develop healthy structural roots and avoid/mitigate root challenges and defects (Day et al. 2009; McGrath et al. 2021). While a good start is required for trees, greater attention and consistent management of root architecture throughout production is necessary for the long-term success of urban trees (McGrath et al. 2021). Therefore, practices for nurseries to develop high-quality root systems is needed across production timelines (from production to finished stock) and across production types (i.e., bareroot, container-produced, and balled and burlapped) of nursery-produced trees. The development of high-quality root systems is therefore important for the long-term viability of the urban canopy.
Selecting “the correct” tree species
In Canada, making evidence-based tree species selections was identified another high-priority research need (McGrath 2021). Improved availability to support species selections, information on species selections across geographic areas, and matching species to design and site conditions were emergent themes in the research-needs discussions by the Canadian urban tree value-chain (McGrath 2021). Purchasing preferences of tree species may be changing, but this doesn’t necessarily translate into changes in production inventories (Sydnor et al. 2010), as described above in relation to the challenges of communication and forecasting demand. In Canada, a first filter for selecting and assigning plants is by using hardiness zones. Currently, hardiness zones assign plants to geographic locations based on the most northerly and southerly zones in which they can grow. This approach has limitations due to the coarse spatial nature and the relatively unsystematic assignment of plants to zones (McKenney et al. 2007a, 2007b). In contrast, Natural Resources Canada is developing tools to move beyond single hardiness zone maps using a climate profile for each plant species based on extensive distribution data and continent-wide climate models, where species-specific climate envelopes are now available for 130 tree species (Natural Resources Canada 2021). Cultivated varieties as well as native species need to be assessed for tolerance to the environmental conditions that are typical of the urban landscape. Climate change exacerbates the variety and the complexity of landscapes into which trees are planted, making tree selection even more challenging as well as impacting tree species survival (Khan and Conway 2020). There is higher demand for more diverse urban forests, including an abundance of locally native trees, to help combat, mitigate, and adapt to climate changes (Moreira da Silva et al. 2016; Khan and Conway 2020; see also Mesquita et al. 2010; UN 2014; Whittet et al. 2016). Further, selecting tree species for anticipated vulnerability is another challenge facing the value chain.
Invasive insects, plants, and diseases as threats to Canada’s urban forest future are some of the biggest biotic conservation and preservation challenges that are the forefront of knowledge gaps faced by actors in the urban forest value-chain. New approaches for forecasting urban forest vulnerability as a complex of interrelated scenarios is an emerging area of interest with new resources emerging for forest managers (Steenberg et al. 2016a, 2016b). Within these scenarios, understanding the effect of invasive plants, pests and diseases can help manage risk through informed decision-making.
Urban forests tend to be much less diverse than the proposed benchmarks set, particularly at a species level (Kendal et al. 2014). Strategic planning for diversity should be based on the composition of the urban forest based on inventories to help set benchmarks for diversity (Alvey 2006). Diversity is a critical parameter contributing to ecological resilience and is recognized as a form of protection for urban forests against catastrophic losses (Raupp et al. 2006; Laćan and McBride 2008). Santamour (1990) proposed a rule to in aid in planning urban tree diversity planning that states that municipal trees should be comprised of no more than 10% of any species, 20% of any one genus, and 30% of any single family. For those that supply products to the market, understanding and forecasting the numbers of trees needed of different species is important to meet the changing demand.
Recognition that biodiversity is essential for urban forest resilience (Sjöman et al. 2018) affords several interesting opportunities. Species selection in urban areas are constrained by numerous factors (e.g., harsh conditions in urban environment, soil characteristics, direct disturbances, functional criteria, public preferences, etc.), and evaluating species tolerance in these environments should be a priority (Sjöman et al. 2018). Due to the long-lived nature of trees, shifts in urban tree composition requires long-term thinking and planning, especially in relation to adaptation to future climatic conditions (Wood and Dupras 2021). There are numerous existing tree species selection tools that may help practitioners. One approach is to identify plant traits that can help characterise a species’ tolerance to stresses, relevant to the urban environment, thus providing evidence for site fitness. For example, water stress is the main abiotic constraint for trees in urban environments (Sieghardt et al. 2005; Hirons and Thomas 2018) and, in many regions, it is likely to increase under future climate scenarios (Allen et al. 2010). Therefore, a quantitative indication of tree drought tolerance should always be a fundamental consideration in tree selection for urban environments. More recently, Sjöman et al. (2018) studied the drought tolerance of 45 tree species by evaluating the leaf water potential at turgor loss. This work provides practical data on species that are heuristic to urban foresters and highlight the importance of quantitative plant trait data to aid in species selection decisions.
Evidence-based decision-support tools
Informed decision-making is a challenge identified by many actor groups in Canada (McGrath 2021). Decisions regarding urban forests and green spaces were historically “… driven by a mix of esthetic preferences, socially progressive intentions, development patterns, and desired environmental benefits” (Campbell et al. 2016, p. 1262; see also Ricard 2005; Jonnes 2011). Decisions appear to be perceived as being heavily influenced by certain value-chain actors. For instance, landscape architects were consistently perceived by other value-chain actors to be driving the market trends without consultation on feasibility or timelines, including diversifying tree lists for cities, and specifying native trees on their projects (Bowen and Jenkins 2021). Differences in perceptions of reality between actors may be a source of conflict (Arshinder and Deshmuk 2008). Inherent in the above example, and recognized as a more pervasive challenge, is the interdependence of actor decisions along the value-chain (Handayati et al. 2015). While actors have a right to make their own decisions and usually operate in a decentralized manner, the result may be sub-optimal performance of the entire value-chain (Yu et al. 2001; Arshinder and Deshmuk 2008).
Several research studies have identified the opportunity to strengthen the value chain through evidence-based tools and not rely on current practices or history of use. Campbell et al. (2016) stated that a robust basis of evidence will ultimately influence decision-making in a more effective way than relying on perceptions and preferences along the value-chain. While Martin et al. (2014) argued that in urban forestry, conservation and management decisions, or recommendations on the fate of individual trees, require an evidence base. This is further supported in the recent work by McGrath (2021) where value-chain actors (e.g., landscape architects, soil suppliers, consultants and contractors) similarly articulated the need for evidenced-based resources to support practice including soil specifications, soil standards, and installation practices. Additional knowledge identified includes quantitative metrics of tree-level characteristics (i.e., species, age, and tree size), objective and repeatable evaluations of tree condition and maintenance, and importance of urban trees at different spatial scales of urban forests (i.e., across land use types, municipalities, neighborhoods, etc.) (Moore 1999; Martin et al. 2014).
