Synthesizing and communicating climate change impacts to inform coastal adaptation planning
Abstract
Planning for adaptation to climate change requires regionally relevant information on rising air and ocean temperatures, sea levels, increasingly frequent and intense storms, and other climate-related impacts. However, in many regions there are limited focused syntheses of the climate impacts, risks, and potential adaptation strategies for coastal marine areas and sectors. We report on a regional assessment of climate change impacts and recommendations for adaptation strategies in the NE Pacific Coast (British Columbia, Canada), conducted in collaboration with a regional planning and plan implementation partnership (Marine Plan Partnership for the North Pacific Coast), aimed at bridging the gaps between climate science and regional adaptation planning. We incorporated both social and ecological aspects of climate change impacts and adaptations, and the feedback mechanisms which may result in both increased risks and opportunities for the following areas of interest: “Ecosystems”, “Fisheries and Aquaculture”, “Communities”, and “Marine Infrastructure”. As next steps within the region, we propose proactive planning measures including communication of the key impacts and projections and cross-sectoral assessments of climate vulnerability and risk to direct decision-making.
Introduction
Global climate change is widely recognized as the singular greatest threat to the world’s ecosystems, cultures, and economies (IPCC 2014a; Mora et al. 2018), including for marine systems (Hoegh-Guldberg and Bruno 2010; Gattuso et al. 2015; Weatherdon et al. 2016a). Anthropogenic climate change has led to increased global temperatures, changes in precipitation patterns, sea level rise, and increasing frequency and severity of extreme weather events (IPCC 2014a). While these changes are global, the ramifications occur at all scales, and decisions for adaptation must be made (Game et al. 2011). Coastal regions are unique and especially vulnerable to climate change (Frusher et al. 2016; Pinsky et al. 2019), and the impacts are diverse and often cumulative. Governments, regions, and communities need to understand what is happening, what knowledge gaps exist, and how they may be able to respond (Hobday et al. 2016).
Global climate change adds complexity to the already convoluted area of coastal planning. Changes in habitat suitability are predicted to cause an overall impact to global marine biodiversity by 60% by 2050 (Cheung et al. 2009). The direct impacts of these changes on ecosystems are either continuous such as shifts in species ranges, changes in phenology (timing of life cycle events), changes in species interactions, and ecological community composition (Parmesan and Yohe 2003; Parmesan 2006) or discrete such as massive coral bleaching events, and extreme weather events such as floods or storms (Hansen et al. 2016)). As climate change leads to shifting habitats and species ranges, many species are likely to be forced out of current reserves (Araújo et al. 2004; Pressey et al. 2007). The static nature of current conservation planning schemes tends to lack the flexibility necessary to fulfill conservation benefits under climate change scenarios (Pressey et al. 2007; Alagador et al. 2014). Even more difficult to predict are the indirect impacts of climate change caused by the human responses to climate change, such as migration, changing agricultural practices, shifting fisheries areas, or seawall construction (Maxwell et al. 2015; Jones et al. 2016).
Regional climate change planning and policy development can be informed by understanding the impacts, risks, vulnerabilities, and opportunities and by adaptive capacity assessments (Moser and Ekstrom 2010). While vulnerability assessments can provide information that is useful for policy-makers (e.g., risk assessments, infrastructure exposed to flooding, etc.), these summaries are only one component of effective adaptation planning. Vulnerability assessments typically focus on one or two climate impacts (e.g., sea level rise), do not identify adaptation strategies that are applicable to specific regions (Reiblich et al. 2017), and are not accessible and therefore actionable by local or sub-regional planners (Beier et al. 2017). While there has been ample research on coastal climate risks and adaptation recommendations specific to sea level rise (Lin et al. 2017), coastal retreat (Hino et al. 2017), and planning to protect coastal infrastructure (Brown et al. 2018), more integrated studies of climate impacts and adaptation planning in collaboration with practitioners are needed (Becker et al. 2018; Ng et al. 2018).
Integrated climate change assessments could inform in-process coastal planning and management, but there are limited examples of coastal planning processes worldwide that integrate climate change assessments (impacts). In the Arctic, a recent assessment of risk associated with climate change on a marine protected area (MPA) network found that climate risks will exceed those of industrial activities, and that Indigenous-led additional MPAs are recommended because of the implications of climate change on the presence and function of the species and ecosystems that MPAs are meant to protect (Bone 2018). In Portugal, expert judgement was applied to evaluate environmental properties, conservation features, and climate change pressures to the ecological components of the MPA network (Stratoudakis et al. 2019). Developing a functional MPA network that is informed by ecological information including current and observed climate impacts and considers social aspects is typically challenged by data limitations and capacity.
We applied a mixed-methods approach to integrate information on scientific climate change impacts with adaptation planning considerations to a NE Pacific coastal region (hereafter referred to as the North Pacific Coast) that is undergoing an integrative marine planning and plan implementation process. The purpose of the project was to synthesize the state of available scientific understanding of climate change impacts and projected further climate changes for the Northern Shelf Bioregion (NSB). In addition, we presented potential adaptation strategies to inform future work within the region or strategic next steps for adaptation that could be taken to address the needs of coastal communities. The literature in this field is wide ranging and growing rapidly, and much of the work is in progress and therefore not published or readily available. Thus, our research, guided by the advice offered by key informants, was based on both peer-reviewed literature and government agency reports (federal and provincial).
Methods
In this section, we describe the project process, the methodology for the review of climate impacts for the North Pacific Coast, and the analysis to evaluate the body of evidence for relevant climate variables and related impacts. We then explain how we evaluated the adaptation strategies in response to those impacts, our ongoing efforts to engage local and sub-regional planners in this topic, and the next steps. The methodology of the research presented here consisted of the following systematic steps: key informant interviews to guide the process, literature and data review, evaluation of evidence qualities, and recommendations for context-relevant adaptation strategies.
Process
Marine planning through the Marine Plan Partnership for the North Pacific Coast (MaPP) started in 2011 in British Columba (BC), Canada, and four sub-regional marine plans for the North Coast, the Central Coast, North Vancouver Island, and Haida Gwaii were completed in the spring of 2015 (mappocean.org). MaPP is a co-led process between 16 First Nations and the Government of the Province of British Columbia that developed and is implementing plans for marine uses on BC’s North Pacific Coast, now and into the future. A Regional Action Framework (RAF) followed and was completed in the spring of 2016. Together, the four sub-regions make up the NSB (Fig. 1). Through MaPP, provincial and Indigenous (First Nations) governments in BC are working towards sustainable development, improving economic opportunity, and supporting ecological integrity for the North Pacific Coast.
Fig. 1.



This project was co-developed with MaPP through the Climate Change Sub-Committee coordinated by the Regional Projects Coordinator, to shape our objectives, framing, and end products. We met with the Regional Projects Coordinator and Climate Change Sub-Committee co-chairs of MaPP on a biweekly basis over a 9-month period and presented draft and final reports to the Climate Change Sub-Committee, which is comprised of First Nations and provincial MaPP Partner technical planners and staff from each sub-region. These meetings helped to ground the project’s goals and focus our research on relevant topics to practitioners and communities in the region. The draft and final products were also shared with the same audience to elicit feedback and target the end product to the needs of the MaPP Partners. We also presented a summary webinar to an inclusive audience of marine planners and managers who work within the NSB to advance the goals of increasing communication and awareness of climate impacts. We emphasized communicating climate impacts and relevant adaptation actions because studies have shown that this is important for adaptation planning (Carpenter et al. 2001; Walker et al. 2004; Malakar 2013). Finally, the ongoing work in this project includes sharing the results through a graphically designed report and an interactive HTML web-based platform whereby First Nations, local and provincial marine planners, educators, and the general public can engage with the material and have further conversations (Nerlich et al. 2010) about the impacts and opportunities related to these climate change impacts in the NSB.
Interviews
To enquire about ongoing projects and to further inform our approach and data sources, we contacted and interviewed key practitioners and experts (hereafter, key informants) in the region involved in the climate change science and adaptation planning.
The criteria used to select the key informants included:
1.
a lead author on a previously published seminal paper or report on climate change impacts in the study area, and (or)
2.
a researcher recommended through snowball sampling; we asked each of our expert contacts whom else we should contact.
We conducted semi-structured interviews with key informants either in person or on the phone; a range of questions were asked regarding their knowledge on the ongoing projects in BC, data sources, and quality of reports and other publications (see Supplementary Material 1 for interview guide).
Literature and data review
We included peer-reviewed, grey literature, and spatial data to synthesize climate change projections, impacts, and adaptation strategies through a focused literature scan including materials provided by MaPP. As this was not intended to be a systematic literature review, and our inclusion criteria allowed for us to incorporate unpublished sources, care should be taken in interpreting the results. Sources included previous climate change vulnerability and risk assessment work from MaPP and associated contractors (including from the MaPP data portal; mappocean.org/resources/data/); publications from the Intergovernmental Panel on Climate Change (IPCC) reports; peer-reviewed academic studies on climate change impacts (published prior to 15 July 2017); assessment methods for climate change vulnerabilities and risks; external reports on predicted climate changes, impacts (e.g., BC Parks Shoreline Sensitivity Analysis 2014 (Biffard et al. 2014), Environment Canada downscaled global climate models (Canadian Centre for Climate Modelling and Analysis 2017), and Natural Resources Canada reports) (Fig. 2). Because of the rapidly evolving state of research on climate change projections, except for some fundamental background information, we only included publications from the year 2000 and newer.
Fig. 2.

We reviewed these available data sources and extracted relevant information for the region, where possible, to categorize climate projections and associated sectoral impacts and exposure to four key areas of interest of the coastal social–ecological system in the NSB: (i) ecosystems, (ii) fisheries and aquaculture, (iii) human communities, and (iv) marine infrastructure. We included both observed and projected information on a set of climate variables that were determined as most relevant to MaPP: air temperature, sea surface temperature, ocean acidification, sea level rise, ocean deoxygenation, sea surface salinity, and precipitation.
To present the uncertainty in climate change projections, we included the projections for climate change variables at the lowest and highest IPCC Representative Concentration Pathway (RCP) scenarios (IPCC 2014b), or the “best-case” and “worst-case” scenario, whenever possible. Long-term projections or trends within BC are expected to align with global trends in a general sense (i.e., warmer temperatures, more acidic ocean, more marine stratification, and rising sea levels), but climate changes may result in regional and sub-regional interactions with localized ocean and atmospheric conditions. The ecological complexity of the BC coast and NSB is high as is the uncertainty in regionally downscaled climate change projections. In some cases, the spatial data for climate projections within BC were only available for certain scenarios (e.g., sea level rise projections, available for RCP 4.5 and RCP 8.5 only). Many of the available regional and sub-regional climate projections are available through the Pacific Climate Impacts Consortium (pacificclimate.org/), who use an average set of climate models from the IPCC to conduct regional analyses for the BC region at a “business as usual” level of emissions (RCP 8.5) (Murdock and Spittlehouse 2011; Sobie and Murdock 2017). The current state of uncertainty in both projections of climate impacts and the choices that society makes in terms of emissions reductions means that our findings include relatively simplified, generalizable impact statements and adaptation options.
Assessing evidence qualities
To assess reporting of climate change projections and sectoral impacts, vulnerabilities, and risks, we used three systematic steps. First, we applied a reliability score based on (i) the robustness of the evidence, such as whether the evidence is the result of an experimental/quasi-experimental (robust), observational (medium), or anecdotal (limited) study (IPCC 2014b; Salafsky et al. 2019) and (ii) the agreement levels, such as high agreement to medium and low agreement levels, to qualitatively assess document quality and reliability of information. Using this method, our aim was not to assess the evidence quality of each climate impacts on different components of different sectoral impacts, rather to present an overall picture of the evidence quality of the climate impacts on different focal areas (e.g., fisheries and aquaculture, ecosystems, communities, and marine infrastructure) and the lack of it in some cases. This method was adopted from that of the IPCC Assessment Report 5’s evidence confidence scale, where findings were grounded in an evidence and agreement evaluation. We ranked each reference on a three-point evidence quality scale based on our literature review, expert interviews, and research team’s knowledge and expertise on the field. Next, we documented all the relevant information and references of each climate variable (existing and projected) and sector (Ecosystems, Communities, Fisheries and Aquaculture, and Marine Infrastructure) for the NSB. Lastly, we calculated overall evidence qualities by averaging evidence qualities scores for each climate variable and sector at both a regional and sub-regional scale based on the cited references and normalized the results to restructure the relational results without distorting differences in the ranges of values. The normalized values then were classified into “low”, “medium”, and “high” groups using tertile cutpoints. The evidence qualities shown in Figs. 3 and 4 demonstrate the results for the combined MaPP regional-scale assessment; sub-regional results are provided in Supplementary Material 2.
