Heliocaminiform structures: plant organs that function as microgreenhouses
Abstract
Graphical Abstract

Introduction and etymology
Major taxon | Family | E.g., genus & species | Temperatures within hollow structures | Notes & references | |
---|---|---|---|---|---|
1 | Magnoliophyta (Angiospermae) | Many | Many | Hollow floral buds that may open with reflexed petals or expose reproductive structures in anthesis in zygomorphic, stereomorphic, urceolate, or actinomorphic flowers are probably heliocaminiform prior to full anthesis. Most trap and semi-trap blossoms are hollow prior to anthesis. There are various species that close when shaded but reopen when re-insolated (see rows 11 and 23). | See van der Kooi et al. (2019), van Doorn and van Meeteren (2003), van Doorn and Kamdee (2014), van Doorn and Kamdee (2014). |
2 | Amaryllidacaea | Many species with hollow stems. Observed temperatures within the stems of Narcissus papyraceus 5.80 ± 0.70 °C in the sun, and 1.15 ± 0.78 °C in shade, within a study greenhouse. Narcicuss sp. (daffodil form) grown on University of Guelph campus gardens had stem interiors up to 6.6 °C warmer than ambient air in sun and ranged from to + 2.7 °C to -0.2 °C in shade. | Kevan & Coates unpublished from University of Guelph Greenhouse, Winter 2019. | ||
The epicalyx bract of some species may form a heliocaminiform structure. | No records have been discovered. | ||||
3 | Anacardiaceae | Pistacia vera | Hollow galls may buffer extreme heat from adverse effects on aphids living within. | Martinez (2009). | |
4 | Apiaceae | Many species | Hollow stems are characteristic of many species. We have made intrastem temperature measurements in cow parsley (Heracleum maximum). In sunshine we found temperatures up to about 5 °C in the stems. | Kevan and team 2018–2019 casual spot checks in Avoca, QC and McMillans Corners, ON. | |
Anisotome latifolia | Large, divided, smooth leaves forming enclosed cavity beneath and becoming warmer than ambient temperatures on sub-Antarctic Campbell Is. | Little et al. (2016). | |||
5 | Apocynaceae | Asclepias syriaca | Hollow fruits (follicles) attain temperatures excesses of over 13 °C in sunshine but the effect is abolished at night and under cloudy conditions. The balloon plant milkweed (Asclepias (a.k.a Gomphocarpus) physocarpa) produces inflated, papery follicles when in fruit. | Kevan & Coates unpublished from Guelph & Cambridge, ON from summers 2019-2021. | |
6 | Araliaceae | Stibocarpus polaris | Large, hairy leaves forming enclosed cavity beneath and becoming warmer than ambient temperatures on sub-Antarctic Campbell Is. | Little et al. (2016). | |
7 | Arecaceae | Many species | The maturing inflorescences of many palms are held within hollow spadices (bracts) which split at anthesis. | No records found on thermal relations. | |
8 | Asphodelaceaea | Bulbinella rossii | Large, smooth leaves form enclosed cavity beneath and those, and the leaves, become warmer than ambient temperatures on sub-Antarctic Campbell Is. | Little et al. (2016). | |
9 | Asteraceae | Many species | Hollow stems and scapes are known in various species. Temperatures in hollow stemmed Cirsium setosum may reach 2.66 ± 0.631 °C above ambient. Within the hollow peduncles of Taraxacum officinale temperatures may reach 6.07 ± 1.81 °C in sunshine: the effects are abolished at night or under cloudy conditions. In Ptarmica salicifolia, internal temperature of stems of 4 study plants reached a maximum of 4.65 ± 0.59 °C above ambient temperature. | Kevan et al. (2019) for C. setosum, T. officinale, P. salicifolia in Magadan oblast, Russia. Results for T. officinale in Guelph, ON remain unpublished from 2019-2022 Kevan and his team. | |
In stems of Gerbera jamesonii temperature excesses during the day in greenhouse grown plants can reach 4 °C. G. jamesonii varieties have inconsistent hollowness, so the temperature range an individual may experience may vary even within a given cultivar and stem. | Kevan and Coates, (2020), COHA Connections. Understanding how temperatures within plants affect their growth. https://cohaconnections.ca/understanding-how-temperatures-within-plants-affect-their-growth/ | ||||
