A systematic conservation planning approach to maintaining ecosystem service provision in working landscapes
Abstract
Balancing human well-being with the maintenance of ecosystem services (ES) for future generations has become one of the central sustainability challenges of the 21st century. In working landscapes, past and ongoing production-centered objectives have resulted in the conversion of ecosystems into simple land-use types, which has also altered the provision of most ES. These inevitable trade-offs between the efficient production of individual provisioning ES and the maintenance of regulating and cultural ES call for the development of a land-use strategy based on the multifunctional use of the landscape. Due to the heterogeneous nature of working landscapes, both protection and restoration actions are needed to improve their multifunctionality. Systematic conservation planning (SCP) offers a decision support framework that can support landscape multifunctionality by indicating where ES management efforts should be implemented. We describe an approach that we developed to include ES provision protection and restoration objectives in SCP with the goal of providing ongoing benefits to society. We explain the general framework of this approach and discuss concepts, challenges, innovations, and prospects for the further development of a comprehensive decision support tool. We illustrate our approach with two case studies implemented in the pan-Canadian project ResNet.
Introduction
Ecosystems provide life-support services that underpin human well-being (Summers et al. 2012; Myers et al. 2013; Villamagna and Giesecke 2014). Ecosystem services (ES)—the benefits that the natural environment provides to people—greatly influence the quality of life in human societies by providing material (food, water, raw materials) and fulfilling nonmaterial needs (Millennium Ecosystem Assessment 2005; Summers et al. 2012; Myers et al. 2013; Villamagna and Giesecke 2014). Since the beginning of the 20th century, society has come to increasingly value the cheap and efficient production of individual provisioning ES (e.g., food, timber, etc.), but at the cost of extensively altering natural ecosystems. Although these changes have been associated with improvements in some health indices globally over the last 60 years, they have also profoundly altered the flow of regulating, supporting, and cultural services from nature to human societies (Raudsepp-Hearne et al. 2010; Duraiappah 2011; Summers et al. 2012; Myers et al. 2013). As the world’s population is forecast to reach nearly 10 billion by 2050 (United Nations 2019), it is uncertain whether altered landscapes will sustain the levels of needs that will accompany such rapid population growth (Myers et al. 2013). Balancing human well-being and prosperity with the maintenance of ES has, therefore, become one of the central sustainability challenges of the 21st century (Fenichel and Zhao 2015; Guerry et al. 2015).
In working landscapes—land actively used for the production of resources such as food, timber, and energy—trade-offs between ES are inevitable, especially between provisioning services and those from the three other categories (Cimon-Morin et al. 2013; Bahadur et al. 2020). But at the same time, maintaining a diversity of ES is essential since the amount and quality of ES supply, notably provisioning ones, is often determined by other ES embedded in the landscape (Schröter et al. 2018). Although investing in technical innovations may help society reach sustainability by developing substitutes for services provided by nature or improving the capacity of ecosystems to provide services (Honey-Rosés et al. 2014), several ES, such as most regulating and cultural ones, cannot easily be replaced by manufactured goods or technological alternatives (Ang and Van Passel 2012; Fenichel and Zhao 2015; Drupp 2018; Gollier 2019). An increasing number of studies suggest that implementing land-use strategies that rely on the multifunctional use of landscapes should provide ecological, socio-cultural, and economic benefits that go beyond the immediate financial gain obtained from fully converted working landscapes such as intensively managed farmland (Mander et al. 2007; de Groot et al. 2010; Law et al. 2017; Juutinen et al. 2019). By simultaneously supporting habitat maintenance and productivity as well as regulatory, social, and economic functions, multifunctional landscapes are expected to provide increased and diversified ES to society (Mander et al. 2007). A key step towards landscape sustainability (see Box 1 for a glossary of bolded concepts) involves developing innovative spatial planning approaches that can be used to more optimally sustain the desired level of ES over time (Seppelt et al. 2013; Albert et al. 2014; Bennett et al. 2015).
Box 1. Concepts and definitions.
Beneficiary: The people whose well-being is influenced by ecosystem services (Daw et al. 2011, Bagstad et al. 2014). How vulnerable beneficiaries are to ES losses depends mainly on the extent to which a marginal decline in ecosystem services would have an immediate impact on their well-being and livelihood (Myers et al. 2013). The preferences and vulnerability of beneficiaries vary and so does the extent to which they value ES benefits (Watson et al. 2019).
Benefit: Direct or indirect gains that people receive from ecosystem services measured in aspects of human well-being (Villamagna et al. 2013). Benefits to people arise from the interaction between capacity and demand for ES (i.e., from ES provision).
Benefit-centered conservation: Conservation planning focused on maximizing human benefits from ecosystem services and thus yielding the greatest returns for human well-being.
Benefit gap: A spatially explicit metric of locations where, for any particular ES, there is a lack of supply or flow connection when there is demand (adapted from Chaplin-Kramer et al. 2019). From a conservation planning perspective, two types of benefit gaps can be distinguished, the first occurs when there is no supply or no flow connection, such that demand is completely unsupplied (type 1). The second type involves undersupplied ES in which the capacity of an ecosystem to fulfill demand is undermined due to its ecological alteration (type 2).
Benefiting area: A spatial unit in which ecosystem services are needed, used, or consumed (Syrbe and Walz 2012, Serna-Chavez et al. 2014). A benefiting area may or may not be supplied by a providing area.
Connecting area: The intervening space between noncontiguous providing and benefiting areas that influence process variables (Syrbe and Walz 2012, Serna-Chavez et al. 2014). The ecological alteration of connecting areas can disrupt ES flow and prevent a providing area from connecting with its benefiting areas.
Demand: ES demand can be defined in several ways (see Wolff et al. 2015). At the planning region scale, demand represents the sum of the benefits currently used that are obtained in or from the area. Assessing ES demand quantitatively can provide information on the quantity of supply that needs to be protected (to set ES conservation targets; Luck et al. 2012). This can ensure that beneficiaries’ needs are met, while preventing the expenditure of unnecessary conservation resources on protecting a surplus of ES capacity. From a spatial prioritization perspective, however, ES demand can also be defined as a measure of the needs of beneficiaries for a given ES, expressed spatially and quantitatively (Villamagna et al. 2013; Cimon-Morin et al. 2014; Wolff et al. 2015; Cimon-Morin et al. 2018). In other words, this second type of demand represents the probability that a specific providing area within the planning region would be used or needed for the delivery of a particular service to a given set of beneficiaries (Cimon-Morin et al. 2014). It is this second type of demand that is integrated in the spatial planning process as a conservation feature, along with ES capacity.
ES capacity (also referred to as ES supply or ES biophysical supply): Ecosystem biophysical conditions and processes that contribute to the potential delivery of a particular ecosystem service (Bagstad et al. 2013, Villamagna et al. 2013). It represents the modeled intrinsic capability of ecosystems to supply a given ES (Mitchell et al. 2021).
