What is habitat sensitivity? A quantitative definition relating resistance, resilience, and recoverability to environmental impacts
Abstract
Habitat sensitivity is a consideration for decision-making under environmental laws in many jurisdictions. However, habitat sensitivity has been variously defined and there is no consistent approach to its quantification, which limits our understanding of how habitat sensitivity varies among systems and in response to different pressures. We review various definitions offered in the scientific literature and policy documents before suggesting a universal framework for habitat sensitivity as (i) a habitat trait that defines the ecological impacts from a given pressure, (ii) which is composed of three components (habitat resistance, resilience, and recoverability), and (iii) which is quantified by measuring the change and recovery in the state of key habitat attributes in response to pressures. In addition, we provide guidance toward a consistent approach to assessing habitat sensitivity, which includes the use of pressure benchmarks and standardized metrics of change in key habitat attributes to create a common scale for comparison among habitat attributes and pressures. Our framework and recommendations should help to standardize the way in which habitat sensitivity is defined and assessed, and could be integrated into decision-making processes to improve ecosystem management in different jurisdictions.
1. Introduction
Habitat sensitivity is a central consideration for decision-making under various environmental statutes and policies in Canada and abroad. For example, under the Fish and Fish Habitat Protection Policy Statement (2019), which outlines Fisheries and Oceans Canada’s approach to implementing the Fish and Fish Habitat Protection provisions of the Fisheries Act (2019), the department intends to “adopt a risk-based approach to the application of the fish and fish habitat protection provisions subject to the sensitivity of the fish and fish habitat in question” and establish ecologically significant areas to “manage fish and fish habitat that is highly sensitive, highly productive, rare, or unique” (DFO 2019). Similarly, habitat sensitivity is a consideration for selecting sites for renewable energy projects under the Wildlife Directive for Alberta Wind Energy Projects (Alberta Environment and Parks 2017), and is a condition under which Indigenous governments can restrict public access to land in some modern treaties in British Columbia (British Columbia 1999, 2013). Internationally, habitat sensitivity is considered when categorizing and protecting habitat under New Zealand’s Exclusive Economic Zone and Continental Shelf Regulations (2013) and California’s Coastal Act (2022), among other statutes.
Despite widespread reference to “habitat sensitivity” in policy and legislation, there is no standardized scientific method for its assessment and quantification. This absence is in part due to the fact that there is also no commonly accepted definition of habitat sensitivity, nor it is clear how habitat sensitivity relates to other concepts in ecosystem management such as impact, vulnerability, and risk. The lack of scientific consistency in the definition and quantification of habitat sensitivity could impede effective ecosystem management by complicating the development and application of new environmental policy and regulations, thwarting meaningful comparative analyses, and placing additional burden on project proponents and environmental practitioners to (re)define habitat sensitivity on a case-by-case basis (Cullen 1990; Stirzaker et al. 2010). It also undermines consistency in governance when key ecological states identified in policy or legislation have no established scientific definition.
As a step towards addressing this issue, we pursue two objectives. First, we briefly review the definitions of “habitat sensitivity” offered in the scientific literature and environmental policy, and identify common features of these definitions. Second, we suggest a unified definition of habitat sensitivity, and propose a framework for assessing it, including recommendations that support a consistent approach to assessing and comparing habitat sensitivity across habitats and jurisdictions. We apply this framework to a case study drawn from previously published data and include general discussion related to the challenges of assessing habitat sensitivity across diverse ecosystems and pressures.
2. Past definitions of habitat sensitivity
That natural systems vary in their response to changing environmental conditions is a fundamental concept in natural science. Based on a history of studies on ecological niches (Strom 1946), food webs (Huxel and McCann 1998), and comparative physiology (Clark and Worland 2008), there are solid theoretical foundations to predict that different species and communities will show different responses to natural and anthropogenic pressures. Similarly, abiotic habitat attributes such as sediment regimes (Abbott et al. 2018; Rathburn et al. 2018), geomorphology (Tooth 2018), and water temperature (Luce et al. 2014) are dynamic and respond differently to changing environmental conditions associated with pressures (Kail et al. 2015).
Starting in the 1990s, references to “habitat sensitivity” in the scientific literature started to bring together ideas related to how biotic and abiotic attributes of ecosystems respond to natural and anthropogenic pressures (e.g., Webb et al. 1994; Michel et al. 1995; Hiscock et al. 1999). In some jurisdictions, substantial progress has been made towards developing a systematic approach to quantifying the sensitivity of certain habitat types (Tyler-Walters et al. 2018; OSPAR Commission 2019), but whether these approaches are applicable to other ecosystems and jurisdictions is unclear. Establishing consistent assessments of habitat sensitivity requires agreement on the definition of habitat sensitivity. However, various authors have characterized habitat sensitivity using widely differing definitions (Table 1).
Table 1.