Fig. 3.





Fig. 4.





Adaptation strategies
Across Canada, awareness of climate change impacts and the necessity of adaptation planning is increasing (Lemmen et al. 2016). In BC, the provincial Adaptation Strategy (Government 2010) aims to prepare the province for the impacts of climate change to the environment and social systems. Under the BC Climate Action Plan, there are a range of actions that are intended to help increase resilience to climate change and reduce vulnerabilities. This adaptation plan is based on three key components: building a strong knowledge base with tools for decision-makers to prepare for climate change, incorporating climate adaptation within policy decisions, and improving assessment, and implementation of appropriate adaptation actions in especially vulnerable sectors (Government 2010). While these reports and adaptation plans are encouraging and signify a shift in management and governance investment in climate adaptation, there has been until recently far less investment in adaptation research and planning for coastal management and fisheries sectors when compared with terrestrial areas and sectors such as forestry and agriculture (Warren and Lemmen 2014a).
We defined adaptation options broadly to include current and potential proactive strategies that could help to address the impacts to coastal ecosystems, fisheries, coastal communities, and marine infrastructure in the social–ecological context of the North Pacific Coast and that could be feasible and effective in increasing the potential for those focal areas to adapt to diverse climate impacts.
Results
Trends and projections of climate variables
Along the coast of BC, ocean water temperatures are warming, and nearshore waters are becoming less salty, less oxygenated, and more acidic (a chemical process resulting from them becoming enriched in carbon dioxide; Chandler et al. 2017; Fig. 3). These changes are contributing to biological changes such as shifting species distributions (Bond et al. 2015; Cheung et al. 2015). To understand and anticipate climate change impacts, a range of modeling approaches has been used to characterize and predict ecological and biological responses along the BC coast and the surrounding marine area. Research focused on and within the NSB has included descriptions of the ecological communities and context, trophodynamic modeling, and the effects of climate change on fisheries and ecosystems (Ainsworth et al. 2002, 2011, 2008; Guénette and Alder 2007; Okey et al., 2014, 2015; Clarke Murray et al. 2015). There is a lack of reliable downscaled projections or regional climate modeled data for most climate impacts in the marine environment of BC and within the NSB. Much of the climate impacts work within BC is focused on terrestrial impacts (e.g., Hamann and Wang 2006) and as such is of limited applicability to the marine and coastal focus of the NSB (T. Murdoch, PCIC, personal communication, July 2017).
Air temperature
Air temperatures have increased throughout BC by ∼1.3 °C over the past century (from 1900 to 2013; 0.12–0.13 °C per decade (Rodenhuis et al. 2006; PCIC 2013a; BCME 2016; Vadeboncoeur 2016), slightly more than the global average during the same period (IPCC 2014b) but less than the rest of Canada (Warren and Lemmen 2014b). Most of this warming trend has been observed during the winter months (average increase of 2.2 °C per century), and in the north (3–3.8 °C per century) and south-central (2.6–2.9 °C per century) areas of BC (Rodenhuis et al. 2006; BCME 2016). Average daily minimum temperatures have increased the most (increased 2.3 °C this century) across the province, while average daily maximum temperatures have increased by 0.7 °C per century (BCME 2016; Chandler et al. 2017). Both daily average and daily minimum air temperatures in BC reached record high levels in 2016; monthly temperatures in the winter of 2016 were more than 5 °C higher than the baseline period of 1971–2000 (Chandler et al. 2017). These higher temperatures negatively as well as positively affect ecosystems and human activities. Increasing air temperatures have been associated with decreased heating requirements over the past century in BC, especially in northern BC (BCME 2016), while the energy demand for cooling infrastructure has increased, especially in the southern interior of BC (BCME 2016). BC is expected to experience more warming than the global average (Pike et al. 2008; Spittlehouse 2008); air temperatures are projected to increase by 1.8 °C by the 2050s and 2.7 °C by the 2080s, and could reach of 3–5 °C by the end of the 21st century (Pike et al. 2008; Spittlehouse 2008; Mote and Salathé 2010; PCIC 2013b; Fig. 3).
Sea surface temperature
Average annual sea surface temperatures have warmed between 0.6 and 1.4 °C per century across the coast of BC (BCME 2016), as monitored at lighthouse stations along the BC coastline. This rate is similar to the global average of 1.1 °C per century (IPCC 2013; BCME 2016), although some areas have warmed by up to 2.2 °C per century (e.g., Strait of Georgia, Entrance Island) (BCME 2016). In BC waters, recent sea surface temperature trends have been the result of climate change warming, El Niño effects (2015–2016), and the “warm blob” phenomenon (2013–2016) where a large area of very warm water (3 °C warmer than usual) settled off the BC coast (Bond et al. 2015; DiLorenzo and Mantua 2016). In 2016, average sea surface temperatures were 1–2 °C warmer than the historical average within the Northeastern Pacific Ocean (Chandler et al. 2017). Sea surface temperatures are likely to increase by between 0.5 and 2.0 °C by the end of the century (Foreman et al. 2014; Fig. 3).
Ocean acidification
Globally, ocean acidification is projected to increase by 100% or more by 2100, but there is large uncertainty and variation among regions and climate scenarios (IPCC 2013; Haigh et al. 2015; Weatherdon et al. 2016a). Ocean acidification is occurring faster as higher latitude areas, due to the effects of temperature on carbon dioxide absorption (Byrne et al., 2010; Doney et al., 2012; Gerber et al., 2014). In the Northeastern Pacific Ocean, ocean acidification is one of the most urgent threats to both marine ecosystems and human communities as these waters are already among the most acidic of the world’s ocean regions, due to ocean currents and upwelling of deep ocean waters (Okey et al., 2014; Haigh et al., 2015). Within the NSB, acidification is projected to continue to increase as carbon dioxide emissions continue to rise. The existing state of knowledge for ocean acidification is limited, and other than global data sets, there are currently no projections at the scale of the BC coast (Hunter et al. 2015). This is partly due to a lack of empirical data as model inputs. Sampling for alkalinity and dissolved organic carbon has occurred as a regular part of Fisheries and Oceans Canada (DFO) surveys since 1992 and 1986, respectively, but the data have been fragmented and inconsistent (Hunter et al. 2015). Ongoing efforts to model changes in ocean chemistry (nitrogen, carbon, and oxygen) will improve the overall understanding of coastal biochemical processes and nearshore acidification and hypoxia levels (K. Hunter, Fisheries and Oceans Canada, personal communication, July 2017). In addition, further efforts to model changes and extremes in pH (Hunter and Wade 2015; Ianson and Monahan unpublished data) will improve the understanding of projected changes in acidification and the associated impacts.
Ocean deoxygenation
Across the NE Pacific Ocean, dissolved oxygen levels have been declining over at least the past several decades, at a range of depths from 100 to 1000 m deep down to the sea floor, and oxygen levels between 100 and 400 m depth have decreased by 22% over the past 50 years (Okey et al. 2014). Oxygen levels are likely to continue to decline in the northeast Pacific Ocean, and coastal shelf and slope marine ecosystems are likely to lose well oxygenated habitats (Whitney et al. 2007; Fig. 4). Seafloor habitats in the North Pacific could experience a reduction of 0.7%–3.7% in oxygen levels by 2100 (Sweetman et al. 2017).
Sea level rise
In BC, the average sea level between 1910 and 2014 has risen along most of the coast, at a rate of 13.3 cm/century at Prince Rupert, 6.6 cm/century at Victoria, and 3.7cm/century at Vancouver (BCME 2016). Sea levels at Prince Rupert and Victoria continue to increase, while off the west coast of Vancouver Island tectonic uplift offsets sea level rise such that water levels appear to be declining (removing this uplift would result in a sea level increase of 13.5 cm per century) (BCME 2016; Chandler et al. 2017). Changes in sea level threaten coastal systems through coastal erosion, seawater inundation, and contamination of freshwater systems, and they can affect food crops grown in low lying areas. Relative sea level across the coast of BC is projected to rise by an average of 20–30 cm by 2100 (Fig. 3). However, there are many uncertainties in the projections for sea level rise, and local ocean currents, tidal patterns, and river discharge rates will influence the observed rate of sea level rise to particular areas. For instance, some models project higher observed sea surface height (SSH) values along the northern coast of BC during the next century in winter and spring related to wind patterns and increasing river discharge (Foreman et al. 2014).
Sea surface salinity
Ocean salinity is declining globally, along the BC coast, and within the Northern Shelf Bioregion (Chandler 2017). Empirical data from lighthouse station monitoring stations in 2016 reflect a long-term trend towards decreasing sea surface salinity across the BC coast, except within the Strait of Georgia (Chandler 2017). Longer-term data from lighthouse station monitoring shows the same freshening trend; however, this is not associated with longer-term climate trends but with local freshwater sources (Cummins and Masson 2014). Freshwater discharge from glaciers is a significant contributor to the nearshore waters of BC. Glacial coverage in BC has declined since 1985, and the volume of glacial ice declined by an average rate of 21.9 km3 from 1985 to 2000 or by approximately 6.4% from 1985 to 2000 in the coastal region (BCME 2016). Coastal runoff contributes to maintaining the salinity levels along the coast, driven by glaciers as well as watersheds driven by fall and winter seasonal precipitation. Large river systems (Fraser River, Naas River, and Skeena River) drain large watersheds and experience pronounced spring freshets that affect coastal salinity levels more than large-scale climate changes (Cummins and Masson 2014).
Precipitation
Precipitation is a key indicator of climate change, and changes will affect all sectors, ecosystems, and communities. In BC, precipitation has increased over the last 50 years in all seasons by some estimates, or just in the summer months by other reports, with variation across the province (Rodenhuis et al. 2006; Walker and Sydneysmith 2008; Okey et al. 2014). Province-wide, annual average precipitation has increased by 12% per century (BCME 2016). However, these changes have been so far statistically insignificant and can largely be explained by the high natural variation in precipitation patterns across the province (PCIC 2013a; BCME 2016; Vadeboncoeur 2016). Winter warming trends led to higher snowpack density (wetter snow) across much of BC from 1950 to 2014, and as winter temperatures increase, winter snows are likely to continue to be wet and heavy or be replaced by rain (BCME 2016). Changes in the amount, type, and timing of precipitation in BC will certainly affect both terrestrial and marine systems, although the relatively high uncertainty in historical monitoring data—and the implications of these changes—means that estimating current precipitation trends and associated impacts is a challenge (BCME 2016).
Projected changes in precipitation in BC are expected to be relatively minor, especially when compared with the historical variability in the province (Fig. 3). Wetter winters are projected to lead to increased runoff in rivers and streams in the winter. Less precipitation will fall as snow in the winter, which is likely to result in a reduction of the spring snowpack by 55% by 2050 (Rodenhuis et al. 2006). Due to this reduction in snowpack, the spring freshet will likely occur earlier in the spring in many rivers. By the end of the century, spring streamflow will likely have increased significantly, while summer river levels will have decreased (Thomson et al. 2008).
Extreme precipitation events will likely increase during some seasons and in some areas of the province (Rodenhuis et al. 2006; Pike et al. 2008; Mote and Salathé 2010; Okey et al. 2014; Fig. 3). Heavy precipitation events in BC include phenomena known as “atmospheric rivers” where highly concentrated water vapour streams move moisture from tropical regions towards the poles. These occur frequently in the fall and winter in BC, and impact coastal areas with periods of intense precipitation and flood events (Rodenhuis et al., 2006; Pike et al., 2008; Nyland and Nodelman, 2017). More frequent atmospheric river events after 2040 (approximately double the current number per year) will affect ecosystems along the coast (Nyland and Nodelman 2017). Changes in wind and wave patterns may interact with other climate change projections such as sea level rise, but the projections of future winds and storm events are highly uncertain (IPCC 2014b). Storm intensities in the North Pacific increased during 1940–1998, and storm surge related winds and storms may become more frequent and intense as air and ocean temperatures increase (Dolan and Walker 2006; Thomson et al. 2008).