ES flow: ES flow corresponds to the interactions and processes that spatially and temporally connect providing areas to benefiting areas (Serna-Chavez et al. 2014, Schröter et al. 2018). While the term ES flow is sometimes used in the literature to refer to actual service delivery (i.e., provision; Villamagna et al. 2013), here we rather define it as a means toward service provision that describes where the benefits are received (Serna-Chavez et al. 2014). A typical ES flow pathway can be characterized by (i) its spatial scale (ranging from in situ to global), (ii) its directions, (iii) its type (biophysical flows of traded goods, flows mediated by migratory or moving species, passive biophysical flows such as flood control along waterways, and information flows), (iv) its temporal dynamics, and (v) the presence of both biophysical barriers restricting and anthropogenic processes facilitating expansion of its spatial extent (Bagstad et al. 2014; Serna-Chavez et al. 2014; Schröter et al. 2018; Boesing et al. 2020; Kleemann et al. 2020).
ES provision: The actual service delivered to society. The concept of ES provision implies that a real contribution to human well-being occurs not only when ES are supplied, but also when the benefits are accessible and when a minimum amount of demand is fulfilled (Cimon-Morin et al. 2018; Mitchell et al. 2021). Thus, for ES provision to arise, ES capacity must be actually used, consumed or enjoyed by society. The mapping process leading to ES provision presented in this study assumes that each actual providing area currently delivers at least a minimal amount of ES benefits to society.
Flow area (also referred to as benefit zone): A spatial unit in which the ES benefits from a providing area are accessible or can be experienced by beneficiaries (see Fig. 2b; Villamagna et al. 2013; Serna-Chavez et al. 2014), but this does not imply that benefits are in demand. The capacity of providing areas and the flow pathway (see ES flows above) define the flow area (Villamagna et al. 2013).
Landscape sustainability: The capacity of a landscape to consistently provide long-term, landscape-specific ecosystem services essential for maintaining and improving human well-being (Wu 2013).
Providing area: A spatial unit from which ecosystem services are sourced (Serna-Chavez et al. 2014). Providing areas are mapped according to ES capacity, while actual providing areas are a subset of providing areas that currently fulfill a least a minimal amount of demand (Mitchell et al. 2021).
Provider-centered conservation: Conservation planning focused on the maintenance of service providers (without necessarily considering benefits). In certain landscapes, benefit-centered conservation may not be sufficient to ensure that the needs (habitats, metapopulation dynamics) of service providers are fulfilled in the long term, possibly leading to provider abundance declines and ultimately to benefit sustainability issues in the long term.
Serviceshed: An area, or a collection of actual providing areas, that provides a particular ES to a particular beneficiary or group of people (Mandle et al. 2015, Tallis et al. 2015). According to Tallis et al. (2015) a serviceshed can be spatially defined by both where the service is produced (i.e., the area that supports ES capacity) and who receives the benefits, which implies that beneficiaries must have physical and institutional access to the benefits (Mandle et al. 2015). A serviceshed delineation is based on the beneficiaries’ perspective. For example, the serviceshed for pollination services is the area around a benefiting area (e.g., pollination-dependent crop field) within the flight distance(s) of pollinator species in relation to habitats (for other examples see Tallis et al. 2015).
Service provider: Key components of nature (i.e., level of organization) that are responsible for the production of discrete units of a specific ES (e.g., population, functional group, community, structural component, habitat type, etc.; Luck et al. 2009; Kontogianni et al. 2010; Ziter 2016). The service provider generally consists of specifically defined biophysical units (e.g., the area of a particular vegetation type required to regulate water flow; Kontogianni et al. 2010). Although it is generally assumed that providers can be delineated only if the human need for an ecosystem process has been identified and if the rate of service delivery meets beneficiary needs (Kontogianni et al. 2010), we argue here that, for a comprehensive ES conservation approach, service providers also need to be protected to ensure the persistence of service provision (see provider-centered conservation above).
Service provider: Combines the concepts “ecosystem services provider” and “service providing unit” (Luck et al. 2009).
Systematic conservation planning (SCP): A structured and multi-step decision support process to identify and implement conservation areas and devise management policy, with feedback, revision, and reiteration as needed (Margules and Sarkar 2007; Pressey and Bottrill 2008; Sarkar and Illoldi-Rangel 2010; McIntosh et al. 2016). SCP is a comprehensive approach to conservation and involves 11 stages including scoping the planning process, holding participatory planning and stakeholder consultations, selecting new conservation areas using decision support software, implementing collaborative strategies, and monitoring the actions implemented (Pressey and Bottrill 2008; McIntosh et al. 2016). SCP offers tools to optimally design and expand reserves by identifying areas with the highest complementarity. Under a SCP framework, two target-based problems can generally be solved, namely minimum-set and maximum-coverage planning. Minimum-set planning tries to satisfy given targets of multiple ecological features at minimum cost, while maximum-coverage planning tries to maximize the level of representation of as many features as possible within the available budget when resources are not sufficient to meet all targets.
Systematic conservation planning (SCP; Margules and Sarkar 2007) offers a decision support framework to guide practitioners in determining cost-efficient solutions to achieve landscape sustainability. Its comparative advantage over other decision support tools is the inclusion of quantitative assessments to indicate where conservation efforts should be made, which features to target, and how best to protect them (Schwartz et al. 2018). Even though several conceptual frameworks have been proposed for ES conservation (e.g., Luck et al. 2012; Cimon-Morin et al. 2018; Villarreal-Rosas et al. 2020), there is currently no definitive approach, particularly regarding SCP, that has been widely adopted for implementation for the benefit of society (Villarreal-Rosas et al. 2020; Mitchell et al. 2021). Building on previous research (Cimon-Morin et al. 2014, 2016a; 2018; Cimon-Morin and Poulin 2018; Goyette et al. in press), we present an operational framework that bridges the gaps between conceptual schemes (e.g., Serna-Chavez et al. 2014; Villarreal-Rosas et al. 2020), and their practical applications to include ES protection and restoration objectives in SCP tools. We explain the general framework and steps of this approach and discuss concepts, challenges, innovations, and prospects for further development of comprehensive decision support tools for working landscapes. We believe that our approach can inform management decisions and planning for the sustainable provision of ES across multifunctional landscapes (Bennett et al. 2009). We illustrate our approach using two case studies from the Canadian ResNet research network (nsercresnet.ca; this issue). ResNet is a project that seeks to promote the production of insightful scientific knowledge to support Canada’s capacity to monitor, model, and manage working landscapes and all the ecosystem services they provide. The case studies we present are being conducted in the province of Quebec, which is referred to as Landscape 2 in the ResNet network. The first case study (Box 2) depicts how our approach can ensure continuous ES provision to beneficiaries through the use of ES capacity and demand. The second case study (Box 3) presents a methodology to fill gaps in ES benefits by managing urban vacant lots.