Navigating the future
Complexity, uncertainty, and change lie ahead for urban forests in Canada. Resilience is required if the urban forestry value-chain is to address contemporary challenges, realize present opportunities, and successfully navigate the future. Resilience involves the ability of a system to persist, adapt, and transform (Folke 2006, 2016; Walker and Salt 2006). In this section we highlight three key touchstones for a resilient urban forestry value-chain in Canada—science and innovation, collaboration, and knowledge mobilization—and discuss the need for novel approaches to programs that bring them together.
Science and innovation
Urban forestry is, by necessity, multi-disciplinary (Konijnendijk et al. 2006). Vogt (2018) employed the analogy of “ships that pass in the night” to question if urban greening has a disciplinary crosstalk problem. Most recently, urban forestry has been defined as “…the transdisciplinary science and practice of planting, maintaining, restoring, preserving, and stewarding trees, forests, greenspace, and natural areas in cities” (Vogt et al. In press; see also Vogt 2017). The actors constituting the urban forest value-chain importantly possess different knowledge (Vogt et al. 2016), and in some instances distinct knowledge systems. Fostering synergies across knowledge systems and catalyzing opportunities for knowledge co-production are recognized ways to generate new insights and innovations in relation to ecosystems (e.g., Tengö et al. 2014; Djenontin and Meadow 2018; Norström et al. 2020).
A rich repository of forestry science exists. The same cannot be said for urban forestry research and theory, although there are notable differences between Europe and North America (see Konijnendijk et al. 2006). The fundamental gaps in our understanding of urban forest ecosystems are a consequence of their “novelty”. Novel ecosystems by definition present a departure from historic assemblages, functioning of biotic components, and abiotic conditions and are therefore distinct from the original characteristics of ecosystems (Hobbs et al. 2009). Where we try to extrapolate, or directly apply theory, from forest ecosystems we discount the biotic and abiotic variations characteristic of urban ecosystems. Sound science of urban forests is urgently needed and underpins the value-chain approach.
In addition to the important innovations that will come from new knowledge, strengthening the connection between the science practice–policy interface is an important tenant for navigating the future. Rigorous science is essential to informing urban forestry practices and governance. However, practitioners and decision-makers are often disconnected from scientific evidence. “Plugging” the gaps in the knowledge–practice continuum is necessary to create and deploy data-driven approaches that will be the foundation of effective urban forest design (i.e., the intractable problem of urban soils sensu Jim 2019).
Collaboration
Taking a value-chain approach to urban forestry emphasizes collaboration and builds upon the collective capacity of the network. In this regard, we ground our perspective with the work of Gray (1989), who described collaboration as the sharing of power among parties to define a problem and construct solutions in the environmental domain (see also Purdy et al. 2018).
Collaborative action has long been garnering attention as a distinct approach to environmental management (e.g., Ostrom 1991; Selin and Chavez 1995; Daniels and Walker 1996). In looking forward, the co-operative management model responds to devolution of responsibility and supplements conventional decision-making structures (Meadowcroft 1998; Armitage et al. 2012). Moreover, it is highlighted as an important innovation to navigating contemporary circumstances of complexity, uncertainty, and change (e.g., Folke et al. 2005; Armitage et al. 2009; Bodin 2017).
Knowledge co-production in urban forestry is an enabling mechanism for success. The blending of roles between researchers and practitioners and among actors facilitates capacity building and creates trust (Campbell et al. 2016). In fostering collaborative relationships and building the underused or undeveloped communication channels the value-chain can create greater understanding of actor roles and responsibilities. In so doing, prospects are elevated for ensuring that emergent solutions fully address the challenges identified by actors. Moreover, collaboration increases the frequency and quality of communication and moves from merely transactional interactions to co-generation of solutions (Österle and Otto 2010).
Collaborative approaches in urban forestry help to break down barriers among actor groups and ensure that outcomes truly reflect the needs of the actors while being reflective of their communities (Locke et al. 2010). Further, with respect to urban forest cover and access to quality urban greening spaces, there are significant social inequities, particularly race-based inequity (Watkins and Gerrish 2018). The shift to more inclusive frameworks (e.g., the “All Lands, All People” approach from Grove 2009) where inclusivity is actively cultivated is important. When common opportunities or goals are defined, for example strategically defining canopy cover increase goals, coordinated collaborative approaches can inform and strengthen sustainable decision-making (Locke et al. 2013).
Knowledge mobilization
Knowledge mobilization is a growing imperative. Strengthening the connection among the value-chain actors and between research and practice is increasingly emphasized internationally (e.g., Bennet and Bennet 2007; Hammersley-Fletcher and Lewin 2015; Coldwell et al. 2017; Malin and Brown 2020). Similarly, efforts for evidence-informed policy and practice based on real-world knowledge are being strongly encouraged (Nutley et al. 2007), with particular prominence to address global pressing issues (Bennet and Bennet 2007). While knowledge mobilization is variously defined (see Levin 2008 for summary), we employ it as “a range of active approaches deployed to encourage the creation and sharing of research-informed knowledge” (Davies et al. 2015, p. 2). In this overarching sense, it encompasses knowledge transfer, utilization, dissemination, and so on. Effective knowledge mobilization involves reciprocal and complementary flow/uptake of research knowledge between actors. Active engagement, which meaningfully involves researchers as well as all potential information users, is essential for this bidirectional process (Bennet and Bennet 2007).
In urban forestry, the breadth of topics and disciplines can present obstacles preventing the successful translation of knowledge to practice. Jim (2019) presented the example of soil science education in urban forestry or arboriculture, where gaps in curricula may not develop “soil awareness” that would allow practitioners to understand relevant references. The complex network of stakeholders that comprise the value-chain may have different expectations, approaches, and understandings (Ugolini et al. 2015). Fostering cross-disciplinary learning opportunities as well as enhancing education and training programs (college, university, and continuing education) in urban forestry will expand the knowledge base and strengthens the value-chain (adapted from Bowen and Jenkins 2021). Developing content and creating accessible, solutions-oriented knowledge transfer events or tools, would help to address the current constraints of access to knowledge that the value-chain experiences (Ugolini et al. 2015; McGrath 2021). Knowledge mobilization facilitates information sharing and learning, which ultimately enhances the capacity for an actor to leverage knowledge in a manner that is actionable and produces value (Bennet and Bennet 2007).