Conceptual definition of habitat sensitivity | Key references |
---|---|
The amount of disturbance a habitat could withstand before its essential characteristics are altered | Webb et al. 1994; Hiscock et al. 1999; Bax and Williams 2001; Eno et al. 2013; La Rivière et al. 2016; Tyler-Walters et al. 2018 |
The magnitude of change in a habitat’s characteristics in response to a pressure of a given intensity; the slope of a stressor–response relationship | Laffoley et al. 2000; Acreman et al. 2008 |
The rate at, or degree to which, a habitat would recover from a pressure | Laffoley et al. 2000; Bax and Williams 2001; Hiddink et al. 2007;Eno et al. 2013; La Rivière et al. 2016; Tyler-Walters et al. 2018; Hodgson et al. 2022 |
The number or abundance of species residing in a habitat | Critical Areas Ordinance of Skamania County, Washington, 2019 |
The rarity or uniqueness of the habitat or its resident species | NIWA, 2013; California Division of Environmental Analysis, 2022 |
The value or importance of the habitat | Goldsmit et al. 2019; Impact Assessment Agency of Canada, 2020; California Division of Environmental Analysis, 2022 |
These initial definitions can be simplified into concepts of: (1) the disturbance and recovery of habitat from a pressure; (2) the ability of that habitat to support a unique assemblage or number of species; or (3) some perceived value of the habitat related to rarity, uniqueness, or importance. To further complicate the issue, habitat sensitivity has frequently been described as a multi-component concept that included two or more of the above elements. Additionally, habitat sensitivity is sometimes differentiated from habitat vulnerability, with the latter relating to the likelihood that a pressure will occur and the former to the habitat response to the pressure (Zacharias and Gregr 2005). Yet, other authors have included the likelihood of pressure occurrence as an integral component of sensitivity, or have considered sensitivity as a component of vulnerability (Hiscock et al.1999; Berry et al. 2003; Tyler-Walters et al. 2018).
While some of the above definitions could allow for habitat sensitivity to be objectively quantified from empirical data, many are not sufficiently specific to promote consistent approaches across contexts. Additionally, some of the previous definitions contain subjective components, such as “habitat value” or “essential characteristics”, which cannot be objectively measured. As a result, much of the history of evaluating habitat sensitivity has been based on qualitative assessments by experts, often using “risk-matrix” like approaches to derive single estimates of sensitivity from multiple criteria (see e.g., La Rivière et al. 2016). However, risk matrices can have questionable validity and many limitations, including a poor ability to correctly discriminate among different quantitative values (Cox 2008; Duijm 2015); these issues are likely to also apply to qualitative assessments of habitat sensitivity.
Perhaps the most common definition of habitat sensitivity is that initially offered by Laffoley et al. (2000), who stated that “A sensitive habitat or species is one that is easily affected by human activity, and is expected to only recover over a long period of time.” This definition includes two components describing (i) the resistance of habitats to change, and (ii) the resilience (i.e., recovery rate) of habitats after pressures cease. With slight modifications, this definition of habitat sensitivity has been adopted by many others (Eno et al. 2013; La Rivière et al. 2016; Tyler-Walters et al. 2018; Hodgson et al. 2022) and forms the basis for some of the more established habitat sensitivity assessment frameworks, including through the UK’s Marine Life Information Network (Tyler-Walters et al. 2018), and OSPAR’s multilateral program to identify sensitive species and habitats in the North-East Atlantic (OSPAR Commission 2019).
Implicit in Laffoley et al.’s definition of habitat sensitivity is the idea that there is a common understanding of the term “habitat”. However, many authors have pointed out that the definition of habitat itself is vague (Bax and Williams 2001; Mitchell 2005; Kearney 2006), and some have gone so far as to suggest that our present concept of habitat is of “very limited use in basic or applied ecology” (Mitchell 2005). When discussing habitat sensitivity, most authors adopt a broad definition of habitat inclusive of the hydrographic, physical, and chemical characteristics of an area, as well as all the species occurring in an area (Tyler-Walters et al. 2018). In this sense, habitat sensitivity is synonymous with ecosystem sensitivity, and diverges from the narrower definition of habitat as the “arena in which chemical and biological phenomena and community dynamics act and interact” (Minns and Wichert 2005). The challenge then is to define key attributes of habitats that are characteristic of the ecosystem and allow for quantification.
3. Towards a consistent and quantitative definition of habitat sensitivity
Building on the definitions of habitat sensitivity reviewed above, we aimed to develop a more precise definition of habitat sensitivity that would encourage a consistent approach to its quantification across ecosystems. Under our framework, habitat sensitivity is a trait of habitats (i.e., ecosystems) that modulates their response to external factors. When combined with the characteristics of a pressure (i.e., the pressure type, intensity, and duration), habitat sensitivity determines the impact to the habitat from the pressure, with higher sensitivity habitats being those that experience a greater impact from a given pressure. Importantly, this definition of habitat sensitivity is distinct from concepts of rareness/uniqueness of habitats, the value of habitats (e.g., for cultural or socioeconomic purposes), and the likelihood that a habitat is exposed to a pressure of a given type and magnitude (i.e., vulnerability). While these are also important considerations in ecosystem management, they should be considered separately from habitat sensitivity to promote clarity in terminology and methodology.
Quantification of habitat sensitivity requires consideration of the way in which pressures cause impacts to habitats. In a general sense, the impact of a pressure on a habitat will be a function of the magnitude and persistence of change in habitat state. The change in habitat state can occur (i) when the pressure is acting on the habitat, (ii) during the period after the pressure subsides and when habitat recovery is occurring, and (iii) continuing through a post-recovery period if the habitat attribute does not achieve full recovery to the pre-pressure state (i.e., if the habitat demonstrates hysteresis; Litzow and Hunsicker 2016). Based on this framework, we define habitat sensitivity as being composed of three components, (i) habitat resistance, which describes the magnitude of change in habitat state to a given pressure, (ii) habitat resilience, which describes the rate at which habitat state recovers from change, and (iii) habitat recoverability, which describes the degree to which habitat state recovers from change.