Box 2. Comparison of different SCP scenarios to secure ES provision.
The case study was undertaken in the Greater Quebec City region (∼1050 km2, ∼582 000 inhabitants) located in Quebec, Canada (Fig. 4; Gouvernement du Québec 2016). The study area consists mainly of natural habitats: forests (51%), wetlands (7%), and aquatic habitats (3%). Agriculture occupies 9% of the area, while other anthropogenic land-cover types reach ∼30%. The interface between urban, rural, and natural areas in the study region supports a combination of commodity production, such as from agriculture and forestry, with the provision of cultural and regulating services, such as flood attenuation and recreation. Hence, the economic, social, and ecological connections between people and nature in this region make it a good study model as a working landscape for evaluating how to plan wetland conservation to ensure ES provision. Regarding a compensation program for wetlands recently adopted at the provincial level (LQ 2017, c14), there is an urgent need to identify wetlands of high priority for conservation. Wetlands of the region provide several ES, among them (i) cooling effects to lessen heat islands, (ii) mitigation of flood risks, and (iii) recreational activities (Cimon-Morin and Poulin 2018).
We tested three scenarios of wetland ES spatial prioritization to assess which one better ensures that selected sites will truly offer service provision to beneficiaries. Before running prioritization analyses, we prepared the input data by following the first four mapping steps described in this paper. First, we delineated our planning units as the existent wetlands in the landscape. Then, using the best data available, we quantified the capacity 223 wetlands to provide the three ES considered (cooling effects, flood mitigation, recreational activities), following Cimon-Morin and Poulin (2018; see Supplementary Material 1A for more details). The flow area of each ES was also mapped for all the wetlands (i.e., providing areas). We then quantified the demand associated to each providing area and identified benefiting areas for each ES separately. For example, we used flood-prone areas to quantify the demand for flood attenuation (the greater the flooding area, the greater the demand), and then used drainage basins to link each wetland to its respective downstream flooding areas. Actual providing areas were identified based on which wetlands currently fulfill demand.
Three prioritization scenarios with optimization algorithms were compared using SCP software Marxan (Ball et al. 2009). The first scenario targeted 50% of each ES capacity only, the second targeted 50% of each ES capacity and demand separately (i.e., the approach presented in this paper) and the third targeted 50% of each ES provision index, which we estimated as the product of capacity and demand (capacity*demand; Villamagna et al. 2013; Watson et al. 2019). In each scenario, only the contribution of actual providing areas was considered to optimize the selection of sites that truly contribute to the delivery of services to beneficiaries. In these three scenarios, all ES features were weighted equally in terms of the importance of reaching the target. The cost of including a wetland in the solution was set equal to its area. Finally, the capacity, demand, and provision index captured in optimized solutions under the different scenarios were extracted (Fig. 5).
At the network scale, our results showed that targeting only the protection of ES capacity neither ensured that selected sites met a high demand nor contributed sufficiently to ES provision (Fig. 5a; Cimon-Morin et al. 2014). This result illustrates that capacity is a poor predictor of provision even though the prioritization focused on actual providing areas, which guaranteed that all candidate sites were both minimally supplied and in demand for one ES. Conversely, by setting targets for both ES capacity and demand in SCP analyses, it is possible to ensure that demand targets can be achieved, without even increasing the cost of the network (Fig. 5b; network cost of 1). More importantly, this approach ensures more balanced ES provision. Finally, targeting a provision index directly resulted in a network of sites with high demand but low capacity (Fig. 5c). While this scenario secured an equal amount of ES provision, but at a lower cost compared with the strategy targeting capacity and demand separately (Fig. 5b vs 5c), the selected sites may collectively be insufficient to guarantee sustainable ES provision. This result highlights the shortcomings associated with targeting either capacity or a provision index directly without considering capacity and demand as separate features.
Box 3. Vacant lots as ES provision restoration opportunities.
Benefit gaps, shortages in ES supply compared to demand, can be reduced or eliminated if ES provision is re-established through land management (Villamagna et al. 2013; Chaplin-Kramer et al. 2019). However, finding sites suitable for management is a challenge in regions intensively used by humans such as farmlands, commercial zones, and residential areas. Opportunity costs and social constraints are important determinants of the feasibility of restoration plans, defining areas in which restoration is realistic and acceptable (Miller and Hobbs 2007; Minnes et al. 2020). A solution to reduce the opportunity costs of restoration and obtain social support is to allocate management efforts in seemingly abandoned or unattractive spaces, such as former farm fields, brownfields (Anderson and Minor 2017), and channelized or polluted rivers (Wohl et al. 2015). In this case study, we focus on the use of vacant lots, unused long-abandoned lands, as opportunities for ES restoration due to the feasibility of managing them without major financial, social, and structural losses.
Our main objective is to evaluate the advantages and costs of managing vacant lots to improve the provision of ecosystem services in complementarity with natural ecosystems. We expect that by including the management of vacant lots in ES conservation plans, benefit gaps could be reduced or eliminated due to the reestablishment of ES capacity in vacant lots. The study area, the Greater Quebec City region (Fig. 4), is covered by a combination of urban, rural, and natural landscapes with vacant lots throughout its area, making it ideal for observing the outcomes of our approach in diverse landscapes. We have chosen to focus on five ES that are important in the context of the study area: carbon storage, recreation, cooling effects, aesthetic value, and pollination. We followed the six mapping steps presented in this study. Accordingly, ES capacity, demand and provision were estimated using a combination of fieldwork and literature data for 100 m × 100 m cells covering the entire study area (see Supplementary Materials 1B and Table S1 for details). Benefit gaps were identified as cells with positive ES demand but unsupplied (i.e., type 1 benefit gap).
Different possibilities for managing vacant lots were considered, such as transforming vacant lots into forests, urban parks, wildflower fields, and lawns (see Supplementary Material 1, Fig S1). The best type of management for reducing benefit gaps depends on management costs and targeted ES benefits. Costs include the price of land, price of management and delay in the return on investments (time until ES is fully restored), with a longer delay resulting in higher costs. Benefits include the increase of targeted ES provision after management. The final step in this study is to include current ES capacity, demand, expected ES capacity after management of vacant lots, and costs in an SCP approach with the objective of selecting sites to create optimal networks for restoring ES provision at lower costs. Finally, the resulting benefit gaps from prioritization exercises, including and not including vacant lots, along with the conservation of other greenspaces, can be compared to evaluate if the management of vacant lots can in fact improve overall provision of ES in the study area.