Temperatures with the hollow stems of Rudbeckia hirta (also variable as to hollowness) in sunshine were about 2 – 4 °C above ambient | Kevan, Smith and Humphrey unpublished data from spot checks from roadside plants near Bell. Falls and Harrington, QC in 2018 and 2019. | ||||
The hollow stems of Zinnia spp. reached up to 2 °C warmer than ambient air. | Zinnias grown on University of Guelph campus, Larson & Coates, unpublished from 2019. | ||||
The bracts around the inflorescences of Saussurea velutina have been shown to protect the reproductive structures from fluctuating weather conditions in high mountains. | Yang and Sun (2009). | ||||
Pleurophyllum spp | Hirsute to downy large leaves form enclosed cavity beneath and that, and the leaves, become warmer than ambient temperatures on sub-Antarctic Campbell Is. | Little et al. (2016). | |||
10 | Balsaminaceae | Impatiens glandulifera and I.capensis | In Ontario, the hollow, septate stems of I. glandulifera reached up to 8 °C warmer than ambient air under direct sunlight. Under partially cloudy conditions, the effect was reduced to 4 °C. In Perthshire shady woodlands temperatures within the stems reached 2 – 3 °C. | Coates unpublished for ON records from 2021, Kevan unpublished Scottish records from 2019 for I. glandulifera. | |
Within the hollow stems of I. capensis temperature excesses of 2 - 4 °C were recorded in sunshine but were only 1 – 2 °C under natural semi-shade. | Kevan unpublished data from Grenville-sur-la-Rouge, QC from summer 2019. | ||||
11 | Brassicacaeae | Lesquerella spp. | Hollow siliques and in L. arctica, the flowers close epinastically when the sun is obscured by cloud. | No records found on thermal relations; Kevan (1970). | |
Caulanthus spp. | Caulanthus inflatus and C. hallii | Both desert-adapted species have specialized inflated stems. No studies on temperature regimes within have been made. https://www.youtube.com/watch?v=CVpjLRNo1jc | |||
12 | Bromeliaceae | Puya spp. | Comparisons of temperature excesses between differentially pubescent species at different altitudes and between experimentally denuded plants indicate the importance of the pubescence. | Miller (1986) | |
13 | Campanulaceae | Platycodon grandiflorus | Balloon flowers become inflated as the floral buds approach anthesis. Various species of Campanula produce inflated floral buds. | No records found on thermal relations. | |
14 | Cannabaceae | Cannabis sativa | Some cultivars have hollow stems. Preliminary observations noted 3.5 °C temperature excess within the stems in sunshine at an air temperature of 27 °C. | Kevan et al. unpublished from near Greenfield, ON 2020. | |
15 | Caryophyllaceae | Silene spp. | Hollow syncalyces of S. uralensis, and S. sorensensis in the High Arctic may attain temperature excesses within of up to 2.5 °C, 6.2 °C respectively under sunny conditions but the effect is abolished when it is cloudy. | Kevan (1970, 1989, 2019). | |
16 | Cleomaceae | Isomeris arborea | Hollow fruits. | No records found on thermal relations. | |
17 | Cucurbitacea | Cucurbita spp. | Hollow peduncles and petioles of C. pepo in flower attained temperature excesses up to 9 °C in sunshine but the effect is abolished under cloud and at night. Highest temperature differences occurred during 10AM -2PM. | Coates unpublished from 2020-2021, Cambridge, ON. | |
Echinocystis lobata. Possibly Ecballium elaterium. | Hollow fruits of E. lobata in sunshine but the effect is abolished at night and under cloudy conditions. On a sunny day (with incident light of 1.165 x 105 LUX), we recorded temperatures within fruits at 8.29 ± 0.48 °C warmer than ambient air. On cloudy days (e.g., with incident light 1.4 x 104 LUX) that average was reduced to 0.09 ± 0.19 °C. | Kevan and Coates unpublished data from E. lobata in summers 2019-2021 at Washington Creek, ON. | |||