Quantification of habitat sensitivity then requires a means to measure resistance, resilience, and recoverability in real-world ecosystems, which are composed of a variety of species (each with a number of different life stages), abiotic traits, and a number of environmental and ecological processes acting at different timelines. Under our suggested framework, habitat sensitivity can be quantified by evaluating the resistance, resilience, and recoverability of a set of key habitat attributes (Fig. 1), which are metrics that characterize the state of important structural, functional, or characteristic species (Tyler-Walters et al. 2018), geological, chemical, or physical features, or measures of biological function (e.g., productivity).
Fig. 1.

We propose defining habitat resistance as the ability of a habitat to maintain its state in the face of a pressure. It is measured as the ratio of the intensity of the pressure to the magnitude of change in a key habitat attribute:where R is the habitat resistance, is the standardized intensity of the pressure (see Box 1), and is the change in a key habitat attribute as a result of the pressure, standardized to the baseline (reference) value for the attribute. Habitat resistance therefore represents the intensity of pressure required to produce a given level of change in a habitat attribute, and is high when habitats are able to maintain key attributes in the face of pressures. Furthermore, this definition of habitat resistance is the inverse of the slope of pressure–response functions, a common paradigm for describing the effect of stressors on ecological variables (Rosenfeld et al. 2022).
(1)
Box 1. Standardizing pressure intensity
A desired feature of quantifying habitat sensitivity is the ability to compare the sensitivity of a key habitat attribute to different pressures. Such an approach requires a way to standardize the intensity of pressures, such that the response of a habitat attribute to different pressure types (with correspondingly different measures and units of intensity) can be compared. To standardize the scale on which pressure intensity is considered, the marSEA habitat sensitivity assessment uses a set of benchmark values for pressures that describe expected pressure intensities, given that the pressure occurs (Tyler-Walters et al. 2018). In its simplest form, this is equivalent to standardizing to the mean pressure intensity for a given pressure type—although for pressures with skewed intensity distributions, a median value may be more appropriate. A pressure with a standardized intensity value > 1 would therefore represent a greater than expected intensity (e.g., a larger than average flood, a higher pollutant concentration than averaged in other spills, etc.), while values < 1 would represent an occurrence of a pressure with smaller than expected intensity. Using this approach, the response of a habitat feature to different pressures can be directly compared by standardizing to the expected pressure intensity and using the unit of “benchmarks”. We denote a standardized pressure intensity as throughout this paper.
Habitat resilience is the rate at which a habitat recovers following the cessation of a pressure:where L is the resilience of the habitat attribute, is the standardized magnitude of recovery in a key habitat attribute after the pressure has subsided, and is the time period over which recovery occurs, standardized relative to the time horizon (th) over which impacts are considered. Note that th is not a function of habitats or pressures, but a practical or policy consideration required for quantification.
(2)
Habitat recoverability is the amount of habitat recovery following a pressure relative to the amount of change as a result of the pressure:where C is the recoverability of a key habitat attribute, and and are as defined above.
(3)
Using these definitions and the framework outlined inFig. 1, we propose that the impact of a given pressure on a key habitat attribute be defined aswhere is the duration of the pressure, standardized to the time horizon. Because both the time variables and habitat variables are standardized, impact ranges between 0 and 1, and reflects the proportion of the maximum possible impact for a given habitat attribute and time horizon.
(4)
Substituting in the relationships defined by eqs. 1–3, the impact to a habitat attribute can also be expressed in terms of the pressure intensity, pressure duration, and the habitat sensitivity (i.e., resistance, resilience, and recoverability):
(5)
For further information on the derivation of eqs. 4 and 5, see the Electronic Supplementary Material. Equation 5 relates the impact of pressures directly to our three components of habitat sensitivity (namely, habitat resistance, habitat resilience, and habitat recoverability), and demonstrates that for a given level of pressure intensity and pressure duration, impact is higher when habitat resistance and habitat resilience are low. Similarly, impact is high when habitat recoverability is low, because increases faster than decreases, with increasing C (since C is bound between 0 and 1). Because we define habitat sensitivity as a trait that determines the level of impact for a given pressure, habitat sensitivity can therefore be said to be high when habitat resistance, habitat resilience, and habitat recoverability are low, which is consistent with a common use of the terms.
The simple geometric framework for estimating the impact of a pressure can be extended to consider impacts that do not occur instantaneously or impacts with delayed effects that continue to increase beyond the release of the pressure (see the supplementary material). While adding these complexities can be valid extensions of this framework, they do not change the main conclusions drawn from the simplified version presented.
4. Practical considerations for assessing habitat sensitivity
Ecosystems are complex, containing many component species, physical and chemical properties, and biological processes. The framework outlined above describes a conceptual basis for understanding how changes in the state of each key habitat attribute can be described in terms of resistance, resilience, and recoverability. However it is infeasible to consider the sensitivity of every attribute in a focal habitat, which is why we recommend that assessments of habitat sensitivity focus on key attributes that characterize important features of the habitat in question (e.g., keystone or regionally important species, variables of management focus). Selection of habitat attributes for assessment should ideally be driven by the purpose of the habitat assessment and be consistent among habitats to support comparisons. For example, under Canada’s Fisheries Act (2019) and the Fish and Fish Habitat Protection Program Policy Statement (2019), impacts on fish habitat are considered with respect to the habitat’s capacity to “support the life processes of fish”. Key habitat attributes appropriate for characterizing sensitivity under this Act and Policy Statement could therefore focus on the compositional and functional attributes of habitat that have a strong conceptual link to fish vital rates and life history, especially those linking to the vital rates and life history of species of regional importance.