Observed and projected climate change impacts
Ecosystems
Increasing sea surface temperatures and declining oxygen levels have affected the northern Pacific Ocean, which has likely led to reduced habitat availability and decreased survival for many fishes and invertebrates (Whitney et al. 2007). The warm blob (2014–2016), a mass of water characterized by water temperatures from 1 to 3 °C above the 30-year average, developed off the west coast of BC, affecting phytoplankton community composition, ocean stratification, and associated with abnormal and dramatic species range shifts (Chandler et al. 2017). Harmful algae blooms during the warm blob event could also be associated with climate warming (Perry and Peña 2016). During that event, warm water zooplankton were much more abundant than cold water species in 2016, with potential implications for fish as warm water zooplankton species have lower nutrient quality (Perry and Peña 2016). Other unusual biological events during that anomalously warm water period included low chlorophyll levels (2014), potentially due to increased stratification and reduced nutrients (Whitney 2015). Species sightings that could indicate dramatic species range shifts include ocean sunfish and warm water sharks off Washington State and Alaska, high catches of albacore tuna off the Washington and Oregon coasts, juvenile pompano sightings near the Columbia River, and widespread stranding of Velella velella off the coast of BC throughout the summer of 2014 (Bond et al. 2015). Warm ocean temperatures have affected the waters off the west coast of Vancouver Island, and the zooplankton community has shifted to more gelatinous species (overall lower nutrient food) and less crustaceans, leading to poor forage conditions for juvenile fish and seabirds (Galbraith and Young 2017). Further increasing sea surface temperatures are likely to affect zooplankton biomass, contributing to overall biomass declines of lower trophic level species (Hunter and Wade 2015). Increasing ocean temperature and the northerly shift in the California Current may lead to higher abundance of low nutrient zooplankton, which would in turn affect juvenile fish species such as herring and salmonids (Cleary et al. 2016; Galbraith and Young 2017). Phytoplankton species composition is also likely to change, which could also affect higher trophic levels who depend on high-quality zooplankton (Ainsworth et al. 2011; Johnson et al. 2011; Perry and Peña, 2016; Galbraith and Young 2017). Kelps (giant kelp and bull kelp) and eelgrass (Zostera marina) are also likely to be negatively affected by warming sea surface temperatures; the cumulative impact of temperature along with decreasing salinity and increasing sedimentation from runoff will influence the productivity and distribution of these important habitat forming species (Okey et al. 2012). Projected changes to climate variables are likely to interact to affect the likelihood of species invasions in BC waters, as habitats and oceanographic conditions change to facilitate the establishment of warm water species through species range expansions (Bond et al. 2015; Cheung et al. 2015). Marine diseases are also likely to increase in frequency and intensity, as species already stressed due to direct climate changes such as rising ocean temperatures are also more susceptible to infection (Okey et al. 2014). The biological interactions and additive effects of disease and invasive species, such as green crab which is already invading BC waters (Gillespie et al. 2015), are highly uncertain (Clarke Murray et al. 2014; Okey et al. 2014; Jones and Cheung 2017).
Ocean acidification will certainly continue to affect coastal BC, although there are significant knowledge gaps in terms of the effects of ocean acidification on marine organisms (Haigh et al. 2015). Where data exist, it is more reliable for commercially viable shellfish species (e.g., oysters) and issues that may affect human health (e.g., harmful algae blooms) (Haigh et al. 2015). Already, the ocean below 300 m depth is corrosive to aragonite shells, and it is likely that the saturation depth will continue to decrease, threatening shelled organisms and the fishes that feed on them, including Pacific salmon (Cummins and Haigh 2010; Haigh et al. 2015). There is a strong likelihood that the negative impacts associated with ocean acidification could increase rapidly, and that the effects on marine species could potentially cause large shifts in species distribution and community assemblages across both latitude and depth (Sweetman et al. 2017). Ocean acidification may affect habitat availability and the abundance of those species dependent upon calcifying organisms for structural habitats and those whose larval stage is affected by decreasing pH. For example, by 2100, cold water corals are projected to degrade due to ocean acidification, which will affect habitat availability for fish populations, therefore affecting fisheries’ productivity (Sweetman et al. 2017). The effects of ocean acidification on trophic dynamics (food web interactions) and the synergistic effects of ocean acidification with other climate change projections, such as increasing ocean temperature and declining oxygen levels, require further research (Haigh et al. 2015; Hunter et al. 2015).
The expansion of low oxygen zones will affect ecosystem structure and function, especially in the deep sea (Sweetman et al. 2017) and in the deep coastal fjords of the northern coast of BC due to their geomorphological and chemical conditions (Okey et al. 2014). Deoxygenation at deeper depths may drive mobile marine species to seek refuge in shallow water; yet, those habitats will be more likely to be too warm. This interactive effect may result in physiological stress or species interactions. The combination of warming temperatures in shallow depths and deoxygenation at deeper depths may lead to habitat compression, unless species can adapt by migrating along continental slopes into more oxygenated environments, where they exist (Sweetman et al. 2017).
Due to the reduction in snowpack associated with decreased winter precipitation and warmer air temperatures, the spring runoff (freshet) will likely occur earlier in the spring in many rivers, with downstream effects on freshwater habitats and linked marine systems (Foreman et al. 2014; BCME 2016). This is likely to negatively impact the reproductive capacity and survival of fish and other aquatic species (Rand et al. 2006; Okey et al. 2012). The sheer increase in freshwater volume entering coastal marine waters will also affect salinity and stratification of ocean surface waters. Changes in the timing of spring currents are also likely to affect biological interactions such as plankton availability, in turn affecting larval fish and invertebrates (Hunter and Wade 2015). The specifics of this impact of species recruitment are currently unknown (Hunter and Wade 2015).
Increased precipitation may lead to more runoff of contaminants and terrestrially derived nutrients—which, when combined with higher water temperatures, could increase the likelihood of toxic algae blooms in freshwater and marine ecosystems (Hunter and Wade 2015). The increase in freshwater volume entering coastal marine waters may also decrease sea surface salinity and increase ocean surface stratification. These stressors are likely to affect marine fishes and mammals that depend on nearshore habitats and are adapted to current oceanographic conditions (Hunter and Wade 2015). Changes in salinity can affect both sexual reproduction and vegetative propagation of seagrasses (Hunter and Wade 2015; Rahman et al. 2017). Declining salinity levels may affect the habitat, survival, and growth of marine fish and shellfish; in the Arctic, declining salinity has been shown to reduce phytoplankton size, which in turn affects productivity of higher trophic levels (Sweetman et al. 2017).
Sea level rise may affect coastal ecosystems through changes to habitat, especially for nearshore areas if important intertidal habitat becomes permanently sub-tidal, affecting nearshore plants, algae, and shellfish (Hunter and Wade 2015). Generally, coastal sensitivity to sea level rise depends on the physical geology of the coastline, and as such low-lying sandy regions will be most impacted. Most of the shoreline of BC has low sensitivity to sea level rise due to the rocky, fjordal coastline, but some areas (near Prince Rupert, Bella Bella, and most of Vancouver Island) are moderately sensitive (Biffard et al. 2014).
Fisheries
For many coastal communities and First Nations, fishing is a way of life that cannot be measured by the contribution to the economy alone, as social and cultural values play a large role. The fishing industry has already declined significantly across the province because of multiple factors including overfishing and fleet restructuring; since the 1980s, the fishing fleet has shrunk by 60% and there are 70% fewer fishers employed in the industry (Foundation TBSE 2015). Existing stresses and impacts on BC’s coastal fisheries are expected to be exacerbated by climate change, in particular for those species that are highly vulnerable to warming conditions (e.g., salmon; Healey 2011; Cheung et al. 2015; Weatherdon et al. 2016b). Some of the effects of climate change on fisheries in this region are reflected in projected species range shifts: the abundance of current target species is projected to decrease, and the species currently available across the region are likely to change (Ainsworth et al. 2011; Cheung et al. 2015; Teh et al. 2016; Weatherdon et al. 2016b). The impacts of these projected changes in fisheries catches could lead to or amplify other socio-economic impacts of climate change on fisheries and communities through reduced food security and economic loss (Weatherdon et al. 2016b). Fisheries and Oceans Canada has an ongoing project to model climate change vulnerabilities in some fisheries, and other work is focused on modeling the impacts of warming river temperatures on juvenile salmon (K. Hunter, Fisheries and Oceans Canada, personal communication, July 2017).
In southern BC, the impacts of warming freshwater and marine water temperatures have been observed, leading to declining productivity and diminished returns of Fraser River sockeye salmon (Walker and Sydneysmith 2008). Low returns of Fraser River sockeye salmon have been observed (e.g., returns in 2016 were the lowest on record) (Grant et al. 2017), and Fraser River sockeye were recently recommended for listing with the Species at Risk Act (Semeniuk 2017). While observations and field evidence are still limited, the interacting effects of ocean temperature and acidification are also of critical concern for many taxa (e.g., molluscs, corals, and calcareous algae). Ocean acidification has affected calcifying organisms, especially larval survival in the oyster aquaculture industry (e.g., Strait of Georgia; Hunter et al. 2015; Ianson et al. 2016a, 2016b). Melting glaciers across BC may also be releasing historical pollutants into freshwater ecosystems, also affecting freshwater fish and downstream marine areas (Whitney 2017). Increasing rates of ocean acidification are likely to negatively affect calcifying organisms, many of which are important for aquaculture and traditional food resources, including molluscs, bivalves, sea urchins, and sea cucumbers (Haigh et al. 2015; Ianson et al. 2016a).
Further fisheries responses to climate change impacts will vary both regionally and by species (Walker and Sydneysmith 2008; Table 1). Species particularly vulnerable to increasing ocean temperatures include Pacific salmonids (Crozier et al. 2008; Healey 2011; Martins et al. 2011; Muñoz et al. 2014). For example, there is a 17%–98% chance of “catastrophic” loss of a Chinook salmon population by 2100, depending on the emissions scenario (Muñoz et al. 2014). Many fish species may expand their range northward (at an average rate of 10–18 km/decade), and in many cases, their abundance may decline precipitously (Walker and Sydneysmith 2008; Ainsworth et al. 2011; Weatherdon et al. 2016b). These impacts are more severe at higher latitudes, so that the relative catch potential is projected to decline more in the North Coast, Haida Gwaii, and Central Coast than southern BC waters (Weatherdon et al. 2016b). Pelagic fisheries that may be especially affected by increasing sea surface temperature include Pacific herring, Pacific sardine, and Pacific salmon (Hunter and Wade 2015; Weatherdon et al. 2016b). Warming ocean surface temperatures will also likely decrease the habitable range of sessile species such as shellfish, which are less likely to be able to move northward at the rate of climate change. However, species’ responses to climate change will also depend on species-specific adaptive capacity and sensitivity; assessing species-specific responses to climate change is highly limited by the lack of data on species traits and potential adaptive responses (Jones and Cheung 2017).
Table 1.