This case study illustrates an application of our approach to plan restoration with the objective of reducing benefit gaps (Fig. 1). The main innovative component of this case study is the development of a framework to consider different management types instead of a single restoration option (Sherren et al. 2020). The focus on vacant lots is also important because of the low social and financial constraints they impose compared to the management of productive or occupied land. We offer a practical solution for selecting sites for management in the face of specific ES needs. The same logic could be applied for different restoration techniques that have different costs (for example natural versus active ecosystem restoration) or outcomes (for example, when restoration objectives can differ). Our forthcoming results will indicate the impact of considering vacant lots for restoration using a prioritization approach for the overall provision of ES as well as the applicability of this method in landscapes with different compositions of urban, rural, and natural land uses.
Spatial prioritization considerations specific to ecosystem services
To date, most ES conservation frameworks have primarily tried to preserve the benefits ES provide to society (hereafter referred to as benefit-centered conservation; Villarreal-Rosas et al. 2020). ES benefits arise from the interactions among three essential components of the ES framework, the supply of the service (i.e., ES capacity), the demand for it (e.g., what people need and want), and the flow that connects areas of supply and demand spatially and temporally (see Box 1; Villamagna et al. 2013; Bagstad et al. 2014; Watson et al. 2019; Villarreal-Rosas et al. 2020). The interaction among these three components generates ES provision when and where benefits are actually delivered to society (Boesing et al. 2020; Mitchell et al. 2021). While ES provision represents the actual service delivered to society, most research still describes ES based primarily on capacity (Boerema et al. 2017; Rieb et al. 2017). For example, Villarreal-Rosas et al. (2020) recently showed that only 22% of the ES included in SCP studies were characterized using spatially explicit indicators of capacity, demand, and flow, whereas 46 % were portrayed using only capacity. This finding raises questions about whether or not most SCP studies are allocating priority areas in the right places.
Indeed, inefficient conservation investments and poor outcomes for beneficiaries may result from failing to consider one of the provision’s three components in ES spatial planning (Cimon-Morin et al. 2014; Watson et al. 2019; Villarreal-Rosas et al. 2020). For example, capacity is usually recognized as a poor spatial predictor of provision (Cimon-Morin et al. 2014; Watson et al. 2019; Mitchell et al. 2021). Therefore, spatial prioritization based only on capacity (see Box 2) can result in the selection of ecologically important areas that are highly supplied, but also divert conservation actions away from the most important places to provide actual benefits to humans (Cimon-Morin et al. 2014; Watson et al. 2019; Villarreal-Rosas et al. 2020; Mitchell et al. 2021). Conversely, putting too much emphasis on society’s needs (demand) could result in increasing the threats to already undersupplied ecosystems or lead to their overexploitation (e.g., ecological degradation of overcrowded nature recreation sites; Boesing et al. 2020). Thus, priority areas for ES conservation are not necessarily zones of either higher capacity or higher demand, but preferably those that are most important to ensure a continuous flow of benefits to beneficiaries.
Few studies have aimed to identify important sites for ES conservation based on their provision (Bagstad et al. 2014; Cimon-Morin et al. 2014, Schröter et al. 2014; Watson et al. 2019; Mitchell et al. 2021). For example, Watson et al. (2019) standardized capacity and demand for three ES on a scale of 0–1 and approximated the provision as their product (although they refer to it as “benefits”). The three provision values were then used in an SCP approach to identify ES priority areas across the state of Vermont (USA). While this approach ensures that sites with a provision value greater than zero deliver actual benefits to people (because it implies that both capacity and demand are greater than zero), focusing on such an index in conservation planning can lead to unpredictable and unbalanced levels of capacity and demand at both the site and network scales (see Box 2; Cimon-Morin et al. 2014). The main reason is that the product of capacity and demand does not provide information about the specific content of each site (e.g., equal provision values can originate from either a site with high capacity but low demand or vice versa). Consequently, securing a specific amount of provision could easily result in protecting sites high in demand but undersupplied, or the reverse, leading to sustainability issues. Similarly, Mitchell et al. (2021) identified ES hotspots across Canada based on an index of the combined provision of three ES. The index was calculated by summing the provision scores of the three ES, which were obtained by multiplying their normalized capacity and demand values. Although this approach may be useful to help identify areas critical for providing multiple ES to society (see Mitchell et al. 2021), relying on such an individual site scoring approach to identify a network of new priority areas also comes with great risks of undermining the representation of individual ES provision and would tend to provide less cost-efficient conservation solutions (Cimon-Morin and Poulin 2018; Wang et al. 2021).
The ultimate goal of “benefit-centered conservation” is to protect areas that supply services sustainably and are connected to places where benefits are currently enjoyed, used, or consumed (Schröter et al. 2018). Therefore, the challenge is how to best consider service provision (and all its components) in spatial planning to select areas with higher levels of sustainable benefit to society (Villarreal-Rosas et al. 2020).
Management strategies for optimizing working landscape ES provision
Historical land-use practices in working landscapes have resulted in substantial trade-offs among ES, where high societal demand for a limited number of services (e.g., food, timber, etc.) has led to increases in their supply but also caused declines in the quantity and quality of many others (e.g., water quality, pollination, carbon storage, pest and flood control, etc.; Foley et al. 2005; Bennett et al. 2009; Bennett et al. 2021). As a result, the demand or need for ES, notably for embedded ES underlying the production of other ES (Schröter et al. 2018), may exceed the capacity of ecosystems to supply them (Boesing et al. 2020; Winkler et al. 2021). This could, in turn, further exacerbate the need to rely on ecologically harmful land-use practices to maintain production, such as intensive applications of fertilizers and pesticides. ES losses could severely affect the resilience of working landscapes and their capacity to provide for a diversity of societal needs in the future. To avoid ES undersupply, potential management solutions include actions aimed at increasing the size and quality of the capacity pool or the flow connection with beneficiaries (i.e., decreasing isolation between supply and demand), which could be implemented using a combination of conservation interventions, such as protection and restoration (Boesing et al. 2020; Metzger et al. 2021).
Protected areas (PA) can play an essential role in securing ES capacity (Hodder et al. 2014; Castro et al. 2015; Hanna et al. 2020; Wei et al. 2020; Resende et al. 2021) and improving human well-being (Ferraro et al. 2011; Naughton-Treves et al. 2011; Canavire-Bacarreza and Hanauer 2013; Ferraro and Hanauer 2014). By effectively reducing the likelihood of future ecosystem conversion within their boundaries, PA can yield high-quality supplies of specific bundles of ES per unit area (e.g., water provisioning, recreation, carbon storage) while also ensuring at least a minimal but continuous flow. Nevertheless, several studies worldwide have shown that existing networks of PA often fail to protect vast areas most important for ES provision (Eigenbrod et al. 2009; Eigenbrod et al. 2010; Durán et al. 2013; Manhães et al. 2016; García Márquez et al. 2017; Xu et al. 2017; Lecina-Diaz et al. 2019; Neugarten et al. 2020). In fact, PA have historically been the primary mechanism for conserving biodiversity features (Rodrigues et al. 2004; Butchart et al. 2012; Le Saout et al. 2013; Lewis et al. 2019) and the methodologies used for their design were generally not intended to represent ES provision. Accordingly, planning the expansion of PA networks to ensure the highest return for human well-being requires the use of prioritization methodologies specially adapted to ES that include spatial considerations associated with their capacity, demand, and flow directly in the planning process (Luck et al. 2012; Cimon-Morin et al. 2014; Verhagen et al. 2017; Cimon-Morin et al. 2018; Watson et al. 2019; Villarreal-Rosas et al. 2020).