In addition, assessing multiple habitat attributes would provide a broader understanding of the overall response of habitats, and would better align with an ecosystem approach to management, relative to assessing single habitat attributes. Depending on the purpose of the habitat assessment, the sensitivity of multiple habitat attributes may need to be combined into a single value to facilitate comparisons among habitats (e.g., during the consideration of candidate areas for protection). In such a case, a possible approach is to define the overall habitat sensitivity as equivalent to the sensitivity of the most sensitive key habitat attribute, with the argument that harm to a single key habitat attribute threatens ecological integrity. Alternatively, overall habitat sensitivity could be defined as the (geometric or arithmetic) mean value of the key habitat attributes under consideration. Additional methods of combining the sensitivity of multiple key habitat attributes into a single synthetic measure could pull from theory on multiple stressors (e.g., Liess et al. 2016; Schäfer and Piggott 2018) and ecological interactions, with the basis that impacts on multiple nodes within a network can cause emergent effects that are not easily predicted (Terborgh et al. 2001). However, the development of such approaches is beyond the scope of this paper.
We recognize that the framework outlined above does not address all practical and theoretical challenges associated with the assessment of habitat sensitivity. For example, when habitats respond nonlinearly to pressures (i.e., pressure–response curves that are nonlinear), the impact of a pressure of a given intensity will depend on the initial state of the habitat (Hunsicker et al. 2016), which further complicates comparisons among and within habitats. Similarly, exposure to other co- or previously occurring stressors can impact the magnitude of response to a focal pressure (Piggott et al. 2015; Jackson et al. 2016; Dey and Koops 2021), and it is not clear how these (potentially non additive) pressure interactions should be accounted for in habitat sensitivity assessment. Future research focused on how resistance, resilience, and recoverability can be quantified for systems experiencing multiple, interacting pressures would be valuable.
Additionally, our framework assumes (i) that the response of habitats to pressures is immediate, (ii) that recovery of habitat attributes occurs linearly, and (iii) that habitat attributes demonstrate some response to pressures (i.e., that the responses are nonzero). While these assumptions are not likely to hold across all systems, we include them in our framework to simplify the definition (and quantification) of impact, habitat resistance, resilience, and recoverability. Extending this framework to accommodate alternate shapes in the response and recovery of habitat attributes to pressures is theoretically possible, but is unlikely to change the general conclusions or applicability of the framework. As an example of such an approach, we demonstrate how relaxing our assumption of an immediate response in habitat attributes to pressures fails to produce meaningful differences in the quantification of impact in the Electronic Supplementary Materials.
5. Case study of the quantification of habitat sensitivity
To demonstrate the application of our framework, we review two case studies focusing on the impact of (i) a flood (Milner et al. 2018) and (ii) a pesticide spill (Reiber et al. 2021) on aquatic habitat in Wolf Point Creek, Alaska and the Holtemme River, Germany, respectively. The first step to assessing habitat sensitivity based on our framework is to define key habitat attributes that characterize the ecosystem. Based on their important role in aquatic food webs, and on data availability for these examples, the abundance and richness of macroinvertebrates will serve as key habitat attributes. In addition, we include the density of juvenile coho salmon as a key habitat attribute for the Alaskan system, as salmon represent a keystone aquatic species in many Alaskan rivers.
Next, we define benchmark pressure intensities for the pressures acting in each case study. In a mature habitat assessment program, such benchmark values would be set a priori (i.e., outside of any particular habitat sensitivity assessment) based on a solid evidentiary basis, and related to the probability distribution of the intensities of the focal pressure (i.e., considering their central tendencies). That is, a benchmark value should indicate the likely intensity of a pressure given that it occurs, such that a particular pressure event can be said to be of high or low intensity (e.g., above or below the average intensity value). Our goal here is not to define scientifically robust benchmark values, but instead to assign example benchmarks for illustrative purposes. In Milner et al. (2018), the flood under consideration is considered a “>1 in 100 year flood,” but no additional information on flood level or change in flow is provided. Assuming annual maximum flow levels follow a Weibull distribution (with shape and scale parameters of 1), then such a flood is at least 6.79 times larger than a flood with a 50% chance of annual occurrence (i.e., a 2 year flood). Therefore, we consider the standardized pressure intensity to be 6.79 benchmarks (i.e., = 6.79) for our reanalysis of data from Milner et al. (2018). In Reiber et al. (2021), the concentration of the focal pesticide cypermethrin increased from 0.2 to 30 ng/g in sediment downstream of the spill site. While we could not find data related to environmental concentrations of cypermethrin from other spills, the 10-day lethal concentration50 for Hyalella azteca exposed to cypermethrin in sediment was 3.6 ng/g (Maund et al. 2002). For illustrative purposes, we use this value (3.6 ng/g) as the pressure benchmark, which means that the focal spill of cypermethrin represents a standardized pressure intensity () of 8.28 benchmarks (i.e., 29.8 ng/g increase ÷ 3.6 ng/g benchmark = 8.28 benchmarks).