Fisheries species of interest | Ecological variable affected by climate change | Probable impacts |
---|---|---|
Sablefish (Anoplopoma fimbria) | Ocean temperature | May negatively affect egg, larval and juvenile survival. Declining ocean productivity may affect recruitment. Overall: climate change over the next 50 years may not impact adult sablefish or long term population dynamics. Projected change in relative abundance of −9% to −11% by 2050. |
Pacific herring (Clupea pallasii) | Ocean temperature | Herring ocean habitats will be affected. Predation from increasing populations of Pacific hake may affect herring populations. Overall: abundance is projected to decline by 32%–49% by 2050. |
Pacific hake (Merluccius productus) | Ocean temperature | Hake biomass may increase. More migration into northern range (BC waters). Overall: abundance may increase. |
Pacific halibut (Hippoglossus stenolepis) | Ocean temperature | Overall: nay reduce recruitment. Projected change in relative abundance of −12% to −13% by 2050. |
Pacific ocean perch (Sebastes alutus) | Ocean temperature | Overall: recruitment may decline if Aleutian Lows decrease. |
Pacific sardine (Sardinops sagax) | Ocean temperature Oceanographic conditions | Unlikely to impact or unknown. Projected change in relative abundance of 33%–44% by 2050. |
Pacific cod (Gadus macrocephalus) | Ocean temperature | Likely to severely deplete populations by reducing or eliminating recruitment. Projected change in relative abundance of −13% to −35% by 2050. |
Pink salmon (Oncorhynchus gorbuscha) | Ocean temperature River temperatures Oceanographic conditions | Overall: abundance is likely to decline, particularly for southern populations. Projected change in relative abundance of −40% to −44% by 2050. |
Sockeye salmon (Oncorhynchus nerka) | Ocean temperature River temperatures Oceanographic conditions | Mortality is likely to increase during all life stages when exposed to warm water temperatures. Overall: abundance is very likely to decline, particularly for southern populations. Projected change in relative abundance of −10% to −36% by 2050. |
Chum salmon (Oncorhynchus keta) | Ocean temperature River temperatures Oceanographic conditions | Mortality is likely to increase, but uncertainty is high. Overall: abundance is likely to decline, particularly in the southern portion of the coast. Projected decline in relative abundance of 10%–12% by 2050. |
The effects of ocean acidification on fisheries are largely unknown, including on the important salmon and Pacific halibut fisheries (Haigh et al. 2015; Hunter et al. 2015; Table 1). In tropical fish, ocean acidification affects behavior and results in increased mortality, but species responses in temperate regions are unknown (Haigh et al. 2015). Adult fish may be more tolerant of ocean acidification than early development stages, but there is limited research on life-stage specific responses to pH for BC fishes specifically (Haigh et al. 2015). The effects of ocean acidification on shelled organisms, however, are much more understood (Cooley et al. 2009; Gazeau et al. 2013; Ekstrom et al. 2015; Haigh et al. 2015). Acidification will affect fisheries through negative impacts on food web dynamics and lower trophic level organisms (Lynn et al. 2013). Shellfish are likely to be affected across life stages, and this decline in economically important species (oysters, scallops, abalone, and mussels) will affect the economies of coastal communities in the region. In the Northern Shelf Bioregion, aquaculture is of great interest to coastal communities and First Nations. Ocean acidification is likely to negatively affect shellfish aquaculture (Gazeau et al. 2013), and changes to oceanographic conditions including acidification are likely to affect finfish aquaculture as well (Haigh et al. 2015). The specific effects of ocean acidification on shelled molluscs vary by species, and a recent review suggested that acidification most negatively affects survival and shell growth (calcification), followed by respiration and clearance rates (Gazeau et al. 2013).
Ocean deoxygenation will affect commercial fish species by reducing high-quality fish habitat. Declining oxygen levels across the Pacific Ocean will contribute to the general decline and potential collapse of sessile (immobile) marine species, or sediment-dwelling organisms, as well as any other species who are intolerant of low oxygen levels. Declining oxygen levels are likely to especially affect groundfish species, whose deep water habitat seems to already be decreasing by 2–3 m per year as deoxygenated strata increase (Cummins and Haigh 2010). Declining oxygen levels, and increasing hypoxia, will also likely affect aquaculture operations for vulnerable species, such as Dungeness crab and spot prawns (Hunter and Wade 2015). Some hypoxia tolerant species (e.g., squid and jellyfish) may increase in abundance and (or) distribution, potentially outcompeting less tolerant species (e.g., finfish) (Doney et al. 2012; Sweetman et al. 2017).
The associated impacts of sea level rise on land erosion and increased runoff is likely to directly affect nearshore species. Existing shellfish beds are likely to be affected, which will have implications for many species that depend upon the intertidal ecosystem for habitat and food, especially as juveniles (salmon, crab, eelgrass, algae, clams, and humans) (Lynn et al. 2013). Spawning habitat for forage fish, and rearing habitats for invertebrates, could decrease or be lost due to erosion, subsidence, and submersion due to sea level rise (Hunter and Wade 2015). This may be particularly problematic for Pacific herring as coastal marine algal species and habitats used for spawning may be lost as sea levels rise (Hunter and Wade 2015).
Communities and marine infrastructure
Coastal First Nations and non-First Nations communities depend on the ocean and nearshore environments for economic, social, and cultural values. The coastal economy of British Columbia is largely based on the recreational tourism (33%), transport (29%), and seafood (12%) sectors (Foundation TBSE 2015, 2017). These communities are at risk for land loss, damage to coastal infrastructure, and changes to resource availability, all of which will affect coastal values (Walker and Sydneysmith 2008). Especially for First Nations communities, access and availability of traditional foods has decreased as ecosystems have been exploited or converted to other uses, and the productivity of remaining intact ecosystems has been impacted by factors including pollution, management actions, or invasive species. An example of an observed impact of climate change on community food security is the changing timing of wild plant ripening and harvest (Turner and Clifton 2009; Lynn et al. 2013). Increasing ocean temperatures will impact coastal communities that are reliant on fisheries and other marine species for food security and economic activities. Many species that are currently important to coastal communities (First Nations and non-First Nations) are projected to shift northwards from their current species range (at rates of 10–18 km/decade) and also decline in relative abundance (up to ∼40% decline depending on the species and climate scenario) (Weatherdon et al. 2016b). Decreased access to these traditional foods also has implications for human health and culture (CIER 2006).
Increasing storm surge events have been observed along the BC coast, along with an increasing frequency of “king tide” events. First Nations cultural sites may be impacted by rising sea levels, storm surge, and king tide events. For example, cultural sites near Prince Rupert and Metlakatla are experiencing erosion and loss of cultural artifacts (A. Paul, personal communication, 24 November 2017). First Nations communities have observed changes to seasonality of local food gathering practices (Turner and Clifton 2009). Increasing sea levels may be affecting coastal built infrastructure (Walker et al. 2006; Sales 2009), and increasing frequency and intensity of storm events may affect marine infrastructure (at sea and near-shore; Walker and Sydneysmith 2008). Increasing intensity and frequency of storms is likely to increase inundation risk (flooding) and erosion risk to marine infrastructure, especially for low-lying communities (Barnard et al. 2015). Extreme weather events are expected to create disruptions to marine transportation lanes, potentially lead to wave and wind damage to infrastructure and utilities, and reduce access to critical services (Andrey and Palko 2017). Extreme precipitation events may damage fixed coastal infrastructure such as airports (e.g., Sandspit Airport on Haida Gwaii) and ports (e.g., Port of Prince Rupert) (Andrey and Palko 2017; Nyland and Nodelman 2017).
Most communities within the NSB are highly dependent on marine infrastructure. The greatest threats to marine infrastructure in the NSB are likely to be sea level rise and increasing extreme weather events. Sea level rise will not affect areas along the BC coast equally because of differences in vertical land movement (Warren and Lemmen 2014b). Notable cases include the northeastern coast of Graham Island, Haida Gwaii, an area which is amongst Canada’s most sensitive coastlines to climate change (Walker and Sydneysmith 2008). In addition, groundwater quality could decline due to saltwater intrusion as sea levels rise (Walker and Sydneysmith 2008). Sea level rise already threatens coastal infrastructure, and the added risk of storm surge flooding increases the vulnerability of coastal infrastructure across the province (Lemmen et al. 2016; Nyland and Nodelman 2017).
Overall, climate-related impacts to marine infrastructure are likely to be high, especially as many communities in coastal BC already have infrastructure deficits that will require increased investment (Foundation TBSE 2015, 2017). Future winter and spring SSH levels, which can be due to the expansion of warming waters, are also projected to be consistently higher, which will further exacerbate the flooding impacts of sea level rise (Foreman et al. 2014). Large peak flows and storms can interrupt delivery of goods such as fuel and food to remote places. On the other hand, climate change may also offer some opportunities for the marine transportation sector: longer construction seasons and reduced winter maintenance could reduce costs and increase annual operating budgets. Over time, increased sea levels may mean that vessels with deeper draughts will be able to enter existing ports (Andrey and Palko 2017).
Climate change impacts are likely to continue to unevenly affect communities along the coast because of different exposures to those impacts. An added dynamic is that any climate-related impact has a cumulative effect on nonclimatic issues already affecting these communities, such as declining resource industries, economic or social restructuring, and ongoing land claims agreements in the case of First Nations. Other impacts on human communities include changing energy demands for heating, which have declined for buildings across BC, especially in northern BC, as average air temperatures have increased (BCME 2016). It is likely that increasing air temperatures, especially during extremely high temperature events, will lead to greater incidence of heat stroke and potential mortality (Henderson et al. 2013). However, increasing air temperatures may attract more tourism for a longer summer season, which will have positive economic implications for coastal communities. In general, rural and remote communities in BC tend to have a lower socio-economic index, indicative of economic hardship, education, health, and other risk factors (Huang et al. 2012; Islam et al. 2014). These trends suggest low adaptive capacity to manage large stressors like climate change (Foundation TBSE 2015).
Adaptation options
In this section, we describe general adaptation strategies that could be applied to the context of coastal BC that build on existing programs and attributes (Table 2). Governments and communities need to remain open to communication and collaboration to develop tools that enable effective adaptations at regional and sub-regional scales. This included integrated and adaptive management practices that increase access to and distribution of resources, such as allocating roles and responsibilities, distribution of relief goods and provision of relief services; developing early warning and evacuation systems; creating education and awareness programs; and making arrangements for secure shelter and food, and access to health care, education, economic, and social resources (Adger et al. 2005; Pelling and High 2005; Green et al. 2006; Pelling et al. 2008; Klein et al. 2014). Here, we present some options that could translate to the social–ecological setting of the North Pacific Coast, relevant to each of the four areas of interest we focused on.
Table 2.
Climate change impact | Regional impacts, by sector | Suggested adaptation actions and some regional research gaps |
---|---|---|
Warming ocean temperatures | Fisheries: shifting species ranges | Consider adaptive or dynamic ocean management (Maxwell et al. 2015; Mills et al. 2015) Fisheries: adapt fisheries allocations after species abundance/distributions have changed (Morrison and Termini 2016) Adjust fishing practices or gears to new species or changing fishery openings (Morrison and Termini 2016) |
Ocean acidification | Aquaculture: declining productivity, larval survival, and reproductive capacity of calciferous organisms | Consider land-based saltwater flow-through aquaculture systems as an alternative to at-sea net pen aquaculture systems |
Sea level rise | Ecosystems: intensifying storms and warming oceans will affect coastal regions through erosion and degradation of shorelines and wetlands | Land-sea parks: nearshore habitat protection can reduce loss and damage, and promote intact habitats that offer natural coastal defense, ultimately supporting human livelihoods and ecological recovery (Roberts et al. 2017) |
Wind, waves, and storm events | Higher coastal erosion and flooding | Apply a precautionary approach: use extreme recurrences for both winds and water levels in setting scenarios for mitigation and adaptation. Research gaps: impacts of storm surges, especially on low lying regions. More modeling is needed to improve projections of storm events and impacts including changes in frequency, intensity, and direction of future extreme events. |
Ecosystems, fisheries, and aquaculture
As fish distributions and abundances shift with climate change, fisheries will have to adjust by changing target species, fishing areas, fisheries openings, and processing locations (Pinsky and Mantua 2014; Morrison and Termini 2016). Scenario planning exercises can help to identify management options to move the spatial extent of fisheries or add value to fishery products if landings are projected to decline. For instance, adjusting fisheries regulations and harvest licensing prior to species shifting their range could allow fishers to target new species and exotics as opportunities arise (Walker and Sydneysmith 2008; Johnson and Welch 2009; Morrison and Termini 2016). As the distribution of target species changes, it is also important to adapt fisheries infrastructure and increase the flexibility of processing and supply chains for fisheries to reduce the impact on local fishing-based economies (Morrison and Termini 2016). Insuring fishers to provide stability in low income years and decrease ratcheting up and overfishing, analogous to crop insurance, could help to support fishing-based communities. Marine conservation tools such as MPAs can also be adapted to climate change by protecting both the current and future habitats of priority species, or by using dynamic reserves where the boundaries change over time (e.g., dynamic ocean management) (Hobday et al. 2013; Creighton et al. 2016). Fisheries management that promotes the adaptive capacity of target fish species and populations can also help to support fisheries adaptation. Specific examples include population-based fisheries management, which depends on understanding the genetic diversity of fish species and population distributions, to protect weak populations or populations on the edges of species distributions (Perry et al. 2010; Pinsky and Fogarty 2012). Fisheries management can also aim to protect fish population age structure to increase resilience to changing environmental conditions by using size limits, modifying fishing gear (e.g., net size and reducing post-release mortality of nontarget individuals; Morrison and Termini 2016).