In the long run, working landscape sustainability may not be reached simply by protecting remnants of natural ecosystems as it may not prevent most ES from still being undersupplied regionally. Even though some ES benefits can be delivered by ecosystems in another region (i.e., food imports), one of the best strategies to foster sustainability in locations where ES are undersupplied relative to demand would be to increase the capacity pool (quantity and quality) via active ecosystem management on a site-by-site basis (Schröter et al. 2018; Boesing et al. 2020; Metzger et al. 2021), such as through restoration actions (Possingham et al. 2015; Barbosa et al. 2019). In conjunction with ecosystem-based management or reduced-impact approaches, production could be reestablished on altered sites as part of a strategy aimed at fostering the emergence of multiple ES without disproportionally interfering in the production-centered objective of working landscapes. Such an approach could conceivably even improve the production capacity of provisioning ES (e.g., crop and timber yield). One of the advantages of restoration is that it allows the reestablishment and accessibility of bundles of ES where beneficiaries lack a connection with ES benefits (i.e., disrupted flow) or in cases of low or absent supply. For example, the restoration of altered commercial forest paired with ecosystem-based management not only increases the amount of harvestable timber but also favors other ES (e.g., carbon sequestration, water flow regulation, recreation) and some biodiversity components (Chazdon 2008; Ciccarese et al. 2012).
Choosing whether to protect or restore and where these solutions can be implemented in the landscape to provide enhanced and diversified provision of ES are decision-making problems that can be solved by spatial planning. Below, we present an operational framework, divided into mapping and spatial planning stages, that was developed to assist in the protection and restoration of ES provision.
Mapping ES provision for conservation planning
Assuming that ES provision occurs only when three conditions are met: (i) ES are supplied, (ii) a minimal amount of demand is fulfilled, and (iii) supply and demand are interlinked (i.e., flow), generating benefits, we present a mapping approach that can be used to integrate these three components of ES provision and prepare the input data required for the spatial prioritization process (see Supplementary Materials 1A and 1B for examples of ES mapping; Cimon-Morin et al. 2014, 2018). Prior to mapping, planning units (which are the spatial units for compiling data and which will later be prioritized) need to be defined. They are generally delineated as either a grid of uniform cells (squares or hexagons) or irregular polygons such as habitat patch outlines or tenure parcels (Nhancale and Smith 2011). Each planning unit is associated to a protection or restoration status based on its ecological conditions and whether or not its current level of alteration may undermine ES provision. Restored planning units can also be integrated into a network of protected areas if the restored ES provision would be better supported by this type of management action (see the next section for details).
The first step involves mapping ES capacity in each planning unit using various methodological approaches and based on best available data and resources (Fig. 1; Martínez-Harms and Balvanera 2012). For the planning units associated with a restoration status, ES capacity should be quantified in two different ways: (i) as predicted values assuming that restoration actions would be entirely successful and (ii) as the current capacity of sites based on their altered conditions (see the next section for details). Mapping ES capacity enables identification of planning units that can be considered as providing areas (see Fig. 2; Syrbe and Walz 2012; Serna-Chavez et al. 2014). However, as suggested earlier, this step alone does not provide sufficient information for making appropriate benefit-centered conservation choices, since not all providing areas yield actual benefits for human populations. They might, for example, be inaccessible or not in demand.
Fig. 1.
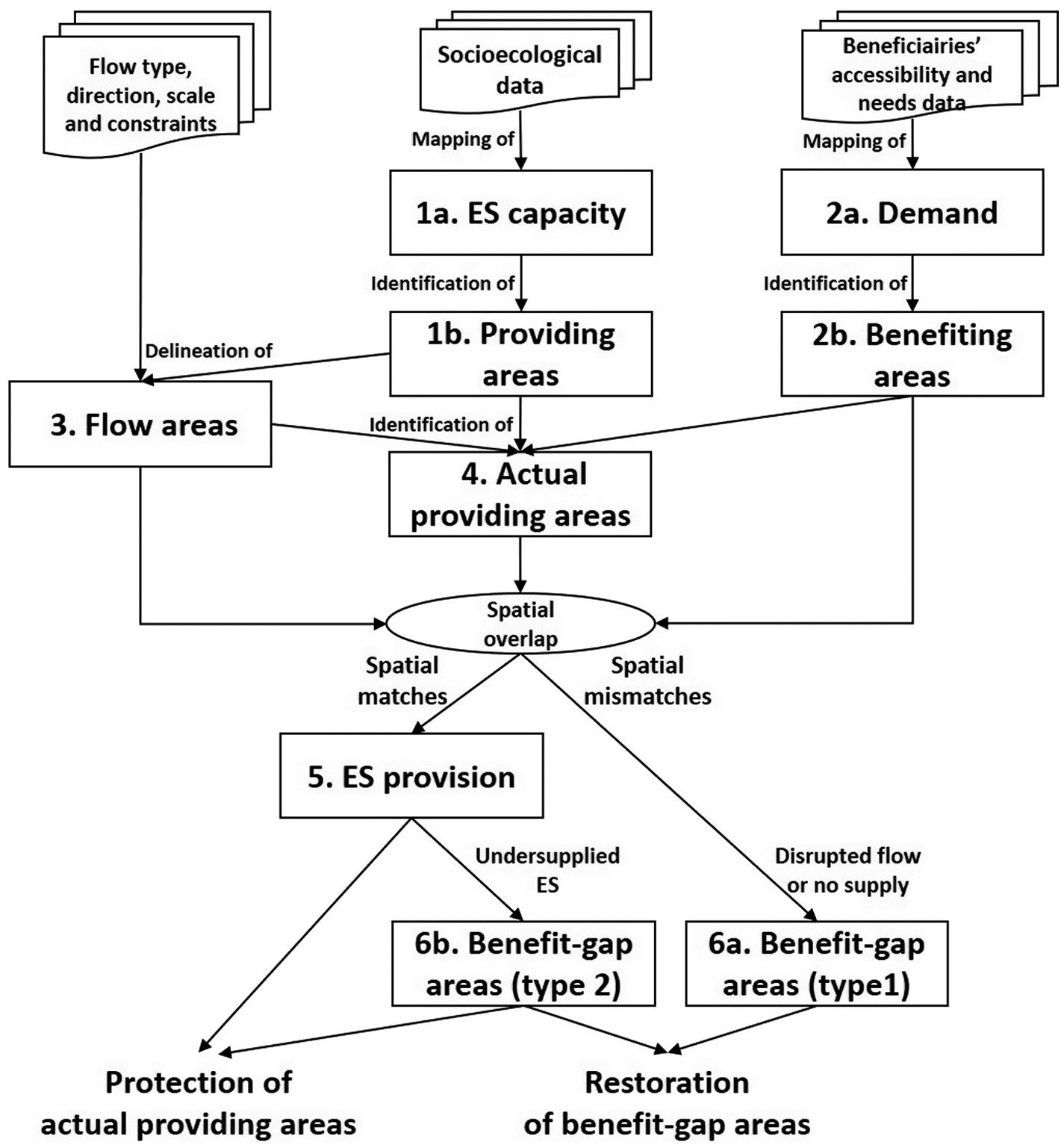
Fig. 2.