We consider the standardized change in each habitat attribute, using the pre-pressure value (Ho) as equivalent to the baseline value (Hb), and a time horizon (th) of 20 years after the pressure was initiated. The response of the habitat attributes varied among and within habitats (Fig. 2), with macroinvertebrate richness decreasing from 17 to 16 taxa in response to the flood in Wolf Point Creek ( = 0.06 units) and from 36 to 5 taxa in response to the pesticide spill in the Holtemme River ( = 0.86 units). Other key habitat attributes also showed relatively large responses (Fig. 2), with calculated resistance values for macroinvertebrate abundance being slightly higher in response to the pesticide spill in the Holtemme River compared to the flood in Wolf Point Creek, due to the higher pressure intensity at that site (Table 2).
Fig. 2.
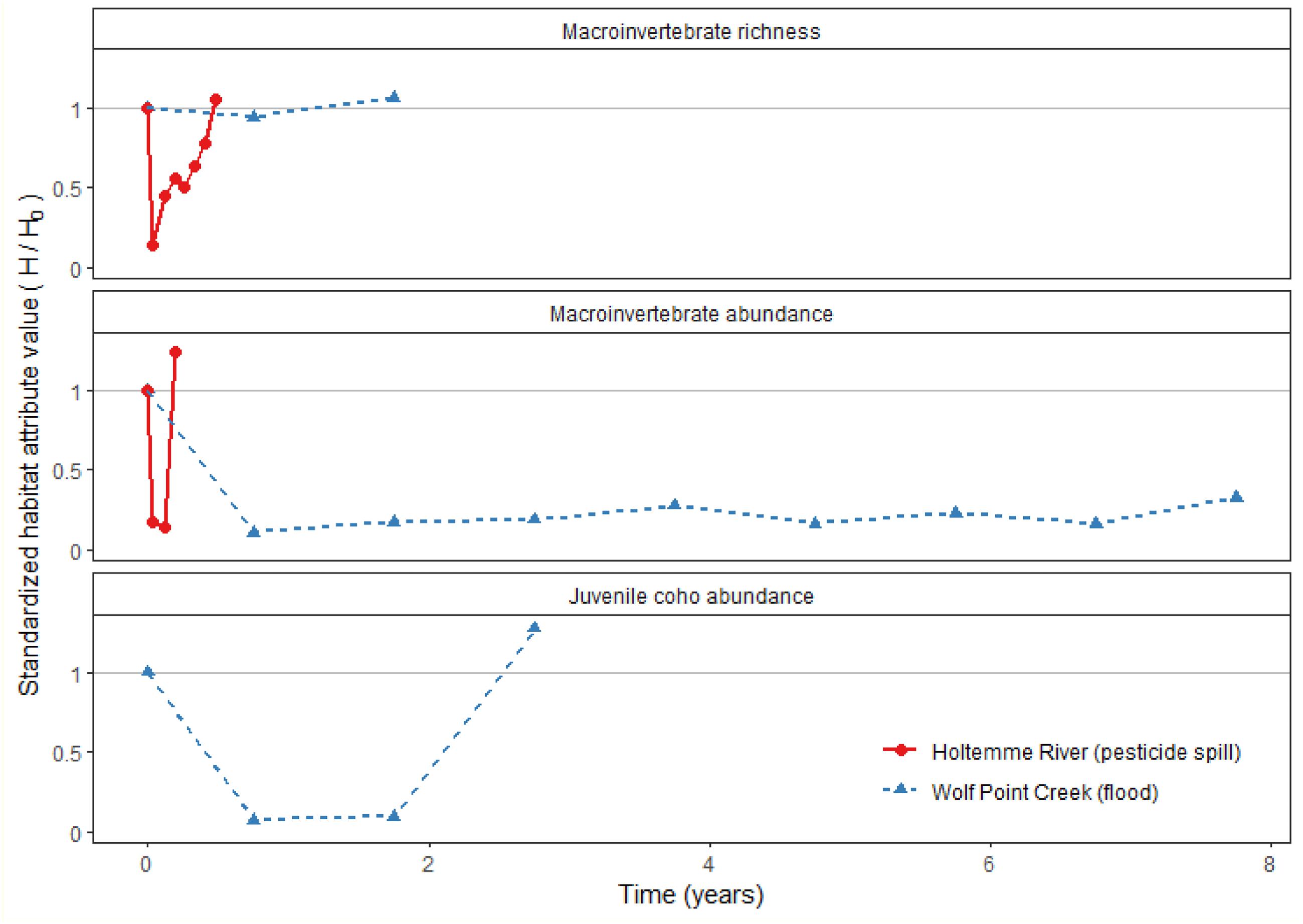
Table 2.