E. elaterium fruits may attain hyperbaric pressure within as part of building up temperature excess within as part of the explosive means of seed dispersal. | No records found on thermal relations. | ||||
18 | Cyperaceae | Carex spp | The inflorescences are contained in involucral bracts until anthesis. | No records found on thermal relations. | |
19 | Ericaceae | Vaccinium (Oxycoccus) spp. | Hollow fruit. | No records found on thermal relations. | |
20 | Euphorbiaceae | Hevea brasiliensis | Explosively dehiscing 3-lobed, 3-seeded capsule (somewhat hollow). | No records found on thermal relations. | |
Ricinus communis | Hollow stemmed. | No records found on thermal relations. | |||
21 | Fabaceae | Peas and beans | Hollow pods are characteristic of numerous species and cultivars of legumes. Notable are Baptisia spp., Sutherlandia frutescens (balloon pea), and Pisum sativum (garden pea). | No records found on thermal relations. | |
22 | Fagaceae | Quercus spp. | Hollow galls. | No records found on thermal relations. | |
23 | Gentianaceae | Gentiana spp., Gentianella spp. | Hollow flowers as in bottle gentians. Gentiana spp.) and in Gentianella amarella produce flowers that close when clouds obscure the sun. | Unpublished field observations by Kevan et al. (2018) for Gentiana spp. from alpine Colorado (G. algida) and G. clausa in Ontario and for Gentianella amarella from Churchill, MB. We have found no records of intrafloral temperatures. | |
24 | Iridacea | Crocus spp. | McKee and Richards (1998) report that illuminated flowers attain internal temperatures of about 3 °C and than purple and white flowers warm more than do yellow ones. | McKee and Richards (1998). | |
Iris spp. | Hollow stems, flower, and fruits. | No records found on thermal relations. | |||
Oncocyclus spp. | Hollow flowers attain temperature excesses of about 2.5 °C in morning sunshine. The flowers are used as sleeping places by pollinating bees. | Sapir et al. (2006). | |||
25 | Lamiaceae | Eriophyton wallichii | Extensive and dense pubescence on leaves of this Himalayan plant dampen temperature fluctuations. | Peng et al. (2015). | |
26 | Liliaceae | Many species have hollow stems. The flowers of Tulipa spp. close at night and when the sun is obscured by clouds. | No records found on thermal relations. | ||
27 | Malvaceae | Abelmoschus spp. | Fruits are hollow capsules. | No records found on thermal relations. | |
28 | Moraceae | Ficus spp. | Ripening fruits are thick-walled and hollow. Comparative studies of 11 species in Panama indicate temperature excesses of 2 – 3 °C of fruit from about 5 – 50 mm in diameter in which insolational heat gains were naturally reduced by evapotranspiration, especially in larger figs, to below the lethal levels for the inquiline pollinating wasp larvae. | Patiño et al. (1994) | |
29 | Orchidaceae | Many orchid flowers present enclosed parts, notably the slipper orchids (Cypripedioideae). Serapias vomeraceae attains temperature excesses of about 3 °C above ambient air temperature, a phenomenon that is associated with early morning pollinating activity of bees that sleep the night in the flowers. | We know of no records of temperature regimes in slipper orchid flower, see Dafni et al. (1981)for S. vomeracea. | ||
30 | Orobanchaceae | Pedicularis spp. | Hollow flowers of P. langsdorfii and P. capitata develop temperature excesses up to about 6 °C in sunshine, an effect that is abolished in cloudy weather. | Kevan (1970, 1989, 2019a, 2019b). | |
Hollow stems may attain internal temperatures several degrees warmer than the ambient air. Pubescence of the plants contributes to the effects (see also row 3). | Meier (1995). | ||||
Rhinanthus spp. | Yellow rattle owes its common name to the hollow capsules in which the seeds develop and rattle around loose until released. Flowers and capsules are probably both heliocaminiform structures. | We know of no records of temperature regimes in this genus. | |||