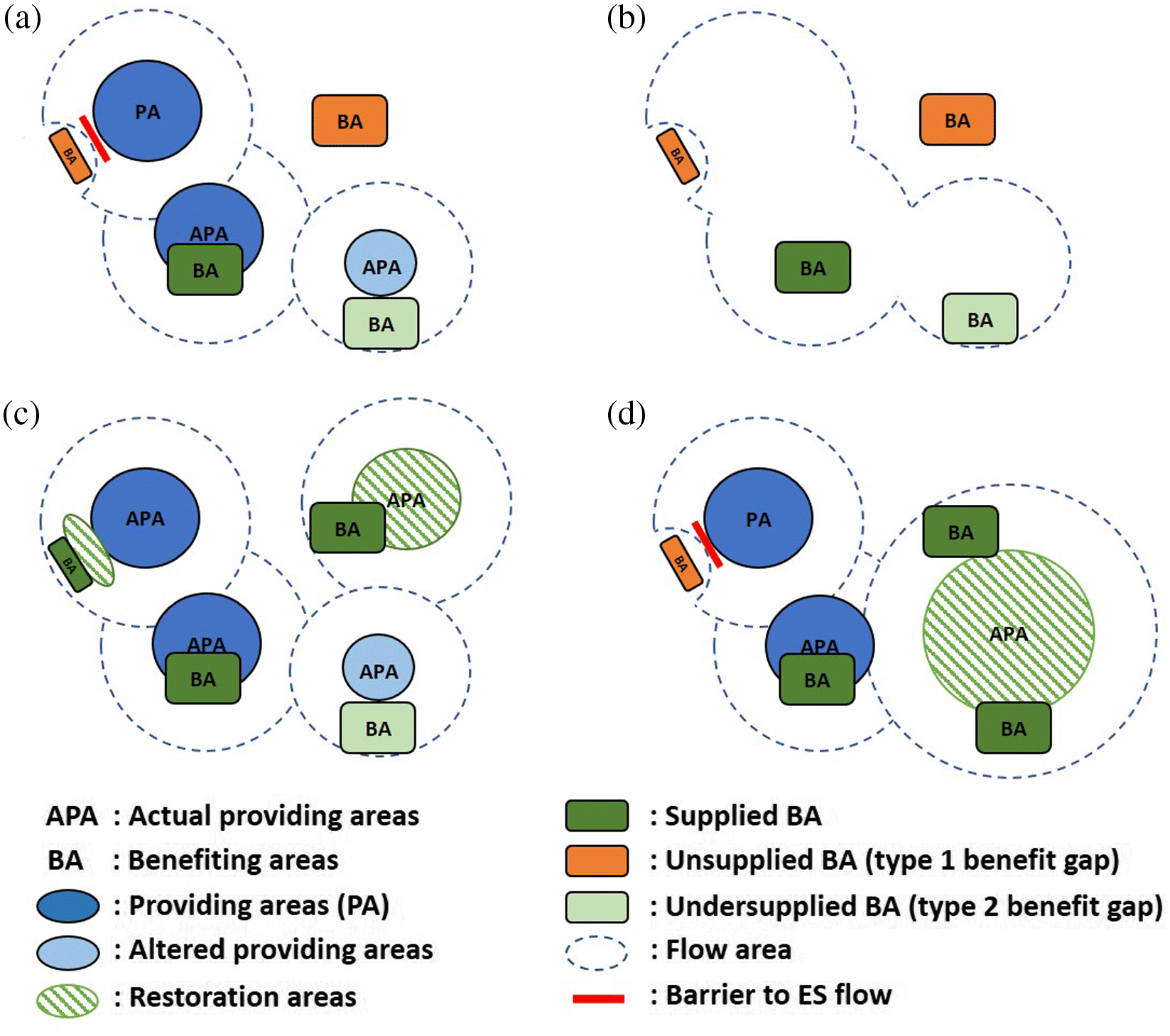
Therefore, the second step involves mapping demand for each ES providing area using quantitative and spatially explicit indicators that reflect both the needs of beneficiaries and their ability to access supply (Cimon-Morin et al. 2014; Wolff et al. 2015; Mitchell et al. 2021). The quantification of demand should consider that, for some services (notably provisioning and cultural ones), beneficiaries may have to move towards areas of supply (e.g., national parks, hunting areas, etc.) to obtain benefits. For example, Mitchell et al. (2021) estimated demand for nature-based recreation based on the ability of people to visit providing areas (i.e., road access) and the number of potential beneficiaries in the vicinity (i.e., population density). At this step, multiple beneficiary groups, with different values, needs, and vulnerabilities, who depend on ES benefits for their livelihood, can be integrated (see the “Challenges and future work” section for more details). A quantitative demand value for each ES is then attributed to each providing area. Expressed spatially, mapping demand enables the delineation of which planning units are considered benefiting areas for each ES (Syrbe and Walz 2012; Serna-Chavez et al. 2014). Then, the spatial overlap of ES capacity and demand (i.e., between providing areas and benefiting areas) yields zones of ES provision, that is to say where both capacity and demand are greater than zero, enabling demand to be fulfilled, at least partially (Cimon-Morin et al. 2014; Mitchell et al. 2021).