In some cases, reactive management approaches may be suitable or successful given that predictive modeling of future environmental conditions and associated fisheries may be uncertain (Johnson and Welch 2009; Miller et al. 2010; Morrison and Termini 2016).
Reactive management approaches for fisheries and fishing-based communities include flexible and adaptive management systems that reward innovation, coordination, and collaboration between regions and management bodies. Increased monitoring and use of indicators can help to prepare managers and planners as conditions change and management needs shift, for example to manage dynamic spatial closures to adjust fishing activity based on real-time data on environmental conditions and fish distributions. Adjusting fisheries reference points to reflect changes in species or stock abundance depends on high-quality monitoring data of fisheries and environmental variability but would be effective in supporting fisheries abundance as target species ranges and abundance shifts (Botsford et al. 2009; Morrison and Termini 2016; Young et al. 2019). If species distributions or abundances have already changed, adjusting fisheries allocations can support adaptive fisheries management, but this depends on high-quality monitoring and modeling of species distributions and abundances and clearly defined allocation rules based on indicators of change (Young et al. 2019). Finally, adjusting fishing practices or fishing gear once fish communities change is also a reactive adaptation strategy. As fish species distributions and abundances change in response to climate impacts, fishers will adjust their fishing practices or gear to reduce interactions with nontarget stocks or protected species. This strategy can have negative tradeoffs based on economic costs and changes to social structure of communities (Johnson and Welch 2009; Young et al. 2019). It also depends on involving fishers early in management discussions (Yates 2014). First Nations may have fewer options for adaptation, especially given their dependence on marine resources for food security and cultural values (Walker and Sydneysmith 2008; Turner and Clifton 2009; Weatherdon et al. 2016b). In these cases, Indigenous knowledge may offer examples of adaptive strategies to enhance food security and thus community resilience in these systems. As an example, recent research on clam garden mariculture illuminated the historical importance of First Nations aquaculture on this coast (Pinkerton et al. 2014; Jackley et al. 2016). Learning from these traditional techniques may offer adaptive strategies for communities now and in the future.
Communities and marine infrastructure
Building adaptive capacity and resilience in remote communities is dependent on a variety of factors, including: (i) existing local and regional institutional capacity, (ii) local socio-economic development, (iii) infrastructure development and condition, and (iv) local experience with extreme weather events and other environmental or socioeconomic stress (Pelling and High 2005; Butler et al. 2015). Other factors that can contribute include social networks and cohesion, income diversification, and self-reliance (Walker and Sydneysmith 2008). However, current social and economic stressors are likely to reduce the capacity for remote communities to undertake or even consider climate change adaptation. Many coastal communities within the region already experience economic hardship and are limited in their capacity to undertake new initiatives or projects, even if the long-term benefits are relevant. Building on initiatives that are already in place to address environmental and economic changes and incorporating considerations of the impacts of climate change should be more effective for those communities (Walker and Sydneysmith 2008).
In BC, the provincial government has conducted vulnerability assessments for highway systems and continues to monitor sea level rise. The British Columbia Ministry of Transportation and Infrastructure is one of the first jurisdictions to require infrastructure design work for the ministry to include climate change implications. However, infrastructure operators in BC have primarily been responding reactively, rather than anticipating and proactively preparing for projected changes or impacts (Nyland and Nodelman 2017). This approach typically results in impacts being more costly or severe than if proactive adaptation actions had been taken (White and O’Hare 2014). Some communities in BC have begun to take action to reduce the risk of sea level rise through investments in built infrastructure for shoreline protection as an adaptation measure (Lemmen et al. 2016). Further adaptation examples can be found in urban areas near the Fraser River floodplain, an area that is highly vulnerable to sea level rise due to low-lying geology and dense population (Yumagulova and Vertinsky 2017). A recent analysis found that the costs of sea level related damage to on-shore built infrastructure would be higher in coastal BC than any other coastal region in Canada (Withey et al. 2016). Regional models of sea level rise and seasonal climatic variability patterns would improve the ability of coastal managers to predict flood hazards and associated risk factors for the region.
Adaptive capacity building at the community level should be supported by national, regional, and sub-regional institutions and policies. Regional and sub-regional management should understand how local communities and local institutions function and are managed to support local adaptation actions and community resilience to major change. A transparent knowledge- and data-based climate change adaptation process can enhance existing adaptive capacities (Pelling 1997; Pelling and High 2005; Berke et al. 2008; Miller et al. 2010; Murphy 2014). Particularly, by empowering First Nations, socio-economic groups that may be already vulnerable can be included in decision-making processes (Green et al. 2006; Cutter et al. 2008). Especially for First Nations communities, past experiences with historical social, cultural, or economic changes can offer lessons for adapting to climate change. Key attributes of social adaptive capacity such as social capital and community networks are often already evident in place-based communities and community ties. However, climate change impacts and adaptation needs to be seen as relevant for present-day local community planning and management (Walker and Sydneysmith 2008; Reid et al. 2014). While attributes of resilience may exist inherently, the impacts of climate change challenge long-term adaptive capacities through rapidly rising sea levels and coastal storms, new impacts that threaten coastal communities who are dependent on vulnerable infrastructure and may lack essential relief services (Walker and Sydneysmith 2008). Additional financial support to cope with these increasing impacts, with land use planning that considers climate change, can improve the adaptive capacity of coastal communities.
To date, most climate change related adaptations documented in British Columbia have been reactive responses to unpredicted events, such as extreme forest fires or the mountain pine beetle epidemic (Parkins and MacKendrick 2007; Baynham and Stevens 2014; Government 2017). Planning for climate change seems to compete with a host of other priorities for the limited capacity of local and regional governance, as only one of the many stressors that affect the ecosystems, industries, and communities of British Columbia. For these reasons, cumulative impacts assessment (Halpern et al. 2008; Ban et al. 2010; Clarke et al. 2015a, 2015b) may be highly applicable for regional (and (or) sub-regional) adaptation planning. Proactive adaptation examples for coastal marine infrastructure include investments in early warning systems, particularly for storm surge related activities. Additional options include planning for emergency management, such as alternative routes for evacuations, identifying infrastructure under risk (roads, docks, rail routes, etc.), and programs to either mitigate impacts, or retreat and relocate if necessary.
Discussion
Effective climate change adaptation requires a strategic stepwise approach that includes identifying climate impacts and adaptation strategies. Here we presented an initial assessment of vulnerability and risk in the NSB that can help identify adaptation actions. We aimed to: (i) develop a baseline of understanding of the expected climate changes and associated impacts across coastal BC and the NSB over the coming decades and (ii) provide targeted adaptation strategies and actions that apply to specific areas of interest to a marine planning process. We also described the process whereby we worked with a marine planning team to develop reporting on climate change impacts and provide strategic recommendations. Our research is a starting point for additional work on climate changes, vulnerability and risk assessments, and climate change adaptation efforts in the NSB. The climate changes—both observed and projected—outlined in our research are likely to result in multiple impacts to the key focal areas in the NSB. Long-range planning and early, proactive adaptation efforts will be very important to accommodate predicted and also unanticipated impacts. This is also the baseline for communicating likely climate change impacts, as the initial report (with more detail than this manuscript) is being developed into an interactive web-based portal as a communications tool for sharing climate change impacts and adaptation recommendations with local and sub-regional managers and communities (to be available on the MaPP website; mappocean.org).
Our approach of synthesizing a range of climate change impacts relevant to particular areas of interest, with direction by a marine planning organization, is intended to be actionable within the NSB but also comparable with other regions undertaking similar processes. The level of cross-sectoral assessment that we provided is atypical; more often, climate change assessments focus on a particular sector or specific climate change impact (e.g., flood control for sea level rise; Yumagulova and Vertinsky 2017). The social–ecological implications of climate change are not novel in and of themselves; the impacts of warming oceans, increasing air temperatures, ocean acidification, and sea level rise are well known (e.g., Foreman et al. 2014; Savo et al. 2017; Frölicher et al. 2018; Pinsky et al. 2019). By bringing together research on four key areas of interest, in collaboration with a regional planning and plan implementation partnership, our research provides a more complete picture of the scale and scope of climate change impacts across social and ecological spheres within this region. We highlight the lack of regionally downscaled information available within this management scale (of the NSB), and in particular, the lack of readily available information for ocean and coastal areas (vs. terrestrial). Improved climate projections and vulnerability and risk assessments would advance integrated decision-making and marine planning supporting healthy coastal communities and economies. In addition, proactive planning for adaptation based on these climate projections and associated risks would decrease the likely impacts on all areas and sectors.
Uncertainties and knowledge gaps
There is still a great deal of uncertainty in global climate change projections for coastal regions, and the associated potential impacts, vulnerabilities, and risks (IPCC 2014a; Cheung et al. 2016; Oppenheimer et al. 2016). Part of this uncertainty is due to lack of or limited access to reliable and continuous data. Statistical downscaling can be used to map coarse-scale global climate model outputs to finer scale (regional or local) detail, using the empirical relationship between observed climatology at the finer resolution and coarse-scale model fields. Regional climate models can also produce local projections, which may better represent the local responses to climate change (Salathé et al. 2007). This uncertainty in global climate projections and knowledge of the implications for local vulnerabilities and risk is further amplified by regional variations in local climate which interact with climate change, such as the El Niño—Southern Oscillation (ENSO) and Pacific Decadal Oscillation (PDO) (IPCC 2014b; Okey et al. 2014; Fleming et al. 2017). Particular knowledge gaps both globally and in our study region include extreme weather events including storm surges (Nyland and Nodelman 2017; Sainsbury et al. 2018) and sea level rise (ACCASP 2017). A better understanding of the interactions between climate change projections and the impacts thereof will improve the ability of regions, communities, and sectors to manage infrastructure and development in a way that facilitates effective adaptation to the risks associated with sea level changes. While there are likely to continue to be uncertainties and knowledge gaps, understanding vulnerability and risk is more meaningful in smaller scales as characteristics of each community, their socio-economic structure, coastal context, environmental conditions, and institutional capacity contributes to their local vulnerability. Therefore, for uncertainties surrounding vulnerability and risk, the main knowledge gap lies with the lack of existing studies investigating the local and regional exposure and sensitivity to various climate change impacts. Additional uncertainties in projecting climate impacts derive from the uncertain probability and extent of emissions reductions at a global scale, and therefore the extent of climate changes.
Next steps and conclusions
The partnership approach that we used was aimed at directing future efforts for climate change vulnerability assessment and adaptation planning. These types of efforts can help practitioners and policy-makers to understand priority risks and to identify and implement actions to increase adaptive capacity with a region or sector (Becker et al. 2018). Even more importantly, developing a dialogue around climate change adaptation will require engaging multiple sectors, governments, and society. For example, further south, the Cascadia Partner Forum is working to bring together both science and people to explore future scenarios, explore adaptation options, and broadly develop a shared adaptation strategy by focusing on specific priority issues within a transboundary region (Forum 2019). By developing the initial report into a public facing interactive web portal (northernshelf.docu.li/), we hope that the process, key outcomes, and recommendations from this work make a larger contribution than a report would alone. Investments in monitoring and research will also improve our understanding of climate change projections and impacts across the coastal region and at finer scales such as the MaPP sub-regions. Within BC, monitoring of climate change related variables, such as sea surface temperature and salinity, is underway within the MaPP sub-regions carried out by Guardian programs, and there are plans to advance regional-level monitoring. Additional monitoring programs through the Hakai Institute (hakai.org/) and MEOPAR (meopar.ca/) are in progress to monitor key climate change indicators including ocean acidification.
Climate change is an inherently dynamic threat with both continuous and discrete impacts on the marine environment. Above all, it is imperative that climate change mitigation—reducing greenhouse gas emissions—is prioritized to minimize the effects of climate change. When new global projections are available from the IPCC, and new regionally downscaled projections are available within BC and the Northern Shelf Bioregion, a more specific understanding of the multiple impacts of climate change may be possible. Vulnerability and risk assessments may be conducted to set regional priorities for further research and monitoring, to understand the economic costs of climate impacts, and to inform planning and implementation of adaptation actions. However, acting now on climate change adaptation is important to prepare for the predicted changes ahead, to increase resilience, and to ensure that economic, social, and cultural opportunities are still available to the communities of the NSB. We emphasize that given the uncertainty that currently exists in climate change projections and associated impacts, adaptation actions should be chosen that support resilient social–ecological systems and are flexible to allow management and communities to evolve as climate change unfolds.