31 | Plantaginaceae | Antirrhinum spp. & Linaria spp. | Common name “snapdragon” indicates this flower opens and closes when squeezed laterally. Differences in pigmentation, as in cultivars of Antirrhinum, may alter the amount of heat trapped within the corolla but our preliminary records have not provided clues as to the effects of colouration. Temperature excesses within the flowers range up to 4.5 °C in sunshine midday in the University of Guelph Bovey greenhouse, but were abolished in shade and cloudy weather. | Photograph of research site appears in Kevan, Coates (2020). | |
32 | Poacaea | Many species | Hollow culms, as in Avena sativa from Magadan, Russia attain temperature excesses within of about 4 C in sunshine and for Phragmites australis temperature excesses with culms of 6.3 °C. The thermal regimes in the boot (reproductive structures enclosed in the developing flag leaves (bracts) and husks as in maize (Zea mays) seem not to have been investigated but we recorded temperature excesses of about 1-2 °C in ears on a production field margin in 2023. | Kevan et al. (2019) for A. staiva; Coates unpublished, ON., for P. australis in summer 2021. Kevan & Coates, unpublished data from Skunks Misery, ON, August 2023. | |
33 | Polygonaceae | Rheum spp. | Several high-altitude species produce overlapping translucent, achlorophyllous bracts that act as microgreenhouses and protect the inflorescence from UV light. The stems, as in many Rheum spp., are hollow. | Bojian and Grabovskaya-Borodina, (2003); Omori and Ohba (1996); Omori et al. (2000); Iwashina et al. (2004); Tsukaya 2002; Song et al. (2013, ). | |
Reynoutria japonica | Japanese knotweed has septate stems, with large hollow cavities that may reach to 4.6 °C warmer in the sun during the day, and down to -3.6 °C below ambient temperature at night. | Kevan & Coates, unpublished data from Cambridge, ON (Riverside Park) 2021. | |||
Rumex spp. | Several species have hollow flowering/fruiting stems. For R. patientia internal statistically significant temperatures excesses of 3.99 ± 0.84 (t = 77.58 p < 0.001) and 4.45 ± 0.74 C (t = 19.76, p < 0.001) were measured in plants growing in sunshine in Belgrade, Serbia | Kevan & Coates unpublished from 2020. | |||
Eriogonum inflatum | Has specialized inflated stem parts below the inflorescence. The gas composition within has been proposed to ameliorate photosynthetic activity. | Osmond et al. (1987) | |||
34 | Ranunculaceae | Nigella damascena. | Hollow fruits studied on garden plants had mean temperature excess of 5.5 ± 0.4 °C in sunshine Mature pods split and so disperse their seeds: high temperatures may contribute to the drying of ovary wall and development of seeds. | Kevan & Coates unpublished from Cambridge, ON summers 2020-2021 | |
Thalictrum pubescens | Hollow stems can attain internal temperatures of about 4 - 5 in sunshine. | Kevan and team unpublished from Grenville-sur-la-Rouge, QC, 2–8 August 2019. | |||
Delphinium barbeyi | Hollow stems may be reach 30–37 C while ambient temperatures are 13–16 C. The effect is abolished at night and in shade. | Billings and Godfrey (1967) from high altitudes (subalpine at 3100 m) in the Medicine Bow Mts, WY. They invoke the greenhouse effect. | |||
35 | Salicaceae | Salix spp. | The pubescence of the catkins (aments) probably acts to result in microgreenhouse effects which differ between the sexes. | Kevan (1990). See main text under "pubescence" for other references. | |
36 | Sapindaceae | Koelreuteria | Hollow, inflated capsular fruit. | No records found on thermal relations. | |