Nevertheless, for most ES, a spatial mismatch occurs between providing and benefiting areas (Serna-Chavez et al. 2014; Winkler et al. 2021). For example, providing areas for pollination services (i.e., wild pollinator habitat patches; Figs. 2a and 2b) do not generally coincide spatially with their benefiting areas (e.g., agricultural fields). In fact, pollination benefits are also accessible within the flow area, which in this case, depends on proximity and is delineated by species-specific maximal foraging distances around each wild pollinator’s habitat patches (Lonsdorf et al. 2009; Serna-Chavez et al. 2014; Perennes et al. 2021). Knowing which benefiting areas rely on which providing areas for the delivery of benefits is mandatory for ES prioritization and spatial planning decisions. Therefore, the third step of our approach consists of mapping each ES flow area based on (i) its specific spatial scale, which varies from in situ to global; (ii) its direction (e.g., unidirectional, omnidirectional); (iii) its type (e.g., flow of species and matter, or through human movement); (iv) potential biophysical barriers capable of restricting its spatial extent; as well as (v) anthropogenic processes that can extend or facilitate the flow (Cimon-Morin et al. 2013; Villamagna et al. 2013; Serna-Chavez et al. 2014; Schröter et al. 2018; Metzger et al. 2021). For example, a water provisioning flow area has a regional scale (i.e., watershed) where the service is delivered from upstream providing areas to downstream benefiting areas along the direction of the water flow, while being potentially impaired by barriers to water flow, such as dams, or extended by canals and exportation. At the fourth step, flow areas are superimposed on the benefiting areas, which enables the identification of providing areas that fulfill at least a minimal amount of demand (i.e., hereafter actual providing area). A planning unit can be considered an actual providing area for one or more ES, yet not for some others (i.e., because of no demand or disrupted flow for them).
The spatial visualization of ES provision (step 5) can reveal either spatial matches or mismatches between areas of benefit availability and demand (Figs. 1 and 2). A spatial mismatch occurs when one of the three components of ES provision is missing. For instance, where there is demand but either no capacity or no flow connection, such as when a benefiting area falls outside the spatial coverage of any flow area, a benefit gap is created (Figs. 1 and 2a). Restoration actions can help manage benefit gaps, for example, by creating new supply patches (i.e., increasing capacity quantity) or by removing barriers to ES flow to expand a currently disrupted flow area, which will facilitate the connections between areas of capacity and demand (Fig. 2c; Metzger et al. 2021). For this first type of benefit gap (type 1), where no benefits are provided to a benefiting area, a separate analysis must be conducted to identify additional management sites (i.e., new providing areas that were potentially not considered in steps 1 and 2 because of no capacity or disrupted flow) and their associated best restoration trajectory (Step 6a). The trajectory should be based on (i) the local bundle of ES with a capacity deficit, (ii) management costs and benefits, and (iii) the recovered capacity after implementation of management actions (see Box 3 for an example of a benefit-gap restoration framework). However, at the time of analysis, these areas only provide “virtual” benefits, which highlights the importance of implementing restorations actions quickly if these sites are selected. Another mismatch situation, which is however not considered a benefit gap, can occur where there is both capacity and flow but no demand, in which case demand must be acted on directly (e.g., by increasing access to capacity by road construction), a scenario beyond the scope of this study. In other words, if a site is not in demand (but being supplied) for an ES, it should not be selected for protection or restoration, at least when spatial planning focuses on immediate benefits (but see the “Challenges and future work” section).
Even when capacity, demand, and flow yield ES provision (i.e., spatial match) a second type of benefit gap can occur (i.e., type 2; see Fig. 1 step 6b and Fig. 2d). For example, where ES is undersupplied due to capacity being undermined by the degree to which the ecosystem has been altered, restoration actions could help increase the quality and quantity of supply and maximize the benefits provided. Such areas correspond to the planning units that were previously associated to restoration needs. In summary, our mapping approach enables the user to map and integrate the different components of ES provision so that it is possible to formulate a spatial prioritization based only on those planning units that can provide at least a minimal amount of benefits to people.
A systematic conservation planning approach for ES provision
Systematic conservation planning (SCP) is increasingly recommended for identifying priority areas for ES conservation (Chan et al. 2006; Cimon-Morin et al. 2013; see Box 1). Villarreal-Rosas et al. (2020) recently proposed a framework to incorporate the components of ES provision when scoping the decision problem underlying SCP. More specifically, it aims to (i) recognize the impact of ecosystem degradation on human well-being (i.e., planning problem definition), (ii) define management objectives linked to human well-being, (iii) quantify ES provision components (capacity, demand, and flow), (iv) identify actions to achieve objectives (solutions), (v) identify possible constraints to solutions, and (vi) describe how solutions will influence ES provision and benefit delivery (for more details see Villarreal-Rosas et al. 2020). The SCP approach we propose below is in line with this framework and expands it by providing an operational procedure to identify where practical solutions (protection and restoration) could be implemented to manage ES provision and the benefits delivered to society. While SCP is a comprehensive conservation procedure and involves multiple stages (see Box 1; Pressey and Bottrill 2008; McIntosh et al. 2016), our approach focuses on the stages related to identifying priority areas.
During spatial prioritization, all conservation features (ES) are considered simultaneously. Indeed, as the spatial congruence between different ES can be weak (i.e., spatial trade-off), a key advantage of using SCP lies in its complementarity-based approach. The sites selected work synergistically to achieve conservation objectives and produce cost-efficient solutions. Also, with the possibility of setting management costs as constraints in the selection process (e.g., acquisition or restoration costs, opportunity cost, etc.), the trade-off between ES conservation and the production-centered objective of working landscapes can be minimized. For example, in a forested landscape, the cost of protecting a planning unit could include the opportunity cost of forestry, which is defined as the cost of foregone opportunities (Naidoo et al. 2006), estimated as the value of the site’s sustainable timber yield. When these costs are included, an SCP approach can identify which planning units are most important for maintaining ES provision while also minimizing the loss in terms of harvestable timber value. In other words, conservation actions can be designed to minimize impacts on different stakeholders and other economic sectors.
In SCP tools, the user must provide the software with the value of each conservation feature present (each ES in this case) in each planning unit and for which different conservation targets are set (Fig. 3). One aspect that distinguishes our approach is that instead of considering either capacity or a provision index (e.g., capacity*demand) for each ES as a conservation feature, we rather consider the capacity and demand of each ES as two separate features (Cimon-Morin et al. 2014). For example, conservation targets could be set to reach 20% of the cooling effect provided by urban vegetation to beneficiaries (capacity) and 40% of the demand for it (where it is needed), while targets for flood control capacity and demand could be set at 30% each. Targets for ES capacity can (when possible) be set to match the sum of the benefits that society currently obtains from a particular area. In contrast, targets for demand can be calibrated to ensure that the site selection process will yield the highest returns for beneficiaries at the lowest cost (Cimon-Morin and Poulin 2018). The rationale behind targeting capacity and demand of each ES separately is that it is possible to determine in advance how much of each feature will be represented in the conservation network. For example (see Box 2), targeting each capacity and demand separately was more effective in protecting provision than directly targeting a provision index of each ES (Cimon-Morin et al. 2014). Furthermore, even the use of an index representing overall ES capacity (or demand or both) for each site (all ES combined) could still underrepresent some ES (Cimon-Morin and Poulin 2018).