The need for novelty
Novel approaches are required for navigating the future. It is imperative to develop urban forestry programs that match the needs of the value-chain (Ugolini et al. 2015), and those are largely reflected in the seven emerging themes presented above. However, catalyzing a resilient urban forestry value-chain in Canada necessitates an approach that also meaningfully brings together science and innovation, collaboration, and knowledge mobilization. Programs that address the needs of the urban forestry value-chain through such an approach are scarce.
Given the impetus outlined in this perspective, we draw attention to consortium research as a model typology and novel approach for the urban forestry value chain. The consortium research approach (sensu Österle and Otto 2010) refers to programs or projects in which a number of partners and (or) companies together with researchers address common research topics. The purpose of the consortium research approach is to undertake science and innovation, promote collaboration between practitioners and researchers, and facilitate knowledge mobilization among the actors in the network. There are defined phases in consortium research: analysis, design, evaluation, and diffusion. Consortium research by its nature is an iterative process; therefore, as new problems emerge within and among the value-chain, the process allows for the redefinition of priorities as part of the feedback loop, helping the value-chain to navigate the future.
Consortium research is proving extremely valuable as it allows companies to tackle questions collaboratively that are too expensive or risky to explore alone (e.g., the Canadian Traumatic Brain Injury Research Consortium (cf Hutchison et al. 2018) and drug discovery (cf Simpson and Wilkinson 2020)). It is worthwhile to note that consortium research is not a panacea. There are many circumstances in which the consortium research approach can become either more or less suitable. A key consideration of the suitability is the competitive nature of the research portfolio and associated expectations from the network, where consortium research lends itself particularly well to precompetitive research scenarios (Wagner et al. 2010).
Although such programs are scarce in urban forestry, the recent launch of the Greening the Landscape Research Consortium (see Vineland Research & Innovative Centre 2021a) illustrates the potential of new programs through this approach. This program aims to “build the collective capacity of the Canadian urban tree value-chain by developing and mobilizing scientific knowledge within our collaborative network” (Vineland Research & Innovative Centre 2021b, p. 1). It is comprised of members from across the value-chain representing the various actor groups (e.g., nurseries, landscape contractors, soil suppliers, arborists, municipalities). The strategic plan of the program is based on the pillars of science and innovation, collaboration, knowledge mobilization, and capacity building. To this end, members help to define the priority areas and work with the research team to define needs and opportunities that are grounded in real-world scenarios using case studies to support direct transfer of findings to the collaborative network. While only recently launched, approaches like this demonstrate the promise of novel programs to support resilient urban forestry value-chain in Canada.
Conclusion
The opening section of this paper highlights the myriad of challenges confronting urban forests in Canada and opportunities for their enhancement. The desire to overcome present challenges as well as respond to climate change has led to much enthusiasm about trees and green spaces in cities (Lee et al. 2015; Mengbing and Zhang 2020) and an array of government and nongovernment mechanisms to this end.
Urban forests are complex systems characterized by interconnections and interdependencies—no part operates in isolation (Czaja et al. 2020). It is therefore imperative to understand the urban tree value-chain (Conway and Vander Vecht 2015). A value-chain perspective illuminates the pathways from source to end, reveals salient contemporary issues such as the seven areas of opportunity explored herein, and affords an opportunity to glean key touchstones of science and innovation, collaboration, and knowledge mobilization to navigate the future. Ultimately, achieving canopy cover goals through aggressive tree planting hinges on the effectiveness and efficiency of the urban forest value-chain.
References
Allen CD, Macalady AK, Chenchouni H, Bachelet D, McDowell N, Vennetier M, et al. 2010. A global overview of drought and heat-induced tree mortality reveals emerging climate change risks for forests. Forest Ecology Management, 259(4): 660–684.
Allen KS, Harper RW, Bayer A, and Brazee NJ. 2017. A review of nursery production systems and their influence on urban tree survival. Urban Forestry & Urban Greening, 21: 183–191.
Alvey AA. 2006. Promoting and preserving biodiversity in the urban forest. Urban forestry & urban greening, 5(4): 195–201.
Armitage D, De Loë R, and Plummer R. 2012. Environmental governance and its implications for conservation practice. Conservation Letters, 5(4): 245–255.
Armitage DR, Plummer R, Berkes F, Arthur RI, Charles AT, Davidson-Hunt IJ, et al. 2009. Adaptive co-management for social–ecological complexity. Frontiers in Ecology and the Environment, 7(2): 95–102.
Aronson M, Christopher FJ, Lepczyk A, Evans KL, Goddard MA, Lerman SB, et al. 2017. Biodiversity in the city: key challenges for urban green space management. Frontiers in Ecology and the Environment, 15(4): 189–196.
Arshinder, KA, and Deshmuk SG. 2008. Supply chain coordination: perspectives, empirical studies and research directions. International Journal of Production Economics, 115(2): 316–335.
Avolio ML, Pataki DE, Trammell TLE, and Endter-Wada J. 2018. Biodiverse cities: the nursery industry, homeowners, and neighborhood differences drive urban tree composition. Ecological Monographs, 88(2): 259–276.
Bardekjian A. 2018. Compendium of best urban forest management practices. 2nd Edition. Originally commissioned to Tree Canada by Natural Resources Canada. [online]: Available from treecanada.ca/resources/canadian-urban-forest-compendium/.
Barker EJ, and Kenney WA. 2012. Urban forest management in small Ontario municipalities. The Forestry Chronicle, 88(2): 118–123.
Bartens J, Day SD, Harris JR, Dove JE, and Wynne TM. 2008. Can urban tree roots improve infiltration through compacted subsoils for stormwater management? Journal of Environmental Quality Abstract – Bioremediation and Biodegradation, 37(6): 2048–2057.
Benedict MA, and McMahon ET. 2002. Green infrastructure: smart conservation for the 21st century. Renewable Resources Journal, 20(3): 12–17.
Bennet A, and Bennet DH. 2007. Knowledge mobilization in the social sciences and humanities: moving from research to action. 1st edition. MQI Press, Frost, West Virginia. 180 p.
Berland A, Shiflett SA, Shuster WD, Garmestani AS, Goddard HC, Herrmann D, et al. 2017. The role of trees in urban stormwater management. Landscape and Urban Planning, 162: 167–177.
Bodin Ö. 2017. Collaborative environmental governance: achieving collective action in social- ecological systems. Science, 357(6352): eaan1114.
Bowen A, and Jenkins A. 2021. Mapping the urban tree value-chain: qualitative interviews of the Ontario industry. [online]: Available from vinelandresearch.com/wp-content/uploads/2020/02/Mapping-the-Urban-Tree-Value-chain.pdf.