System and pressure | Key habitat attribute | Pressure intensity | Habitat resistance | Habitat resilience | Habitat recoverability | Impact |
---|---|---|---|---|---|---|
Wolf Point Creek flood | macroinvertebrate richness | 6.79 | 115.430 | 0.671 | 1 | 0.006 |
Wolf Point Creek flood | macroinvertebrate abundance | 6.79 | 7.605 | 0.561 | 0.244 | 0.717 |
Wolf Point Creek flood | juvenile coho abundance | 6.79 | 7.319 | 6.739 | 1 | 0.064 |
Holtemme River pesticide spill | macroinvertebrate richness | 8.28 | 9.615 | 35.921 | 1 | 0.010 |
Holtemme River pesticide spill | macroinvertebrate abundance | 8.28 | 9.964 | 87.918 | 1 | 0.004 |
Habitat resilience, estimated as a linear rate of recovery between the time that the pressure ceases (here, the same as the date the pressure initiated, because = 0) and the time that recovery ceased, was relatively high in response to the pesticide spill compared to the flood. Additionally, both the recoverability and the resilience of macroinvertebrate abundance in response to flooding at Wolf Point Creek was low, with abundance still 67.5% below the pre-pressure state nearly 8 years after the pressure occurred (Fig. 2). Other habitat attributes showed recoverability values of 1, with recovery achieved between 69 and 1005 days post-pressure, depending on the habitat attribute (Fig. 2).
6. Conclusion
The sensitivity of habitats to anthropogenic and natural pressures is a primary consideration for many ecosystem management decisions. We suggest that a universal definition of habitat sensitivity, as well as a standardized approach to its quantification, could improve the implementation of environmental policy and legislation, as well as improve the body of evidence concerning how ecosystems respond to pressures. The definition and framework presented herein precisely define the components of habitat sensitivity in a manner that allows for quantitative assessment, while also directly integrating the concept of habitat sensitivity with that of (environmental) impact. Such a link is helpful because impact (also called harm; DFO 2019) is an important consideration for ecosystem managers when considering whether to authorize development projects, as well as when considering whether habitat offsetting or banking activities provide sufficient compensation for anticipated alteration, destruction, or disruption of habitats (Clarke and Bradford 2014; Doka et al. 2022). Additionally, our framework for habitat sensitivity could be integrated with concepts of vulnerability and risk by applying probability distributions to define the likelihood of occurrence of pressures of different intensities and durations, and variance in the estimate values of resistance, resilience, and recoverability, and avoids a variety of issues associated with risk-matrix-like approaches that have poor discriminatory ability and high subjectivity.
A drawback in our framework is that the relationship between the components of habitat sensitivity and impact is complex as a result of (nonlinear) interactions between variables (Levin 1998). Therefore, comparison of sensitivity among different habitats is not straightforward unless there are systematic differences across all components of habitat sensitivity. That is, if one habitat has higher resistance, resilience, and recoverability values than another, it can be said to have lower sensitivity. However, the relative importance of resistance, resilience, and recoverability in determining impact depends on the dynamics of the pressure (i.e., its intensity and duration), as well as on the time horizon. As a result, it is not currently possible to define a single value for habitat sensitivity and comparison of sensitivity among different habitats will require engaging with its multiple components (i.e., resistance, resilience, and recoverability). Nonetheless, we believe this framework represents a significant step forward for generating repeatable and comparable estimates of habitat sensitivity, and that such an approach could provide great value in informed ecosystem management decisions.
Acknowledgements
This study was funded by DFO’s Freshwater Habitat Science Initiative and Competitive Science Research Fund. The manuscript was improved by discussions with Sean Naman and by reviews provided by Jordan Rosenfeld and one anonymous reviewer.
References
Abbott S., Julian J.P., Kamarinas I., Meitzen K.M., Fuller I.C., Mccoll S.T., Dymond J.R. 2018. State-shifting at the edge of resilience: river suspended sediment responses to land use change and extreme storms. Geomorphology, 305: 49–60.
Acreman M.C., Booker D.J., Goodwin T.H., Dunbar M.J., Maddock I., Hardy T., et al. 2008. Rapid assessment of physical habitat sensitivity to abstraction (RAPHSA). United Kingdom Environment Agency. Science Report - SC020081, https://assets.publishing.service.gov.uk/government/uploads/system/uploads/attachment_data/file/291707/scho0508boao-e-e.pdf.
Alberta Environment and Parks. 2017. Interpreting the areas of wildlife habitat sensitivity map. Available from https://open.alberta.ca/publications/areas-of-wildlife-habitat-sensitivity [accessed June 2021].
Bax N.J., Williams A. 2001. Seabed habitat on the south-eastern Australian continental shelf: context, vulnerability. Marine and Freshwater Research, 52: 491–512.
Berry P.M., Dawson T.P., Harrison P.A., Pearson R., Butt N. 2003. The sensitivity and vulnerability of terrestrial habitats and species in Britain and Ireland to climate change. Journal for Nature Conservation, 11(1): 15–23.
British Columbia. 1999. Nisg̱a'a Final Agreement Act.
British Columbia. 2013. Tla'amin Final Agreement Act.
California Division of Environmental Analysis. 2022. Environmentally Sensitive Habitat Areas. https://dot.ca.gov/programs/environmental-analysis/coastal-program/coastal-act-policy-resource-information/esha.
Clark M.S., Worland M.R. 2008. How insects survive the cold: molecular mechanisms—a review. Journal of Comparative Physiology B: Biochemical, Systemic, and Environmental Physiology, 178: 917–933.
Clarke K.D., Bradford M.J. 2014. A review of equivalency in offsetting policies. Canadian Science Advisory Secretariat Research Document, 109(December): i–v, 1–18.
Cox L. 2008. What’s wrong with risk matrices? Risk Analysis, 28(2): 497–512.
