Integrative adaptive management to address interactions between biological invasions and protected area connectivity: a Canadian perspective
Abstract
Introduction
The threat of invasive species in protected areas

Confounding factors for protected area network planning
Anthropogenic contexts for protected area connectivity
Variation in edge effects across protected areas and corridor linkages

An integrative adaptive management framework addressing interactions between biological invasions and protected area connectivity
Assessing ecological conditions
Inter-organizational planning
Risk assessment and management prioritization
Scheduling and budgeting considerations
Partnerships and outreach
Concluding remarks
Acknowledgements
References
Information & Authors
Information
Published In
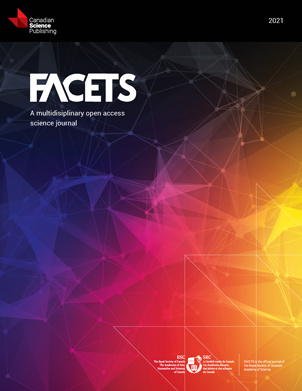
History
Copyright
Data Availability Statement
Key Words
Sections
Subjects
Authors
Author Contributions
Competing Interests
Funding Information
Metrics & Citations
Metrics
Other Metrics
Citations
Cite As
Export Citations
If you have the appropriate software installed, you can download article citation data to the citation manager of your choice. Simply select your manager software from the list below and click Download.
There are no citations for this item