Brandt L, Derby Lewis A, Fahey R, Scott L, Darling L, and Swanston C. 2016. A framework for adapting urban forests to climate change. Environmental Science & Policy, 66: 393–402.
Campbell LK, Svendsen ES, and Roman LA. 2016. Knowledge co-production at the research- practice interface: embedded case studies from urban forestry. Environmental Management, 57: 1262–1280.
Carmichael C, and McDonough M. 2018. The trouble with trees? Social and political dynamics of street tree-planting efforts in Detroit, Michigan, USA. Urban Forestry & Urban Greening, 31: 221–229.
Cheng Z, Nitoslawski S, van den Bosch CK, Sheppard S, Nesbitt L, and Girling C. 2021. Alignment of municipal climate change and urban forestry policies: a Canadian perspective. Environmental Science & Policy, 122: 14–24.
Coldwell M, Greany T, Higgins S, Brown C, Maxwell B, Stiell B, et al. 2017. Evidence-informed teaching: an evaluation of progress in England. London: Department for Education, Research Report. 74 p.
Conlin TSS, and van den Driessche R. 1996. Short-term effects of soil compaction on growth of Pinus contorta seedlings. Canadian Journal of Forest Research, 26(5): 727–739.
Conway TM. 2016. Tending their urban forest: residents’ motivations for tree planting and removal. Urban Forestry & Urban Greening, 17: 23–32.
Conway TM, and Vander Vecht J. 2015. Growing a diverse urban forest: species selection decisions by practitioners planting and supplying trees. Landscape and Urban Planning, 138: 1–10.
Cooke B. 2020. The politics of urban greening: an introduction. Australian Geographer, 51(2): 137–153.
Cutting-Decelle AF, Young BI, Das BP, Case K, Rahimifard S, Anumba CI, et al. 2007. A review of approaches to supply chain communications: from manufacturing to construction. Journal of Information Technology in Construction, 12: 73–102. [online]: Available from itcon.org/2007/5.
Czaja M, Kolton A, and Muras P. 2020. The complex issue of urban trees-stress factor accumulation and ecological service possibilities. Forests, 11: 932.
Dale AG, and Frank SD. 2017. Warming and drought combine to increase pest insect fitness on urban trees. PLoS ONE 12(3): e0173844.
Daniel C, Morrison TH, and Phinn S. 2016. The governance of private residential land in cities and spatial effects of tree cover. Environmental Science and Policy, 62: 79–89.
Daniels SE, and Walker GB. 1996. Collaborative learning: improving public deliberation in ecosystem-based management. Environmental Impact Assessment Review, 16(2): 71–102.
Davies HJ, Doick KJ, Hudson MD, and Schreckemberg K. 2017. Challenges for tree officers to enhance the provision of regulating ecosystem services from urban forests. Environmental Research, 156: 97–107.
Davies HT, Powell AE, and Nutley SM. 2015. Mobilising knowledge to improve UK health care: learning from other countries and other sectors: a multimethod mapping study. Health Services and Delivery Research, 3(27): 1–185.
Day SD, and Bassuk NL. 1994. A review of the effects of soil compaction and amelioration techniques on landscape trees. Journal of Arboriculture, 20: 9–27.
Day SD, Watson G, Wiseman PE, and Harris JR. 2009. Causes and consequences of deep structural roots in urban trees: from nursery production to landscape establishment. Arboriculture & Urban Forestry, 35(4): 182–191.
De Lucia B, Cristiano G, Vecchietti L, and Bruno L. 2013. Effect of different rates of composted organic amendment on urban soil properties, growth and nutrient status of three Mediterranean native hedge species. Urban Forestry & Urban Greening, 12(4): 537–545.
Department of Finance Canada. 2021. Budget 2021 a healthy environment for a healthy economy [online]: Available from canada.ca/en/department-finance/news/2021/04/budget-2021-a-healthy-environment-for-a-healthy-economy.html.
Djenontin INS, and Meadow AM. 2018. The art of co-production of knowledge in environmental sciences and management: lessons from international practice. Environmental Management, 61(6): 885–903.
Folke C. 2006. Resilience: the emergence of a perspective for social–ecological systems analyses. Global Environmental Change, 16(3): 253–267.
Folke C. 2016. Resilience (republished). Ecology and Society, 21(4): 44.
Folke C, Hahn T, Olsson P, and Norberg J. 2005. Adaptive governance of social-ecological systems. Annual Review of Environment and Resources, 30: 441–473.
Forests Ontario. 2020. Forests Ontario’s 50 million tree program fact sheet [online]: Available from assets.ctfassets.net/e09p19lzfrfe/2XOk57YgzS3Uip5mVgC5V2/4ae230ea497b552936b98388c7e20c04/FO_50MTP_FACTSHEET_Dec_2020_final_.pdf.
Gilman EF. 2013. Anchorage influence by production method and root pruning. Arboriculture & Urban Forestry, 39(1): 1–5.
Gilman EF, Paz M, and Harchick C. 2016. Effect of container type and root pruning on growth and anchorage after planting Acer rubrum L. into landscape soil. Arboriculture & Urban Forestry, 42(2): 73–83.
Gray B. 1989. Collaborating: finding common ground for multiparty problems. Jossey-Bass, San Francisco, California. 358 p.
Green Infrastructure Ontario. 2015. Ontario’s urban forests: call to action. [online]: Available from greeninfrastructureontario.org/app/uploads/2016/07/GIO_Urban_Forest_Call_to_Action_Sept15Print.pdf.
Groninger JW, Close DD, and Basman CM. 2002. Can small, rural communities practice urban forestry? Journal of Forestry, 100: 23–28.
Grove JM. 2009. Cities: managing densely settled social-ecological systems. In Principles of ecosystem stewardship. Edited by C Folke, GP Kofinas, and FS Chapin, III. Springer, New York, New York. pp. 281–295.
Haan NL, Hunter MR, and Hunter MD. 2012. Investigating predictors of plant establishment during roadside restoration. Restoration Ecology, 20(3): 315–332.
Hall T. 2010a. Goodbye to the backyard?—The minimisation of private open space in the Australian outer-Suburban Estate. Urban Policy and Research, 28(4): 411–433.
Hall T. 2010b. The life and death of the Australian backyard. CSIRO Publishing, Melbourne.
Hammersley-Fletcher L, and Lewin C. 2015. Evidence-based teaching: advancing capability and capacity for enquiry in schools: interim report. Manchester, UK: National College for Teaching and Leadership, Interim Report. 35 p.