Acknowledgements
Thank you to all the researchers who generously shared their time with us to offer insights on the status of climate change research and future recommendations in this area. We thank Emily Goodman for creating the map. This was initially a report for the Marine Plan Partnership for the North Pacific Coast—MaPP. Funding was provided by Tides Canada Initiatives-MaPP Implementation Funding Support Project (grant No. GI00128). Thank you to all MaPP Partners for helping to scope this work and for providing valuable feedback throughout the process.
References
ACCASP. 2017. Aquatic climate change adaptation services program [online]: Available from dfo-mpo.gc.ca/science/rp-pr/accasp-psaccma/projects-projets/022-eng.html.
Adger WN, Arnell NW, and Tompkins EL. 2005. Successful adaptation to climate change across scales. Global Environmental Change, 15: 77–86.
Ainsworth C, Heymans J, Pitcher T, and Vasconcellos M. 2002. Ecosystem models of northern British Columbia for the time periods 2000, 1950, 1900 and 1750. UBC Fisheries Centre Research Reports, 10: 1–41.
Ainsworth CH, Pitcher TJ, Heymans JJ, and Vasconcellos M. 2008. Reconstructing historical marine ecosystems using food web models: Northern British Columbia from Pre-European contact to present. Ecological Modelling, 216: 354–368.
Ainsworth CH, Samhouri JF, Busch DS, Cheung WWL, Dunne J, and Okey TA. 2011. Potential impacts of climate change on Northeast Pacific marine foodwebs and fisheries. ICES Journal of Marine Science, 68: 1217–1229.
Alagador D, Cerdeira JO, and Araújo MB. 2014. Shifting protected areas: scheduling spatial priorities under climate change. Journal of Applied Ecology, 51: 703–713.
Andrey J, and Palko K. 2017. Introduction. In Climate risks and adaptation practices for the Canadian transportation sector 2016. Edited by K Palko and DS Lemmen. Government of Canada, Ottawa, Ontario. pp. 2–10.
Araújo MB, Cabeza M, Thuiller W, Hannah L, and Williams PH. 2004. Would climate change drive species out of reserves? An assessment of existing reserve-selection methods. Global Change Biology, 10: 1618–1626.
Ban NC, Alidina HM, and Ardron JA. 2010. Cumulative impact mapping: advances, relevance and limitations to marine management and conservation, using Canada’s Pacific waters as a case study. Marine Policy, 34: 876–886.
Barnard PL, Short AD, Harley MD, Splinter KD, Vitousek S, Turner IL, et al. 2015. Coastal vulnerability across the Pacific dominated by El Nino/Southern Oscillation. Natural Geoscience, 8: 801–807.
Baynham M, and Stevens M. 2014. Are we planning effectively for climate change? An evaluation of official community plans in British Columbia. Journal of Environmental Planning and Management, 57: 557–587.
BCME. 2016. Indicators of climate change for British Columbia. Ministry of Environment, Victoria, British Columbia.
Beamish RJ. 2008. Impacts of climate and climate change on the key species in the fisheries in the North Pacific. PICES Scientific Reports, 246.
Becker A, Ng AKY, McEvoy D, and Mullett J. 2018. Implications of climate change for shipping: ports and supply chains. Wiley Interdisciplinary Reviews: Climate Change, 9: 1–18.
Beier P, Hansen LJ, Helbrecht L, and Behar D. 2017. A how-to guide for coproduction of actionable science. Conservation Letters, 10: 288–296.
Berke PR, Chuenpagdee R, Juntarashote K, and Chang S. 2008. Human-ecological dimensions of disaster resiliency in Thailand: social capital and aid delivery. Journal of Environmental Planning and Management, 51: 303–317.
Biffard D, Stevens T, Rao A, and Woods B. 2014. BC parks shoreline sensitivity model. BC Ministry of Environment, Victoria BC.
Bond NA, Cronin MF, Freeland H, and Mantua N. 2015. Causes and impacts of the 2014 warm anomaly in the NE Pacific. Geophysics Research Letters, 42: 3414–3420.
Bone B. 2018. Overview of marine protected areas in the eastern Canadian Arctic and their ability to mitigate current and future threats. Dalhousie University, Halifax, NS.
Botsford LW, Brumbaugh DR, Grimes C, Kellner JB, Largier J, O’Farrell MR, et al. 2009. Connectivity, sustainability, and yield: bridging the gap between conventional fisheries management and marine protected areas. Reviews in Fish Biology and Fisheries, 19: 69–95.
Brown JM, Morrissey K, Knight P, Prime TD, Almeida LP, Masselink G, et al. 2018. A coastal vulnerability assessment for planning climate resilient infrastructure. Ocean Coastal Management, 163: 101–112.
Butler JRA, Wise RM, Skewes TD, Bohensky EL, Peterson N, Suadnya W, et al. 2015. Integrating top-down and bottom-up adaptation planning to build adaptive capacity: a structured learning approach. Coastal Management, 43: 346–364.
Byrne RH, Mecking S, Feely RA, and Liu X. 2010. Direct observations of basin-wide acidification of the North Pacific Ocean. Geophysics Research Letters, 37: L02601.
Canadian Centre for Climate Modelling and Analysis. 2017. Climate model data overview. Government of Canada [online]: Available from climate-modelling.canada.ca/climatemodeldata/data.shtml.
Carpenter S, Walker B, Anderies JM, and Abel N. 2001. From metaphor to measurement: resilience of what to what? Ecosystems, 4: 765–781.
Chandler P. 2017. Sea surface temperature and salinity trends observed at lighthouses and weather buoys in British Columbia, 2016. In State of the physical, biological and selected fishery resources of Pacific Canadian Marine Ecosystems in 2016. Edited by PC Chandler, SA King, and J Boldt. Fisheries and Oceans Canada, Sidney BC.
Chandler PC, King SA, and Editors JB. 2017. State of the physical, biological and selected fishery resources of Pacific Canadian marine ecosystems in 2016. Canadian Technical Report of Fisheries and Aquatic Sciences, 3225.
Chandler PC, King SA, and Perry RI. 2016. State of the Physical, Biological and Selected Fishery Resources of Pacific Canadian Marine Ecosystems in 2015. Sidney, BC, Canada.
Cheung WWL, Brodeur RD, Okey TA, and Pauly D. 2015. Projecting future changes in distributions of pelagic fish species of Northeast Pacific shelf seas. Progress in Oceanography, 130: 19–31.
Cheung WWL, Jones MC, Reygondeau G, Stock CA, Lam VWY, and Frolicher TL. 2016. Structural uncertainty in projecting global fisheries catches under climate change. Ecological Modelling, 325: 57–66.
Cheung WWL, Lam VWY, Sarmiento JL, Kearney K, Watson R, and Pauly D. 2009. Projecting global marine biodiversity impacts under climate change scenarios. Fish and Fisheries, 10: 235–251.
CIER. 2006. Report 2: how climate change uniquely impacts the physical, social, and cultural aspects of First Nations.
Clarke C, Agbayani S, Alidina HM, and Ban NC. 2015a. Advancing marine cumulative effects mapping: an update in Canada’s Pacific waters. Marine Policy, 58: 71–77.
Clarke C, Agbayani S, and Ban NC. 2015b. Cumulative effects of planned industrial development and climate change on marine ecosystems. Global Ecology and Conservation, 4: 110–116.
Clarke Murray C, Agbayani S, and Ban NC. 2015. Cumulative effects of planned industrial development and climate change on marine ecosystems. Global Ecology Conservation, 4: 110–116.
Clarke Murray C, Gartner H, Gregr EJ, Chan K, Pakhomov E, and Therriault TW. 2014. Spatial distribution of marine invasive species: environmental, demographic and vector drivers. Diversity and Distributions, 20: 824–836.
Cleary J, Grinnel M, Daniel K, Thompson M, and Boldt J. 2016. Pacific herring in British Columbia, 2016. In State of the physical, biological and selected fishery resources of Pacific Canadian marine ecosystems in 2016. Canadian Technology Report Fisheries Aquatic Sciences 3225. Edited by PC Chandler, SA King, and J Boldt, Fisheries and Oceans Canada, Sidney BC.
Cooley S, Kite-Powell HL, and Doney SC. 2009. Ocean acidification’s potential to alter global marine ecosystem services. Oceanography, 22: 172–181.
Creighton C, Hobday AJ, Lockwood M, and Pecl GT. 2016. Adapting management of marine environments to a changing climate: a checklist to guide reform and assess progress. Ecosystems, 19: 187–219.
Crozier LG, Hendry AP, Lawson PW, Quinn TP, Mantua NJ, et al. 2008. Potential responses to climate change in organisms with complex life histories: evolution and plasticity in Pacific salmon. Evolutionary Applications, 1: 252–270.
Cummins P, and Haigh R. 2010. Ecosystem status and trends report for North Coast and Hecate Strait ecozone. DFO Canadian Science Advisory Secretariat Research Document 2010/045. 61 p.
Cummins PF, and Masson D. 2014. Climatic variability and trends in the surface waters of coastal British Columbia. Progress in Oceanography, 120: 279–290.
Cutter SL, Barnes L, Berry M, Burton C, Evans E, Tate E, et al. 2008. A place-based model for understanding community resilience to natural disasters. Global Environmental Change, 18: 598–606.
DiLorenzo E, and Mantua N. 2016. Multi-year persistence of the 2014/15 North Pacific marine heatwave. Natural Climate Change, 6: 1042–1047.
Dolan AH, and Walker IJ. 2006. Understanding vulnerability of coastal communities to climate change related risks. Journal of Coastal Research, 39: 1316–1323.
Doney SC, Ruckelshaus M, Emmett Duffy J, Barry JP, Chan F, English C, et al. 2012. Climate change impacts on marine ecosystems. Annual Review of Marine Science, 4: 11–37.
Ekstrom JA, Suatoni L, Cooley SR, Pendleton LH, Waldbusser GG, Cinner JE, et al. 2015. Vulnerability and adaptation of US shellfisheries to ocean acidification. Natural Climate Change, 5: 207–214.
Fleming SW, Whitfield PH, Fleming SW, and Whitfield PH. 2017. Spatiotemporal mapping of ENSO and PDO surface meteorological signals in British Columbia, Yukon, and southeast Alaska. Atmosphere-Ocean, 48: 121–131.
Foreman MGG, Callendar W, Masson D, Morrison J, Fine I, Callendar W, et al. 2014. A model simulation of future oceanic conditions along the British Columbia Continental Shelf. Atmosphere-Ocean, 48, 1–19.
Forum CP. 2019. Cascadia climate adaptation strategy [online]: Available from cascadiapartnerforum.org/.
Foundation TBSE. 2015. The State of British Columbia Coastal Communities in 2015. T. Buck Suzuki Foundation, New Westminster, BC.
Foundation TBSE. 2017. The State of British Columbia Coastal Communities in 2017. T. Buck Suzuki Foundation, New Westminster, BC.
Frölicher TL, Fischer EM, and Gruber N. 2018. Marine heatwaves under global warming. Nature, 560: 360–364.
Frusher S, van Putten I, Haward M, Hobday AJ, Holbrook NJ, Jennings S, et al. 2016. From physics to fish to folk: supporting coastal regional communities to understand their vulnerability to climate change in Australia. Fisheries Oceanography, 25: 19–28.
Galbraith M, and Young K. 2017. Zooplankton along the B.C. continental margin 2016. In State of the physical, biological and selected fishery resources of Pacific Canadian Marine Ecosystems in 2016. Canadian Technology Report Fisheries Aquatic Sciences 3225. Edited by PC Chandler, SA King, and J Boldt. Fisheries and Oceans Canada, Sidney BC.
Game ET, Lipsett-Moore G, Saxon E, Peterson N, and Sheppard S. 2011. Incorporating climate change adaptation into national conservation assessments. Global Change Biology, 17: 3150–3160.
Gattuso J-P, Magnan A, Billé R, Cheung WWL, Howes EL, Joos F, et al. 2015. Contrasting futures for ocean and society from different anthropogenic CO2 emissions scenarios. Science, 349.