Cullen P. 1990. The turbulent boundary between water science and water management. Freshwater Biology, 24(1): 201–209.
Dey C.J., Koops M.A. 2021. The consequences of null model selection for predicting mortality from multiple stressors. Proceedings of the Royal Society B: Biological Sciences, 288(1948).
DFO. 2019. Fish and fish habitat protection policy statement. Available from https://www.dfo-mpo.gc.ca/pnw-ppe/policy-politique-eng.pdf [accessed June 2021].
Doka S.E., Minns C.K., Valere B.G., Cooke S.J., Portiss R.J., Sciscione T.F., Rose A. 2022. An ecological accounting system for integrated aquatic planning and habitat banking with case study on the Toronto waterfront, Ontario, Canada. Environmental Management, 952–971.
Duijm N.J. 2015. Recommendations on the use and design of risk matrices. Safety Science, 76: 21–31.
Eno N.C., Frid C.L.J., Hall K., Ramsay K., Sharp R.A.M., Brazier D.P., et al. 2013. Assessing the sensitivity of habitats to fishing: from seabed maps to sensitivity mapsa. Journal of Fish Biology, 83(4): 826–846.
Goldsmit J., McKindsey C., Archambault P., Howland K. L. 2019. Ecological risk assessment of predicted marine invasions in the Canadian Arctic. PloS one 14(2): e0211815.
Hiddink J. G., Jennings S., Kaiser M. J. 2007. Assessing and predicting the relative ecological impacts of disturbance on habitats with different sensitivities. Journal of Applied Ecology, 44(2): 405–413.
Hiscock K., Jackson A., Lear D. 1999. Assessing seabed species and ecosystems sensitivities. Report to the Department of the Environment Transport and the Regions from the Marine Life Information Network (MarLIN).
Hodgson E., Chu C., Mochnacz N.J., Shikon V., Millar E. 2022. Information needs for considering cumulative effects in fish and fish habitat decision-making. Canadian Science Advisory Secretariat (CSAS) Research Document.
Hunsicker M.E., Kappel C.V., Selkoe K.A., Halpern B.S., Scarborough C., Mease L., Amrhein A. 2016. Characterizing driver-response relationships in marine pelagic ecosystems for improved ocean management. Ecological Applications, 26(3): 651–663.
Huxel G.R., Mccann K. 1998. Food web stability: the influence of trophic flows across habitats. American Naturalist, 152(3): 460–469.
Impact Assessment Agency of Canada. 2020. Tailored Impact Statement Guidelines Template for Designated Projects Subject to the Impact Assessment Act and the Nuclear Safety and Control Act. https://www.canada.ca/en/impact-assessment-agency/services/policy-guidance/practitioners-guide-impact-assessment-act/tailored-impact-statement-guidelines-projects-impact-assessment-nuclear-safety-act.html.
Jackson M.C., Loewen C.J.G., Vinebrooke R.D., Chimimba C.T. 2016. Net effects of multiple stressors in freshwater ecosystems: a meta-analysis. Global Change Biology, 22(1): 180–189.
Kail J., Guse B., Radinger J., Schröder M., Kiesel J., Kleinhans M., et al. 2015. A modelling framework to assess the effect of pressures on river abiotic habitat conditions and biota. PLoS ONE, 10(6): 1–21.
Kearney M. 2006. Habitat, environment, and niche: what are we modelling. Oikos, 115(1): 186–191.
La Rivière M., Aish A., Gauthier O., Grall J., Guérin L., Janson A-L., et al. 2016. Natural Heritage Service assessing benthic habitats’ sensitivity to human pressures: a methodological framework—summary report, MNHN, p. Rapport SPN 2016–87.
Laffoley Dd'A, Connor D.W., Tasker M.L., Bines T. 2000. Nationally important seascapes, habitats and species. A recommended approach to their identification, conservation and protection, English Nature Research Report. Peterborough.
Levin S.A. 1998. Ecosystems and the biosphere as complex adaptive systems. Ecosystems, 1:431–436.
Liess M., Foit K., Knillmann S., Schäfer R.B., Liess H.D. 2016. Predicting the synergy of multiple stress effects. Scientific Reports, 6: 1–8.
Litzow M.A., Hunsicker M.E. 2016. Early warning signals, nonlinearity, and signs of hysteresis in real ecosystems. Ecosphere, 7(12).
Luce C., Staab B., Kramer M., Wenger S., Isaak D., McConnell C. 2014. Sensitivity of summer stream temperatures to climate variability in the Pacific Northwest. Water Resources Research, 50: 3428–3443.
Maund S.J., Hamer M.J., Lane M.C.G., Farrelly E., Rapley J.H., Goggin U.M., Gentle W.E. 2002. Partitioning, bioavailability, and toxicity of the pyrethroid insecticide cypermethrin in sediments. Environmental Toxicology and Chemistry, 21(1): 9–15.
Michel J., Halls J., Dahlin J. 1995. Environmental Sensitivity Index Guidelines, NOAA Technical Memorandum NOS ORCA 92. Seattle, Washington.
Milner A.M., Picken J.L., Klaar M.J., Robertson A.L., Clitherow L.R., Eagle L., Brown L.E. 2018. River ecosystem resilience to extreme flood events. Ecology and Evolution, 8(16): 8354–8363.