Handayati Y, Simatupang TM, and Perdana T. 2015. Value co-creation in agri-chains network: an agent-based simulation. Procedia Manufacturing, 4: 419–428.
Harland K. 2019. Genomics and society: forest-dependent communities. The Forestry Value Chain: Stakeholder Engagement and Strategy Session. Vancouver, BC. [online]: Available from genomequebec.com/DATA/TEXTEDOC/20191127%20Forestry%20Value%20Chain%20report%20and%20supporting%20documents.pdf.
Hill E, Dorfman JH, and Kramer E. 2010. Evaluating the impact of government land use policies on tree canopy coverage. Land Use Policy, 27(2): 407–414.
Hilbert DR, Koeser AK, Roman LA, Hamilton K, Landry SM, Hauer RJ, et al. 2019. Development practices and ordinances predict inner-city variation in Florida urban tree canopy coverage. Landscape and Urban Planning, 190: 103603.
Hirons A, and Percival, G. 2011. Fundamentals of tree establishment: a review. Conference: Trees, People and the Built Environment. Birmingham, UK. [online]: Available from researchgate.net/publication/274953179_Fundamentals_of_tree_establishment_a_review.
Hirons AD, and Thomas PA. 2018. Applied tree biology. Wiley-Blackwell, Chichester. 432 p.
Hobbs RJ, Higgs E, and Harris JA. 2009. Novel ecosystems: implications for conservation and restoration. Trends in Ecology & Evolution, 24(11): 599–605.
Hutchison JS, Emery C, Gagnon I, Léger C, Riopelle R, Wellington C, et al. 2018. The Canadian Traumatic brain injury research consortium: epitomizing collaborative research in Canada. Journal of Neurotrauma, 35(16): 1858–1863.
Ingram V, Levang P, Cronkleton P, Degrande A, Leakey R, and van Damme P. 2014. Forest and tree product value chains. Forests Trees and Livelihoods, 23(1–2): 1–5.
Jiang B, Chang CY, and Sullivan WC. 2014. A dose of nature: tree cover, stress reduction and gender differences. Landscape and Urban Planning, 132: 26–36.
Jim CY 1998a. Physical and chemical properties of a Hong Kong roadside soil in relation to urban tree growth. Urban Ecosystems, 2: 171–181.
Jim CY. 1998b. Urban soil characteristics and limitations for landscape planting in Hong Kong. Landscape and Urban Planning, 40(4): 235–249.
Jim CY. 2001. Managing urban trees and their soil envelopes in a contiguously developed city environment. Environmental Management, 28(6): 819–832.
Jim CY. 2013. Sustainable urban greening strategies for compact cities in developing and developed economies. Urban Ecosystems, 16: 441–761.
Jim CY. 2017. Constraints to urban trees and their remedies in the built environment. In Routledge handbook of urban forestry. Edited by F Ferrini, BC Van den Konijnendijk, and A Fini. Routledge, London. pp. 273–290.
Jim CY. 2019. Resolving intractable soil constraints in urban forestry through research-practice synergy. Socio-Ecological Practice Research, 1: 41–53.
Johnston M. 2004. Impacts and adaptation for climate change in urban forests. Proceedings of 6th Canadian Urban Forest Conference, Kelowna, B.C.
Jonnes J. 2011. What is a tree worth? Wilson Quarterly, 34–41.
Just MG, Frank SD, and Dale AG. 2018. Impervious surface thresholds for urban tree site selection. Urban Forestry & Urban Greening, 34: 141–146.
Kardan O, Gozdyra P, Misic B, Moola F, Palmer LJ, Paus T, et al. 2015. Neighborhood greenspace and health in a large urban center. Scientific Reports, 5: 11610.
Kaplinsky R, and Morris M. 2000. A handbook for value chain research. Canada: IDRC.
Kapoor, R. 2018. Ecosystems: broadening the locus of value creation. Journal of Organization Design, 7(12).
Kendal D, Dobbs C, and Lohr VI. 2014. Global patterns of diversity in the urban forest: is there evidence to support the 10/20/30 rule? Urban Forestry & Urban Greening, 13(3): 411–417.
Kenney WA. 2003. A strategy for Canada’s urban forests. The Forestry Chronicle, 79(4): 785–789.
Khalili J, and Alinezhad A. 2018. Performance evaluation in green supply chain using BSC, SEA, and data mining. IJSOM, 5(2): 182–191.
Khan T, and Conway TM. 2020. Vulnerability of common urban forest species to projected climate change and practitioners perceptions and responses. Environmental Management, 65: 534–547.
Kirkpatrick JB, Daniels GD, and Davison A. 2011. Temporal and spatial variation in garden and street trees in six eastern Australian cities. Landscape and Urban Planning, 101(3): 244–252.
Kirkpatrick JB, Davison A, and Daniels GD. 2013. Sinners, scapegoats or fashion victims? Understanding the deaths of trees in the green city. Geoforum, 48: 165–176.
Konijnendijk CC, Ricard RM, Kenney A, and Randrup TB. (2006). Defining urban forestry-A comparative perspective of North America and Europe. Urban Forestry & Urban Greening, 4(3–4): 93–103.
Laćan I, and McBride JR. 2008. Pest Vulnerability Matrix (PVM): a graphic model for assessing the interaction between tree species diversity and urban forest susceptibility to insects and diseases. Urban Forestry & Urban Greening, 7(4): 291–300.
Layman RM, Day SD, Mitchell DK, Chen Y, Harris JR, and Daniels WL. 2016. Below ground matters: urban soil rehabilitation increases tree canopy and speeds establishment. Urban Forestry & Urban Greening, 16: 25–35.
Lee AC, Jordan HC, and Horsley J. 2015. Value of urban green spaces in promoting healthy living and wellbeing: prospects for planning. Risk Management and Healthcare Policy, 8: 131–137.
Leff M. 2016. The sustainable urban forest: a step-by-step approach. Davey Institute. [online]: Available from mostcenter.umd.edu/sites/default/files/2020-08/SustainableUrbanForestGuide.pdf.
Lévesque M, Rigling A, Bugmann H, Weber P, and Brang P. 2014. Growth response of five co- occurring conifers to drought across a wide climatic gradient in Central Europe. Agricultural and Forest Meteorology, 197: 1–12.
Lévesque M, Saurer M, Siegwolf R, Eilmann B, Brang P, Bugmann H, et al. 2013. Drought response of five conifer species under contrasting water availability suggest high vulnerability of Norway spruce and European larch. Global Change Biology, 19(1): 3184–3199.