37 | Solanaceae | Physalis spp. | Hollow syncalyces of P. heterophylla and P. virginiana may attain temperature excess of up to 10.0 °C in sunshine but the phenomenon is abolished under cloudy conditions. In experimental greenhouse trials at the University of Guelph, the temperatures within the hollow syncalyxes of P. peruviana reached 6.6 °C warmer than the surrounding air temperature in sunny weather. At night, the temperatures within the same hollow syncalyxes were about 1-2 °C cooler than the surround air. | Kevan et al. unpublished from Cambridge, ON, Guelph summer & fall 2018 & 2019. Li et al. (2019) recorded somewhat similar conditions in P. floridana in their detailed study. | |
Capsicum spp. | Hollow fruits of C. annuum may attain temperatures excesses of up to about 4 – 6 °C in greenhouse conditions even without insolation. They may be generating heat metabolically but this effect has yet to be investigated. | Coates and Kevan (2020). | |||
38 | Equisetales | Equisetum spp. | Equisetum spp. have hollow stems which have been investigated for biomechanical properties. | Niklas (1992); Niklas and Spatz (2012). | |
Preliminary checks on the internal thermal regimes in fertile shoots of sporophytes of E. arvense indicate temperature excesses of only 1 - 2 °C in sunshine but -1 °C in roadside shade. Similar results were found for E. hylemale involving more detailed preliminary study, no statistical differences were found. | Kevan unpublished from Grenville-sur-la-Rouge, QC summer 2019 from roadside. E. arvense. Preliminary results by Coates & Kevan for E. hylemale at Skunk's Misery, ON 10 August, 2022. | ||||
39 | Phaeophyta | Phaeophycaceae | Pneumatocysts in Fucus, Macrocsytis, Nereocystis, Sargassum are well-known and usually explained as aiding in buoyancy of the photosynthetic lamina. As far as we are aware, temperatures have not been measured in pneumatocysts until now but ambient temperature regimes affect the gas (notably CO2) within. | See King (2001); Supratya et al. (2020); Liggan and Marton (2020). Temperatures within the pneumatocysts of Fucus vesiculosus and Nereocystis luetkeana were recorded at ca. 4 °C in sunshine on the coast of Washington in July, 2023 (Fig. 5). | |
40 | Bryophyta | Sphagnum spp. | Sporophytes explosively release spores when insolated. Temperatures within remain unstudied. | Kimmerer (1994) | |
41 | Lichens | Cladonia spp. and Cladina spp. | Hollow podetia. Preliminary measurements from a Laurentian forest site at Bell Falls, Avoca, QC, Canada indicate up to ca. 4 C temperature excess within in sunshine in Cladonia cristatella. | Kevan, unpublished casual observations, summer 2021; Tikhmenev, E, Personal communication, 2021 | |
42 | Fungi | Catherellus and Craterellus, Morchella spp., and many basidiocarp fungi. | Hollow stipe or pileus. Most fungi known to have hollow structures grown in shaded environments so microgreenhouse effects from insolation probably do not apply. The structure of the Nidulariaceae suggests that thermal relations and possibly insolation may play a role in maturation. | No records found on thermal relations. |
Note: Many of the values presented for internal temperatures are based on unpublished spot-checks as explained in Materials and Methods in various localities over the years 2018–2023. The values represent the approximate ranges of values one might expect when making more detailed studies.
Results
Discussion
Plant pubescence

Flowers and Inflorescences

Calyces
Bracts
Fruits


Galls
Stems


Algae

Bryophytes
Fungi
Lichens
Conclusion
Process and product
Phenology, climate change, and phenological mismatches
Acknowledgements
References
Information & Authors
Information
Published In
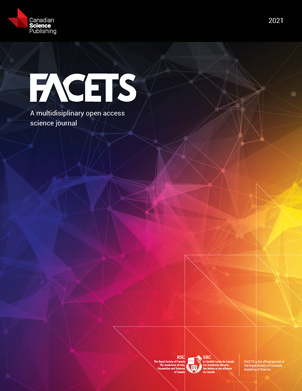
History
Copyright
Data Availability Statement
Key Words
Sections
Subjects
Authors
Author Contributions
Competing Interests
Metrics & Citations
Metrics
Other Metrics
Citations
Cite As
Export Citations
If you have the appropriate software installed, you can download article citation data to the citation manager of your choice. Simply select your manager software from the list below and click Download.
There are no citations for this item