Minns C.K., Wichert G.A. 2005. A framework for defining fish habitat domains in Lake Ontario and its drainage. Journal of Great Lakes Research, 31: 6–27.
Mitchell S.C. 2005. How useful is the concept of habitat?—A critique. Oikos, 110(3): 634–638. Available from:.
NIWA. 2013. Sensitive marine benthic habitats defined. National Institute of Water and Atmospheric Research Ltd. Report WLG2013-18, https://www.qualityplanning.org.nz/sites/default/files/Sensitive%20marine%20benthic%20habitats%20defined.pdf.
OSPAR Commission. 2019. Criteria for the identification of species and habitats in need of protection and their method of application, OSPAR Agreement 2019-03.
Piggott J.J., Townsend C.R., Matthaei C.D. 2015. Reconceptualizing synergism and antagonism among multiple stressors. Ecology and Evolution, 5(7): 1538–1547.
Rathburn S.L., Shahverdian S.M., Ryan S.E. 2018. Post-disturbance sediment recovery: implications for watershed resilience. Geomorphology, 305: 61–75.
Reiber L., Knillmann S., Kaske O., Atencio L.C., Bittner L., Albrecht J.E., et al. 2021. Long-term effects of a catastrophic insecticide spill on stream invertebrates. Science of the Total Environment, 768: 144456.
Rosenfeld J., Gonzalez-Espinosa P., Jarvis L., Enders E., Bayly M., Paul A., et al. 2022. Stressor–response functions as a generalizable model for context dependence. Trends in Ecology and Evolution, 37(12): 1032–1035.
Schäfer R.B., Piggott J.J. 2018. Advancing understanding and prediction in multiple stressor research through a mechanistic basis for null models. Global Change Biology, 24(5): 1817–1826.
Stirzaker R., Biggs H., Roux D., Cilliers P. 2010. Requisite simplicities to help negotiate complex problems. Ambio, 39: 600–607.
Strom K.M. 1946. The ecological niche. Nature, 157: 375.
Terborgh J., Lopez L., Nuñez P., Rao M., Shahabuddin G., Orihuela G., et al. 2001. Ecological meltdown in predator-free forest fragments. Science, 294: 1923–1926.
Tooth S. 2018. The geomorphology of wetlands in drylands: resilience, nonresilience, or …?. Geomorphology, 305: 49–60.
Tyler-Walters H., Tillin H.M., d'Avack E.A.S., Perry F., Stamp T. 2018. Marine Evidence-based Sensitivity Assessment (MarESA) – A Guide. Marine Life Information Network. Marine Biological Association of the UK, Plymouth. https://plymsea.ac.uk/id/eprint/7942/1/MarESA-Sensitivity-Assessment-Guidance-Rpt-Mar2018v2.pdf.
Webb T., Daniel C.J., Korman J., Meisner J.D. 1994. Development of a fish habitat sensitivity indexing scheme for application in the Fraser River Basin. Canadian Manuscript Report of Fisheries and Aquatic Sciences, 2234.
Zacharias M.A., Gregr E.J. 2005. Sensitivity and vulnerability in marine environments: an approach to identifying vulnerable marine areas. Conservation Biology, 19(1): 86–97.
Supplementary material
Supplementary Material 1 (DOCX / 258 KB).
- Download
- 258.37 KB
Information & Authors
Information
Published In
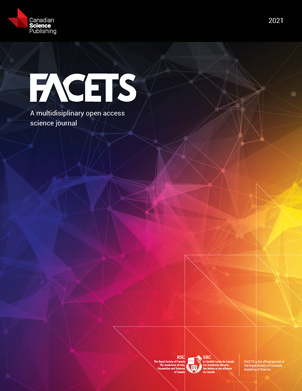
FACETS
Volume 9 • 2024
Pages: 1 - 9
Editor: Mark Mallory
History
Received: 16 September 2022
Accepted: 2 November 2023
Version of record online: 7 March 2024
Copyright
© 2024 The Author(s). This work is licensed under a Creative Commons Attribution 4.0 International License (CC BY 4.0), which permits unrestricted use, distribution, and reproduction in any medium, provided the original author(s) and source are credited.
Data Availability Statement
No original data were generated during this study. Data compiled for the case study (Section 5) are available from the original sources.
Key Words
Sections
Subjects
Plain Language Summary
A clear definition of habitat sensitivity to support environmental management
Authors
Author Contributions
Conceptualization: CJD, DARD, MAK, CC
Data curation: CJD, EIT
Formal analysis: CJD, EEH, MAK
Supervision: CC
Writing – original draft: CJD, EEH, DARD, MAK, CC
Writing – review & editing: CJD, EEH, DARD, MAK, CC
Competing Interests
The authors declare there are no competing interests.
Metrics & Citations
Metrics
Other Metrics
Citations
Cite As
Cody J. Dey, Erik I. Tuononen, Emma E. Hodgson, D. Andrew R. Drake, Marten A. Koops, and Cindy Chu. 2024. What is habitat sensitivity? A quantitative definition relating resistance, resilience, and recoverability to environmental impacts. FACETS.
9: 1-9.
https://doi.org/10.1139/facets-2022-0205
Export Citations
If you have the appropriate software installed, you can download article citation data to the citation manager of your choice. Simply select your manager software from the list below and click Download.
Cited by
1. Forestry impacts on stream flows and temperatures: A quantitative synthesis of paired catchment studies across the Pacific salmon range