Levin B. 2008. Thinking about knowledge mobilization: a discussion paper prepared at the request of the Canadian Council on Learning and the Social Sciences and Humanities Research Council. [online]: Available from sshrc-crsh.gc.ca/about-au_sujet/publications/KMb_-_LevinDiscussionPaper_-_E.pdf.
Locke DH, Grove M, Galvin MF, O’Neil-Dunne J, and Murphy C. 2013. Applications of urban tree canopy assessment and prioritization tools: supporting collaborative decision making to achieve urban sustainability goals. Cities and the Environment, 6(1): 7.
Locke DH, Grove MJ, Lu, JWT, Troy A, O’Neil-Dunne JPM, and Beck BD. 2010. Prioritizing preferable locations for increasing urban tree canopy in New York City. Cities and the Environment, 3(1): 18.
Ludwig D. 2001. The era of management is over. Ecosystems, 4(8): 758–764.
MacDonald H, McKenney D, McLaven K, and Perry S. 2020. Realizing expectations from planting trees on private land in Ontario, Canada. Landscape Online, 78: 1–9.
Maco SE, and McPherson EG. 2003. A practical approach to assessing structure, function, and value of street tree populations in small communities. Journal of Arboriculture, 29(2): 84–97.
Malin JR, and Brown C. 2020. Joining worlds: knowledge mobilization and evidence informed practice. In The role of knowledge brokers in education: connecting the dots between research and practice. Edited by JR Malin, and C Brown. Routledge, New York. pp. 1–12.
Martin AR, Davies WE, Puric-Mladenovic D, and Smith SM. 2014. Is evidence-based conservation applied in urban forestry? A case study from Toronto, Canada. Open Journal of Forestry, 4(1): 28–33.
McGrath D. 2021. Urban tree value-chain research priority setting workshop: report on the findings. [online]: Available from vinelandresearch.com/wp-content/uploads/2020/02/Urban-Tree-Value-Chain-Research-Priority-Setting-Workshop- Report.pdf.
McGrath D, Henry J, Munroe R, and Williams C. 2020. Compost improves soil properties and tree establishment along highway roadsides. Urban Forestry & Urban Greening, 55: 126851.
McGrath D, Henry J, Munroe R, and Williams C. 2021. From propagation to field: influence of tray design on tree seedling quality and performance. Journal of Environmental Horticulture, 39(1): 33–40.
McKenney DW, Pedlar JH, Lawrence K, Campbell K, and Hutchinson MF. 2007a. Beyond traditional hardiness zones: using climate envelopes to map plant range limits. BioScience, 57(11): 929–937.
McKenney DW, Pedlar JH, Lawrence K, Campbell K, and Hutchinson MF. 2007b. Potential Impacts of Climate Change on the Distribution of North American Trees. BioScience, 57(11): 939–948.
Meadowcroft J. 1998. Co-operative management regimes: a way forward? In Co-operative Environmental Governance. Edited by P Glasbergen. Springer, Dordrecht. pp. 21–42.
Mengbing D, and Zhang X. 2020. Urban greening: a new paradox of economic or social sustainability? Land Use Policy, 92: 104487.
Mesquita CAB, Holvorcem CGD, Lyrio CH, and Dimas De Menezes P. 2010. COOPLANTAR: a Brazilian initiative to integrate forest restoration with job and income generation in rural areas. Ecological Restoration, 28(2): 199–207. [online]: Available from jstor.org/stable/43443232.
Mincey SK, Hutten M, Fischer BC, Evans TP, Stewart SI, and Vogt JM. 2013. Structuring institutional analysis for urban ecosystems: a key to sustainable urban forest management. Urban Ecosystems, 16: 553–571.
Moore W. 1999. The combined use of the resistograph and the shigometer for the accurate mapping and diagnosis of the internal condition of woody support organs of trees. Arboricultural Journal: The International Journal of Urban Forestry, 23(3): 237–287.
Moreira da Silva AP, Schweizer D, Marques HR, Teixeira AMC, Nascente dos Santosb TVM, Sambuichi RHR, et al. 2016. Can current native tree seedling production and infrastructure meet an increasing forest restoration demand in Brazil? Restoration Ecology: 1–7. [online]: Available from onlinelibrary.wiley.com/doi/10.1111/rec.12470/suppinfo.
Mytton OT, Townsend N, Harry Rutter H, and Foster C. 2012. Green space and physical activity: an observational study using Health Survey for England data. Health Place, 18(5): 1034–1041.
Nasar JL, Evans-Cowley JS, and Mantero V. 2007. McMansions: the extent and regulation of super-sized houses. Journal of Urban Design, 12(3): 339–358.
Natural Resources Canada. 2021. Plant hardiness of Canada [online]: Available from planthardiness.gc.ca.
Norström AV, Cvitanovic C, Löf MF, West S, Wyborn C, Balvanera P, et al. 2020. Principles for knowledge co-production in sustainability research. Nature Sustainability, 3(3): 182–190.
Nowak DJ. 2012. Contrasting natural regeneration and tree planting in fourteen North American cities. Urban Forestry & Urban Greening, 11(4): 374–382.
Nowak DJ, Hoehn RE, Bodine AR, Greenfield EJ, and O’Neil-Dunne J. 2016. Urban forest structure, ecosystem services and change in Syracuse, NY. Urban Ecosystems, 19(4): 1455–1477.
Nutley SM, Walter I, and Davies HT. 2007. Using evidence: how research can inform public services. The Policy Press, Bristol, UK. 376 p.
Ordóñez C, Threlfall CG, Livesley SJ, Kendal D, Fuller RA, Davern M, et al. 2020. Decision- making of municipal urban forest managers through the lens of governance. Environmental Science and Policy, 104: 136–147.
Österle H, and Otto B. 2010. Consortium research: a method for researcher-practitioner collaboration in design-oriented IS research. Business and Information Systems Engineering, 2(5): 283–293. [online]: Available from aisel.aisnet.org/bise/vol2/iss5/3.
Ostrom E. 1991. Governing the commons: the evolution of institutions for collective actions. Cambridge University Press, Cambridge. 289 p.
Park People. 2020. The Canadian city parks survey 2020 [online]: Available from dev.ccpr.parkpeople.ca/uploads/ccpr_print2020_EN_G2_1a87af8874.pdf.
Pearce LM, Kirkpatrick JB, and Davison A. 2013. Using size class distributions of species to deduce the dynamics of the private urban forest. Arboriculture & Urban Forestry, 39(2): 74–84.
Phelan K, Hurley J, and Bush J. 2019. Land-use planning’s role in urban forest strategies: Recent local government approaches in Australia. Urban Policy and Research, 37(2): 215–226.