Gazeau F, Parker LM, Comeau S, Gattuso JP, O’Connor W, Martin S, et al. 2013. Impacts of ocean acidification on marine shelled molluscs. Marine Biology, 160: 2207–2245.
Gerber LR, Del Mark Mancha-Cisneros M, O’Connor M, and Selig ER. 2014. Climate change impacts on connectivity in the ocean: implications for conservation. Ecosphere, 5: art33–18.
Gillespie GE, Norgard TC, Anderson ED, Haggarty DR, and Phillips AC. 2015. Distribution and biological characteristics of European Green Crab, Carcinus maenas, in British Columbia, 2006–2013. Canadian Technical Report of Fisheries and Aquatic Sciences, 3120.
Government B. 2010. Preparing for climate change: British Columbia’s adaptation strategy. BC Ministry of Environment, Victoria BC.
Government B. 2017. Adaptation & climate impacts [online]: Available from www2.gov.bc.ca/gov/content/environment/climate-change/adaptation.
Grant SCH, Michielsens CGJ, and MacDonald BL 2017. Fraser River Sockeye: abundance and productivity trends. In State of the physical, biological and selected fishery resources of Pacific Canadian Marine Ecosystems in 2016. Canadian Technology Report Fisheries Aquatic Sciences 3225. Edited by PC Chandler, SA King, and J Boldt.
Green JJ, Gill DA, and Kleiner AM. 2006. From vulnerability to resiliency: assessing impacts and responses to disaster. South Rural Sociology, 21: 89–99.
Guénette S, and Alder J. 2007. Lessons from marine protected areas and integrated ocean management initiatives in Canada. Coastal Management, 35: 51–78.
Haigh R, Ianson D, Holt CA, Neate HE, and Edwards AM. 2015. Effects of ocean acidification on temperate coastal marine ecosystems and fisheries in the northeast pacific. PLoS ONE, 10: e0117533.
Halpern BS, McLeod KL, Rosenberg AA, and Crowder LB. 2008. Managing for cumulative impacts in ecosystem-based management through ocean zoning. Ocean Coastal Management, 51: 203–211.
Hamann A, and Wang T. 2006. Potential effects of climate change on ecosystem and tree species distribution in British Columbia. Ecology, 87: 2773–2786.
Hansen J, Sato M, Hearty P, Ruedy R, Kelley M, Masson-Delmotte V, et al. 2016. Ice melt, sea level rise, and superstorms: evidence from paleoclimate data, climate modeling, and modern observations that 2°C global warming could be dangerous. Atmospheric Chemistry and Physics, Discussion, 16: 1–52.
Healey MC. 2009. Resilient salmon, resilient fisheries for British Columbia, Canada. Ecology and Society, 14(1): 2. [online]: Available from ecologyandsociety.org/vol14/iss1/art2/.
Healey M. 2011. The cumulative impacts of climate change on Fraser River sockeye salmon (Oncorhynchus nerka) and implications for management. Canadian Journal of Fisheries and Aquatic Sciences, 68: 718–737.
Henderson SB, Wan V, and Kosatsky T. 2013. Differences in heat-related mortality across four ecological regions with diverse urban, rural, and remote populations in British Columbia, Canada. Health Place, 23, 48–53.
Hino M, Field CB, and Mach KJ. 2017. Managed retreat as a response to natural hazard risk. Nature Climate Change, 7.
Hobday A, Maxwell S, Forgie J, and McDonald J. 2013. Dynamic ocean management: integrating scientific and technological capacity with law, policy, and management. Stanford Environment Law Journal, 33: 125–165.
Hobday AJ, Cochrane K, Downey-Breedt N, Howard J, Aswani S, Byfield V, et al. 2016. Planning adaptation to climate change in fast-warming marine regions with seafood-dependent coastal communities. Reviews in Fish Biology and Fisheries, 26: 249–264.
Hoegh-Guldberg O, and Bruno JF. 2010. The impact of climate change on the world’s marine ecosystems. Science, 328: 1523–1528.
Huang Y, Li F, Bai X, and Cui S. 2012. Comparing vulnerability of coastal communities to land use change: analytical framework and a case study in China. Environmental Science & Policy, 23: 133–143.
Hunter K, and Wade J. 2015. Pacific large aquatic basin climate change impacts, vulnerabilities and opportunities assessment—marine species and aquaculture. Fisheries and Oceans Canada.
Hunter KL, Ross ARS, Ianson D, Miller LA, Pearce CM, Christian JR, et al. 2015. Pacific Ocean Acidification Working Group. 2014/2015 Report Canadian Manuscript Report. Fisheries and Aquatic Sciences, 3070.
Ianson D, Allen S, Moore-maley B, Haigh R, and Johannessen S. 2016a. The vulnerability of the Strait of Georgia (Canada) to future hypoxia and ocean acidification. In EGU General Assembly 2016, Vienna Austria, 17–22 April 2016. 18, EGU2016-12687.
Ianson D, Allen SE, Moore-Maley BL, Johannessen SC, and Macdonald RW. 2016b. Vulnerability of a semienclosed estuarine sea to ocean acidification in contrast with hypoxia. Geophysical Research Letters, 43: 5793–5801.
IPCC. 2013. Climate change 2013: the physical science basis. Contribution of Working Group I to the Fifth Assessment Report of the Intergovernmental Panel on Climate Change 2013. Cambridge, UK and New York, New York.
IPCC. 2014a. Summary for policymakers. In Climate change 2014: impacts, adaptation, and vulnerability. Part A: global and sectoral aspects. Contribution of Working Group II to the Fifth Assessment Report of the Intergovernmental Panel on Climate Change. Edited by C.B. Field, V.R. Barros, D.J. Dokken, K.J. Mach, M.D. Mastrandrea, T.E. Bilir et al., Cambridge University Press, Cambridge, UK and NewYork, NewYork. pp. 1–32.
IPCC. 2014b. Climate change 2014: synthesis report. Contribution of Working Groups I, II and III to the Fifth Assessment Report of the Intergovernmental Panel on Climate Change. IPCC, Geneva, Switzerland.
Islam MM, Sallu S, Hubacek K, and Paavola J. 2014. Vulnerability of fishery-based livelihoods to the impacts of climate variability and change: insights from coastal Bangladesh. Regional Environmental Change, 14: 281–294.
Jackley J, Gardner L, Djunaedi AF, and Salomon AK. 2016. Ancient clam gardens, traditional management portfolios, and the resilience of coupled human-ocean systems. Ecology and Society, 21: 20.
Johnson CR, Banks SC, Barrett NS, Cazassus F, Dunstan PK, Edgar GJ, et al. 2011. Climate change cascades: shifts in oceanography, species’ ranges and subtidal marine community dynamics in eastern Tasmania. Journal of Experimental Marine Biology and Ecology, 400: 17–32.
Johnson JE, and Welch DJ. 2009. Marine fisheries management in a changing climate: a review of vulnerability and future options. Reviews in Fisheries Science, 18: 106–124.
Jones KR, Watson JEM, Possingham HP, and Klein CJ 2016. Incorporating climate change into spatial conservation prioritisation: a review. Biological Conservation, 194: 121–130.
Jones MC, and Cheung WWL. 2017. Using fuzzy logic to determine the vulnerability of marine species to climate change. Global Change Biology, 24: e719–e731.
Klein RJT, Midgley GF, Preston BL, Alam M, Berkhout FGH, Dow K, et al. 2014. Adaptation opportunities, constraints, and limits. In Climate change 2014: impacts, adaptation, and vulnerability. Part A: global and sectoral aspects. Contribution of Working Group II to the Fifth Assessment Report of the Intergovernmental Panel on Climate Change. Edited by CB Field, VR Barros, and DJ Dokken. pp. 899–943.
Lemmen DS, Warren FJ, James TS, and Mercer Clarke CSL. 2016. Canada’s marine coasts in a changing climate. Government of Canada, Ottawa, Ontario.
Lin BB, Capon T, Langston A, Taylor B, Wise R, Williams R, et al. 2017. Adaptation pathways in coastal case studies: lessons learned and future directions. Coastal Management, 45: 384–405.
Lynn K, Daigle J, Hoffman J, Lake F, Michelle N, Ranco D, et al. 2013. The impacts of climate change on tribal traditional foods. Climate Change, 120: 545–556.
Malakar Y. 2013. Increasing adaptive capacity: what is the role of local institutions? Risk, hazards cris. Public Policy, 3: 60–76.
Martins EG, Hinch SG, Patterson DA, Hague MJ, Cooke SJ, Miller KM, et al. 2011. Effects of river temperature and climate warming on stock-specific survival of adult migrating Fraser River sockeye salmon (Oncorhynchus nerka). Global Change Biology, 17: 99–114.
Maxwell SL, Venter O, Jones KR, and Watson JEM. 2015. Integrating human responses to climate change into conservation vulnerability assessments and adaptation planning. Annals of the New York Academy of Science, 1355: 1–20.
Miller K, Charles A, Barange M, Brander K, Gallucci VF, Gasalla MA, et al. 2010. Climate change, uncertainty, and resilient fisheries: institutional responses through integrative science. Progress in Oceanography, 87: 338–346.
Mills M, Weeks R, Pressey RL, Gleason MG, Eisma-Osorio RL, Lombard AT, et al. 2015. Real-world progress in overcoming the challenges of adaptive spatial planning in marine protected areas. Biological Conservation, 181: 54–63.
Mora C, Spirandelli D, Franklin EC, Lynham J, Kantar MB, Miles W, et al. 2018. Broad threat to humanity from cumulative climate hazards intensified by greenhouse gas emissions. Natural Climate Change, 8: 1062–1071.
Morrison WE, and Termini V. 2016. A review of potential approaches for managing marine fisheries in a changing climate. US Department of Commerce, NOAA. NOAA Technical Memorandum NMFS-OSF-6, 35 p.
Moser SC, and Ekstrom JA. 2010. A framework to diagnose barriers to climate change adaptation. Proceeding of the National Academy of Sciences of the United States of America, 107: 22026–22031.
Mote PW, and Salathé EP. 2010. Future climate in the Pacific Northwest. Climate Change, 102: 29–50.
Muñoz NJ, Farrell AP, Heath JW, and Neff BD. 2014. Adaptive potential of a Pacific salmon challenged by climate change. Natural Climate Change, 5: 163–166.
Murdock TQ, and Spittlehouse DL. 2011. Selecting and using climate change scenarios for British Columbia. In Pacific Climate Impacts Consortium, 39.
Murphy DWA. 2014. Theorizing climate change, (im)mobility and socio-ecological systems resilience in low-elevation coastal zones. Climate Development, 1–18.
Nerlich B, Koteyko N, and Brown B. 2010. Theory and language of climate change communication. WIREs Climate Change, 1: 97–110.
Ng AKY, Zhang H, Afenyo M, Becker A, Cahoon S, Chen S, et al. 2018. Port decision maker perceptions on the effectiveness of climate adaptation actions. Coastal Management, 46: 148–175.
Nyland D, and Nodelman JR. 2017. British Columbia. In Climate risks and adaptation practice for the Canadian transportation sector 2016. Edited by K Palko and DS Lemmen. Government of Canada, Ottawa, Ontario. pp. 66–103.
Okey TA, Alidina H, and Agbayani S. 2015. Mapping ecological vulnerability to recent climate change in Canada’s Pacific marine ecosystems. Ocean Coastal Management, 106: 35–48.
Okey TA, Alidina HM, Lo V, and Jessen S. 2014. Effects of climate change on Canada’s Pacific marine ecosystems: a summary of scientific knowledge. Reviews in Fish Biology and Fisheries, 24: 519–559.
Okey TA, Alidina HM, Lo V, Montenegro A, and Jessen S. 2012. Climate change impacts and vulnerabilities in Canada’s Pacific marine ecosystems. CPAWS BC and WWF-Canada, Vancouver, British Columbia.
Oppenheimer M, Little CM, and Cooke RM. 2016. Expert judgement and uncertainty quantification for climate change. Natural Climate Change, 6: 445–451.
Parkins JR, and MacKendrick NA. 2007. Assessing community vulnerability: a study of the mountain pine beetle outbreak in British Columbia, Canada. Global Environment Change, 17: 460–471.
Parmesan C. 2006. Ecological and evolutionary responses to recent climate change. Annual Review of Ecology, Evolution, and Systematics, 37: 637–669.