Purdy J, Kish-Gephart J, Labianca G, and Ansari S. 2018. Connections and collaboration— celebrating the contributions of Barbara Gray. Negotiation and Conflict Management Research, 11(1): 88–107.
Puskás I, and Farsang A. 2009. Diagnostic indicators for characterizing urban soils of Szeged, Hungary. Geoderma, 148(3-4): 267–281.
Rahman MA, Armson D, and Ennos AR. 2015. A comparison of the growth and cooling effectiveness of five commonly planted urban tree species. Urban Ecosystems, 18(2): 371–389.
Raupp MJ, Cumming AB, and Raupp EC. 2006. Street tree diversity in eastern North America and its potential for tree loss to exotic borers. Arboriculture & Urban Forestry, 32: 297–304.
Ricard RM. 2005. Shade trees and tree wardens: revising urban forest history. Journal of Forestry, 103(5): 230–233.
Richardson EA, Pearce J, Mitchell R, and Kingham S. 2013. Role of physical activity in the relationship between urban green space and health. Public Health, 127(4): 318–324.
Roman LA, Conway TM, Eisenman TS, Koeser AK, Barona CO, Locke DH, et al. 2021. Beyond ‘trees are good’: disservices, management costs, and tradeoffs in urban forestry. Ambio, 50: 615–630.
Roy S, Byrne J, and Pickering C. 2012. A systematic quantitative review of urban tree benefits, costs, and assessment methods across cities in different climatic zones. Urban Forestry & Urban Greening, 11(4): 351–363.
Ryder CM, and Moore GM. 2013. The arboricultural and economic benefits of formative pruning street trees. Arboriculture & Urban Forestry 39(1): 17–24.
Safford H, Larry E, McPherson EG, Nowak DJ, and Westphal LM. 2013. Urban forests and climate change. U.S. Department of Agriculture, Forest Service, Climate Change Resource Center. [online]: Available from fs.usda.gov/ccrc/topics/urban-forests.
Santamour FS. 1990. Trees for urban plantings: diversity, uniformity, and common sense. Proceedings from the 7th Conference Metropolitan Tree Improvement Alliance (METRIA), Morton Arboretum, Lisle, IL. pp. 57–65.
Scharenbroch BC, Carter D, Mailecki M, Scheberl L, Catania M, Roman LA, et al. 2017. A rapid urban site index for assessing the quality of street tree planting sites. Urban Forestry & Urban Greening, 27: 279–286.
Scharenbroch BC, and Catania M. 2012. Soil quality attributes as indicators of urban tree performance. Arboriculture & Urban Forestry, 38(5): 214–228.
Selin S, and Chavez D. 1995. Developing a collaborative model for environmental planning and management. Environmental Management, 19(2): 189–195.
Sieghardt M, Mursch-Radlgruber E, Paoletti Couenberg E, Dimitrakopoulus A, Rego F, Hatzistatthis A, et al. 2005. The abiotic urban environment: impact of urban growing conditions on urban vegetation. In Urban Forests and Trees. Edited by CC Konijneendijk, K Nilsson, TB Randrup, and J Schipperijn. Springer, Berlin. pp. 281–323.
Simatupang TM, Wright AC, and Sridharan R. 2002. The knowledge of coordination for supply chain integration. Business Process Management, 8(3): 289–308.
Simpson PB, and Wilkinson GF. 2020. What makes a drug discovery consortium successful? Nature Reviews Drug Discovery, 19: 737–738.
Sinnett D. 2020. Mitigating air pollution and the urban heat island effect: the roles of urban trees. In The Routledge Handbook of Urban Ecology. 2nd ed. Edited by I Douglas, D Goode, M Houck, and D Maddox. Routledge. p. 1176.
Sjöman H, Gunnarsson A, Pauleit S, and Bothmer R. 2012. Selection approach of urban trees for inner-city environments: learning from nature. Arboriculture & Urban Forestry, 38(5): 194–204.
Sjöman H, Hirons AD and Bassuk NL. 2018. Improving confidence in tree species selection for challenging urban sites: a role for leaf turgor loss. Urban Ecosystems, 21: 1171–1188.
Sjöman H, Morgenroth J, Sjöman JD, Sæbø A, and Kowarik I. 2016. Diversification of the urban forest- can we afford to exclude exotic tree species? Urban Forestr & Urban Greening, 18: 237–241.
Sjöman H, Nielsen AB, Pauleit S, and Olsson M. 2010. Habitat studies identifying potential trees for urban paved environments: a case study from Qinling Mt., China. Arboriculture & Urban Forestry, 36(6): 261–271.
Statistics Canada. 2017. Population centre (POPCTR) [online]: Available from www12.statcan.gc.ca/census-recensement/2016/ref/dict/geo049a-eng.cfm.
Steenberg JWN, Millward AA, Nowak DJ, and Robinson PJ. 2016a. A conceptual framework of urban forest ecosystem vulnerability. Environmental Reviews, 25(1): 115–126.
Steenberg JWN, Millward AA, Nowak DJ, Robinson PJ, and Ellis A. 2016b. Forecasting urban forest ecosystem structure, function, and vulnerability. Environmental Management, 59: 373–392.
Sydnor TD, Subburayalu S, and Bumgardner M. 2010. Contrasting Ohio nursery stock availability with community planting needs. Arboriculture & Urban Forestry, 36(1): 46–54.
Tengö M, Brondizio ES, Elmqvist T, Malmer P, and Spierenburg M. 2014. Connecting diverse knowledge systems for enhanced ecosystem governance: the multiple evidence base approach. Ambio, 43(5): 579–591.
Threlfall CG, Walker K, Williams NSG, Hahs AK, Mata L, Stork, N, et al. 2015. The conservation value of urban green space habitats for Australian native bee communities. Biological Conservation, 187: 240–248.
Tree Canada. 2015. Ontario sets standards for the urban forest [online]: Available from treecanada.ca/blog/ontario-sets-standards-for-the-urban-forest/.
Tree Canada. 2019a. Canadian urban forest strategy 2019-2024 [online]: Available from treecanada.ca/wp-content/uploads/2019/04/TC-CUFS-2019-2024-Eng.pdf.
Tree Canada. 2019b. Tree Canada’s growing support of urban forestry in indigenous communities across Canada [online]: Available from treecanada.ca/blog/tree-canadas-growing-support-of-urban-forestry-in-indigenous-communities-across-canada/.
Trudeau J. 2019. Minister of natural resources mandate letter [online]: Available from pm.gc.ca/en/mandate-letters/2019/12/13/minister-natural-resources-mandate-letter.
Tubby KV, and Webber JF. 2010. Pests and diseases threatening urban trees under a changing climate. Forestry, 83(4): 451–459.
Ugolini F, Massetti L, Sanesi G, and Pearlmutter D. 2015. Knowledge transfer between stakeholders in the field of urban forestry and green infrastructure: results of a European survey. Land Use Policy, 49: 365–381.
Ulmer JM, Wolf KL, Backman DR, Tretheway RL, Blain CJA, O’Neil-Dunne JPM, et al. 2016. Multiple health benefits of urban tree canopy: the mounting evidence for a green prescription. Health & Place, 42: 54–62.
United Nations (UN). 2014. New York declaration on forests: declaration and action plan. climate summit 2014. [online]: Available from undp.org/content/dam/undp/library/Environment%20and%20Energy/Forests/New%20York%20Declaration%20on%20Forests_DAA.pdf.
Vineland Research & Innovation Centre. 2021a. Greening the landscape research consortium[online]: Available from vinelandresearch.com/research-programs/greening-the-landscape-research-consortium/.
Vineland Research & Innovation Centre. 2021b. Greening the landscape research consortium strategy map [online]: Available from vinelandresearch.com/wp-content/uploads/2021/09/Greening-the-Landscape-Research-Consortium-Strategy- Map.pdf.
Vogt J. 2017. Urban forests in the Anthropocene: the responsibility of urban forestry in meeting global sustainability challenges. In Urban Forest Sustainability. Edited by Z Ning, DK Nowak, and G Watson. International Society of Arboriculture, Champaign, Illinois. 365 p.
Vogt J. 2018. “Ships that pass in the night”: Does scholarship on the social benefits of urban greening have a disciplinary crosstalk problem? Urban Forestry & Urban Greening, 32: 195–199.
Vogt J, Hauer RJ, and Fischer BC. 2015. The costs of maintaining and not maintaining the urban forest: a review of the urban forestry and arboriculture literature. International Society of Arboriculture, 41(6): 293–323.
Vogt J, Fischer BC, and Hauer RJ. 2016. Urban forestry and arboriculture as interdisciplinary environmental science: importance and incorporation of other disciplines. Journal of Environmental Studies and Sciences, 6: 371–386.
Vogt J, Roman LA, Brandt L, and Ries P. (In press). Urban forestry for the Anthropocene: transdisciplinary efforts for meeting new challenges. [online]: Available from lufa-depaul.org/interdisciplinary.html#:∼:text=Urban%20forestry%20is%20the%20transdisciplinary,there%20have%20been%20permanent%20settlements.
Walker B, and Salt D. 2006. Living in a complex world: an introduction to resilience thinking. In Resilience thinking: sustaining ecosystems and people in a changing world. Island Press, Washington. pp. 1–14.
Wagner JA, Prince M, Wright EC, Ennis MM, Kochan J, Nunez DJR, et al. 2010. The Biomarkers consortium: practice and pitfalls of open-source precompetitive collaboration. Clinical Pharmacology & Therapeutics, 87(5): 539–542.
Watkins SL, and Gerrish E. 2018. The relationship between urban forests and race: a meta-analysis, Journal of Environmental Management, 209(1): 152–168.
Watson G. 1985. Tree size affects root regeneration and top growth after transplanting. Journal of Arboriculture, 11(2): 37–40.
Watson GW, Hewitt AM, Custic M, and Lo M. 2014. The management of tree root systems in urban and suburban settings: a review of soil influence on root growth. Arboriculture & Urban Forestry, 40(4): 193–217.
Whittet R, Cottrell J, Cavers S, Pecurul M, and Ennos R. 2016. Supplying trees in an era of environmental uncertainty: identifying challenges faced by the forest nursery sector in Great Britain. Land Use Policy, 58(15): 415–426.
Wood SLR, and Dupras J. 2021. Increasing functional diversity of the urban canopy for climate resilience: potential tradeoffs with ecosystem services? Urban Forestry & Urban Greening, 58: 126972.
Yu Z, Yan H, and Cheng TE. 2001. Benefits of information sharing with supply chain partnerships. Industrial Management & Data Systems, 101(3): 114–121.
Zupancic T, Westmacott C, and Bulthuis M. 2015. The impact of green space on heat and air pollution in urban communities: a meta-narrative systematic review. [online]: Available from davidsuzuki.org/wp-content/uploads/2017/09/impact-green-space-heat-air-pollution-urban-communities.pdf.
Information & Authors
Information
Published In
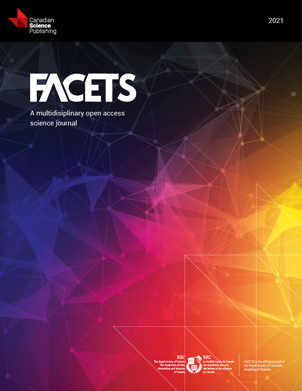
FACETS
Volume 6 • Number 1 • January 2021
Pages: 2084 - 2109
Editor: Peter G. Kevan
History
Received: 17 June 2021
Accepted: 16 September 2021
Version of record online: 16 December 2021
Copyright
© 2021 McGrath et al. This work is licensed under a Creative Commons Attribution 4.0 International License (CC BY 4.0), which permits unrestricted use, distribution, and reproduction in any medium, provided the original author(s) and source are credited.
Data Availability Statement
All relevant data are within the paper.
Key Words
Sections
Subjects
Authors
Author Contributions
All conceived and designed the study.
DM and AB performed the experiments/collected the data.
DM and AB analyzed and interpreted the data.
DM and RP contributed resources.
DM and RP drafted or revised the manuscript.
Competing Interests
The authors have declared that no competing interests exist.
Metrics & Citations
Metrics
Other Metrics
Citations
Cite As
Darby McGrath, Ryan Plummer, and Amy Bowen. 2021. Cultivating our urban forest future: a value-chain perspective. FACETS.
6: 2084-2109.
https://doi.org/10.1139/facets-2021-0076
Export Citations
If you have the appropriate software installed, you can download article citation data to the citation manager of your choice. Simply select your manager software from the list below and click Download.
Cited by
1. Strategi Pengelolaan Ruang Terbuka Hijau (RTH) Kabupaten Kuningan Berdasarkan Tingkat Kesehatan Pohon
2. Why Can’t We All Just Get Along? Conflict and Collaboration in Urban Forest Management
3. The Big Picture and Future Directions for Urban Bat Conservation and Research