Parmesan C, and Yohe G. 2003. A globally coherent fingerprint of climate change impacts across natural systems. Nature, 421: 37–42.
PCIC. 2013a. Climate summary for: West Coast Region. Pacific Climate Impacts Consoritum, University of Victoria, Victoria BC.
PCIC. 2013b. Plan2Adapt: summary of climate change for British Columbia 2050s & 2080s. PCIC [online]: Available from pacificclimate.org/analysis-tools/plan2adapt.
Pelling M. 1997. What determines vulnerability to floods; a case study in Georgetown, Guyana. Environmental Urban, 9: 203–226.
Pelling M, and High C. 2005. Understanding adaptation: what can social capital offer assessments of adaptive capacity? Global Environment Change, 15: 308–319.
Pelling M, High C, Dearing J, and Smith D. 2008. Shadow spaces for social learning: a relational understanding of adaptive capacity to climate change within organisations. Environment and Planning A: Economy and Space, 40: 867–884.
Perry RI, Cury P, Brander K, Jennings S, Möllmann C, and Planque B. 2010. Sensitivity of marine systems to climate and fishing: concepts, issues and management responses. Journal of Marine System, 79: 427–435.
Perry RI, and Peña A. 2016. BC CDC. In Marine Biotoxin Workshop, North Vancouver, British Columbia, October 2016.
Pike RG, Spittlehouse DL, Bennett KE, Egginton VN, Tschaplinski PJ, Murdock TQ, et al. 2008. Climate change and watershed hydrology: Part I—recent and projected changes in British Columbia. Streamline Water Management Bullet, 11: 1–7.
Pinkerton E, Angel E, Ladell N, Williams P, Nicolson M, Thorkelson J, et al. 2014. Local and regional strategies for rebuilding fisheries management institutions in coastal British Columbia: what components of comanagement are most critical? Ecology and Society, 9.
Pinsky ML, Eikeset AM, McCauley DJ, Payne JL, and Sunday JM. 2019. Greater vulnerability to warming of marine versus terrestrial ectotherms. Nature, 569: 108–111.
Pinsky ML, and Fogarty M. 2012. Lagged social-ecological responses to climate and range shifts in fisheries. Climate Change, 115: 883–891.
Pinsky ML, and Mantua NJ. 2014. Emerging adaptation approaches for climate-ready fisheries management. Oceanography, 27: 146–159.
Pressey RL, Cabeza M, Watts ME, Cowling RM, and Wilson KA. 2007. Conservation planning in a changing world. Trends in Ecology & Evolution, 22: 583–592.
Rahman A, Worldfish S, Fisheries EC, and Sunny AR. 2017. A review on effect of global climate change on seaweed and seagrass. International Journal of Fisheries and Aquatic Studies, 5: 19–22.
Rand PS, Hinch SG, Morrison J, Foreman MGG, Macnutt MJ, Macdonald JS, et al. 2006. Effects of river discharge, temperature, and future climates on energetics and mortality of adult migrating Fraser River sockeye salmon. Transactions of the American Fisheries Society, 135: 655–667.
Reiblich J, Wedding LM, and Hartge EH. 2017. Enabling and limiting conditions of coastal adaptation: local governments, land uses, and legal challenges. Ocean and Coastal Law Journal, 22.
Reid MG, Hamilton C, Reid SK, Trousdale W, Hill C, Turner N, et al. 2014. Indigenous climate change adaptation planning using a values-focused approach: a case study with the Gitga’at nation. Journal of Ethnobiology, 34: 401–424.
Rodenhuis DR, Bennet KE, Werner AT, Murdock TQ, and Bronaugh D. 2006. Hydro-climatology and future climate impacts in British Columbia. In Pacific Climate Impacts Consortium, University of Victoria, Victoria, British Columbia. 132 p.
Sainsbury NC, Genner MJ, Saville GR, Pinnegar JK, O’Neill CK, Simpson SD, et al. 2018. Changing storminess and global capture fisheries. Nature Climatic Change, 8: 655–659.
Salafsky N, Boshoven J, Burivalova Z, Dubois NS, Gomez A, Johnson A, et al. 2019. Defining and using evidence in conservation practice. Conservation Science Practice, 1: e27.
Salathé EP, Mote PW, and Wiley MW. 2007. Review of scenario selection and downscaling methods for the assessment of climate change impacts on hydrology in the United States Pacific northwest. International Journal of Climatology, 27: 1611–1621.
Sales RFM. 2009. Vulnerability and adaptation of coastal communities to climate variability and sea-level rise: their implications for integrated coastal management in Cavite City, Philippines. Ocean & Coastal Management, 52: 395–404.
Savo V, Morton C, and Lepofsky D. 2017. Impacts of climate change for coastal fishers and implications for fisheries. Fish and Fisheries, 18: 877–889.
Semeniuk I. 2017. Sockeye salmon recommended for listing under Species at Risk Act. Globe Mail.
Sobie SR, and Murdock TQ. 2017. High-resolution statistical downscaling in Southwestern British Columbia. Journal of Applied Meteorology and Climatology, 56: 1625–1641.
Spittlehouse DL. 2008. Climate change, impacts, and adaptation scenarios: climate change and forest and range management in British Columbia. B.C. Technical Report 045.
Stratoudakis Y, Hilario A, Ribeiro C, Abecasis D, Goncalves EJ, Andrade F, et al. 2019. Environmental representativity in marine protected area networks over large and partly unexplored seascapes. Global Ecology and Conservation, 17: e00545.
Sweetman AK, Thurber AR, Smith CR, Levin LA, Mora C, Wei C, et al. 2017. Major impacts of climate change on deep-sea benthic ecosystems. Elementa: Science of the Anthropocene, 5: 4.
Teh LSL, Cheung WWL, and Sumaila UR. 2016. Scenarios for investigating the future of Canada’s oceans and marine fisheries under environmental and socioeconomic change. Regional Environmental Change, 17: 619–633.
Thomson RE, Bornhold BD, and Mazzotti S. 2008. An examination of the factors affecting relative and absolute sea level in coastal British Columbia. Canadian Technical Report of Hydrography and Ocean Sciences, 260: 49.
Turner NJ, and Clifton H. 2009. “It’s so different today”: climate change and indigenous lifeways in British Columbia, Canada. Global Environmental Change, 19: 180–190.
Vadeboncoeur N. 2016. Perspectives on Canada’s West Coast region. In Canada’s marine coasts in a changing climate. Edited by DS Lemmen, FJ Warren, TS James, and CSL Mercer Clarke. Government of Canada, Ottawa, Ontario. pp. 207–252.
Walker AIJ, Barrie JV, Walkerf IJ, and Barriej JV. 2006. Geomorphology and sea-level rise on one of Canada’s most sensitive coasts: Northeast Graham Island, British Columbia. Journal of Coastal Research, III: 220–226.
Walker B, Holling CS, Carpenter SR, and Kinzig A. 2004. Resilience, adaptability and transformability in social-ecological systems. Ecology and Society, 9: 5.
Walker IJ, and Sydneysmith R. 2008. Chapter 8: British Columbia. In From impacts to adaptation: Canada in a changing climate. Edited by DS Lemmen, FJ Warren, J Lacroix, and E Bush. Government of Canada, Ottawa, Ontario. pp. 329–386.
Warren FJ, and Lemmen DS. 2014a. Canada in a changing climate: sector perspectives on impacts and adaptation. Edited by FJ Warren and DS Lemmen. Government of Canada, Ottawa, ON. p. 286.
Warren FJ, and Lemmen DS. 2014b. Synthesis; in Canada in a changing climate: sector perspectives on impacts and adaptation. Edited by FJ Warren and DS Lemmen. Government of Canada, Ottawa, ON. pp. 1–18.
Weatherdon LV, Magnan AK, Rogers AD, Sumaila UR, and Cheung WWL. 2016a. Observed and projected impacts of climate change on marine fisheries, aquaculture, coastal tourism, and human health: an update. Frontiers in Marine Science, 3: 48.
Weatherdon LV, Ota Y, Jones MC, Close DA, and Cheung WWLL. 2016b. Projected scenarios for coastal first nations’ fisheries catch potential under climate change: management challenges and opportunities. PLoS ONE, 11: e0145285.
White I, and O’Hare P. 2014. From rhetoric to reality: which resilience, why resilience, and whose resilience in spatial planning? Environment and Planning C: Government and Policy, 32: 934–950.
Whitney FA. 2015. Anomalous winter winds decrease 2014 transition zone productivity in the NE Pacific. Geophysical Research Letters, 42: 428–431.
Whitney FA. 2017. Are central coast sockeye affected by glacial retreat? In State of the physical, biological and selected fishery resources of Pacific Canadian Marine Ecosystems in 2016. Edited by PC Chandler, SA King, and J Boldt. Fisheries and Oceans Canada, Sidney BC.
Whitney FA, Freeland HJ, and Robert M. 2007. Persistently declining oxygen levels in the interior waters of the eastern subarctic Pacific. Progress in Oceanography, 75: 179–199.
Withey P, Lantz VA, and Ochuodho TO. 2016. Economic costs and impacts of climate-induced sea-level rise and storm surge in Canadian coastal provinces: a CGE approach. Applied Economics, 48: 59–71.
Yates KL. 2014. View from the wheelhouse: perceptions on marine management from the fishing community and suggestions for improvement. Marine Policy, 48: 39–50.
Young T, Fuller EC, Provost MM, Coleman KE, St. Martin K, McCay BJ, et al. 2019. Adaptation strategies of coastal fishing communities as species shift poleward. ICES Journal of Marine Science, 76: 93–103.
Yumagulova L, and Vertinsky I. 2017. Climate change adaptation and flood management in metro Vancouver regional area: can an exercise in herding cats be successful? Journal of Sustainable Development of Energy, Water and Environment Systems, 5: 273–288.
Supplementary materials
Supplementary Material 1 (DOCX / 13.6 KB)
- Download
- 13.62 KB
Supplementary Material 2 (PDF / 856 KB)
- Download
- 856.40 KB
Supplementary Material 3 (DOCX / 16.8 KB)
- Download
- 16.86 KB
Information & Authors
Information
Published In
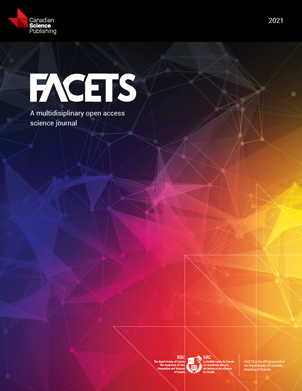
FACETS
Volume 5 • Number 1 • January 2020
Pages: 704 - 737
Editor: Steven J. Cooke
History
Received: 1 June 2019
Accepted: 17 June 2020
Version of record online: 10 September 2020
Copyright
© 2020 Whitney et al. This work is licensed under a Creative Commons Attribution 4.0 International License (CC BY 4.0), which permits unrestricted use, distribution, and reproduction in any medium, provided the original author(s) and source are credited.
Data Availability Statement
All relevant data are within the paper and Supplementary Material.
Key Words
Sections
Subjects
Authors
Author Contributions
CKW, TC, and RM conceived and designed the study.
CKW and TC performed the experiments/collected the data.
CKW and TC analyzed and interpreted the data.
NCB and RM contributed resources.
All drafted or revised the manuscript.
Competing Interests
The authors have declared that no competing interests exist.
Metrics & Citations
Metrics
Other Metrics
Citations
Cite As
Charlotte K. Whitney, Tugce Conger, Natalie C. Ban, and Romney McPhie. 2020. Synthesizing and communicating climate change impacts to inform coastal adaptation planning. FACETS.
5(1): 704-737. https://doi.org/10.1139/facets-2019-0027
Export Citations
If you have the appropriate software installed, you can download article citation data to the citation manager of your choice. Simply select your manager software from the list below and click Download.
Cited by
1. Integration of satellite image–derived temperature and water depth for assessing fish habitability in dam controlled flood plain wetland
2. Climate-Related Vulnerability and Risk Assessment of Main Ocean Uses: An Overview
3. A climate-resilient marine conservation network for Canada
4. Optimizing power system operations for extreme weather events: A review
5. Indigenous knowledge of key ecological processes confers resilience to a small‐scale kelp fishery
6. Stakeholder perceptions on actions for marine fisheries adaptation to climate change