Fig. 3.

Nevertheless, considering capacity and demand separately in SCP also introduces an issue inherent in ES target-based conservation planning that we encountered in previous research. A planning unit can be selected for its contribution to the capacity of an ES even though the demand for it shows a nil value (or vice-versa). Actual providing areas were specifically mapped to overcome this issue. In fact, we recommend that only the capacity and demand originating from actual providing areas (those that fulfill demand) and type 1 benefit-gap areas should be compiled in planning units that will be accounted for during prioritization. For example, a planning unit that is an actual providing area for pollination (supplied and in demand), but not for its cooling effect (supplied but no demand), should only be considered for its contribution to pollination provision (but see the “Challenges and future work” section). Considering all providing areas instead of only actual providing areas can result in a failure to meet the main objective of an ES benefit-centered conservation plan. Focusing on actual providing areas ensures that all candidate planning units can procure a minimal amount of provision for at least one ES. Because SCP seeks efficiency, targeting both capacity and demand has generally favored the overall selection of sites where both capacity and demand are simultaneously high for multiple ES (Box 2), which suggests that the approach prioritizes sites that procure greater ES provision (Cimon-Morin and Poulin 2018; Goyette et al. 2021).
Planning for the restoration of ES provision consists mostly of either filling the spatial gap between unfulfilled and fulfilled ES demand (type 1 benefit gaps) or increasing the capacity of undersupplied ecosystems (type 2 benefit gaps) and involves a method similar to that described above (Goyette et al. 2021). During the site selection process, the main distinction between planning units for protection and those for restoration lies in their cost, since restoration generally involves greater cost per unit area (for implementing restoration actions). The total restoration cost will also depend on whether restored sites would be protected or not. Indeed, restored sites in working landscapes could be either protected or brought back into production using ecosystem-based management or other integrative approaches more conducive to the maintenance of multiple ES. The total cost of restoration should reflect the preferred management option chosen for each site (e.g., excluding site acquisition cost if not protected). While protection and restoration planning could be done in separate assessments, we suggest they be integrated within the same conservation exercise. For instance, planning simultaneously for both protection and restoration makes it possible to consider the contribution of restored sites (using predicted capacity values) to conservation objectives. These sites could not only have good intrinsic value once they have been restored (whether protected or not) but also increase network connectivity and contribute to ES provision.
Even before any restoration actions are undertaken, most altered sites can already contribute to provision, at least to a certain degree (i.e., type 2 benefit gap). For instance, they can provide connectivity within the whole network or contribute to carbon sequestration (Wallington et al. 2005; Lindenmayer and Noss 2006; Doerr et al. 2011). Therefore, a separate analysis can be carried out in which the ES capacity of disturbed sites corresponds to their current condition, and in which the cost to include them in the network would be lowered when compared with a restoration scenario (e.g., set equal to their protection cost, excluding restoration action costs). By comparing the results of this second analysis with those from application of the main approach, it would be possible to assess the contribution of altered sites to meet overall conservation objectives.
Challenges and future work: toward a more comprehensive approach
Maintenance of service providers
Currently, most ES conservation frameworks try to focus on preserving the flow of benefits to society. However, in some contexts, benefit-centered conservation is not entirely in line with the ultimate goal of ensuring the sustainability of ES benefits, since the specific habitats that support service providers may not be fully taken into account (Fig. 3, dashed lines). For example, the spatial prioritization that resulted from applying our approach to local flow ES in a remote region in regard to moose (Alces alces) hunting, directly resulted in the selection of areas that would benefit local populations (i.e., where moose are hunted by beneficiaries; Cimon-Morin et al. 2014, 2018). However, as most of this region is largely inaccessible, the sites secured were mostly concentrated along its southern edges. In the long run, the sustainability of the hunting potential there (i.e., the benefits) may greatly depend on the process by which moose immigrate from outside the protected providing areas and then disperse. Since the region is prone to industrial development (Cimon-Morin et al. 2016a), sustaining this service may require protection of the supply (and—the service providers—moose populations and their habitats) outside of the spatial range of this ES provision.
To fully sustain ES provision, we argue that ES protection and restoration should integrate both benefit- and provider-centered considerations simultaneously, at least when a provider can be identified and associated with supporting habitat management requirements. Even though these requirements may be identified more easily for certain services (such as game or iconic species or those directly associated to certain species populations), almost all ES could be linked to a specific provider, such as a collection of individuals, species, or functional groups having specific habitat and connectivity needs (Luck et al. 2009). While the maintenance of ES providers is more connected to the biodiversity conservation realm, unstable provider “populations” could compromise ES provision conservation. Considering that the spatial congruence of providers (i.e., biodiversity components) and actual benefits (delivered by those providers) can be low in certain contexts, it is essential to include them in the same SCP assessment to increase complementarity and conservation efficiency (Cimon-Morin et al. 2013, 2016b; Watson et al. 2019). Finally, a provider-centered conservation approach would also be crucial, acting as an insurance policy to meet future demand in a world undergoing continual change, notably in terms of climate and human population size. Little additional data on providers would be necessary; for example, linking habitat suitability models (Polasky et al. 2005; Edenius and Mikusinski 2006; Shifley et al. 2006) with ES capacity in places where the capacity lacks protection could suffice.
Ensuring benefit sustainability at the site scale
Overall, at the conservation network scale, our approach (Box 2 and Fig. 5b) makes it possible to attain the main objective of benefit-centered conservation, which is to ensure a continuous flow of benefits to people. Indeed, it allows us to not only reach targets of both ES capacity and demand but also generally favors the selection of planning units that provide high levels of provision (i.e., high ES capacity and demand values for a specific service) because SCP seeks efficiency. Nevertheless, at the planning unit scale, our approach raises an issue inherent in almost all spatial planning exercises involving multiple ES, which is that it does not currently guarantee that each site in the network can sustain its own level of demand. For example, some selected sites can be associated with high demand but relatively low supply (intra- or interservice). According to Villamagna et al. (2013), provision of an ES is considered sustainable only when fulfilling demand does not increase pressure or degrade ecosystem capacity to provide that service or cause declines in others. Several ES may require a minimum local area to sustain provision, notably to allow underlying ecological processes to operate (Kukkala and Moilanen 2017). For example, some services, such as outdoor recreation, typically cannot be provided by very small areas, while pollination may have both local area size and connectivity requirements (Kukkala and Moilanen 2017). More importantly, different levels of demand may increase the area threshold that these ES require to sustain provision. It is crucial to identify for which ES “unbalanced” capacity and demand could be problematic and assess their minimal area and connectivity requirements. These requirements could be included in the SCP process or could at least help identify potential sites for restoration (type 2 benefit gap).
Fig. 4.
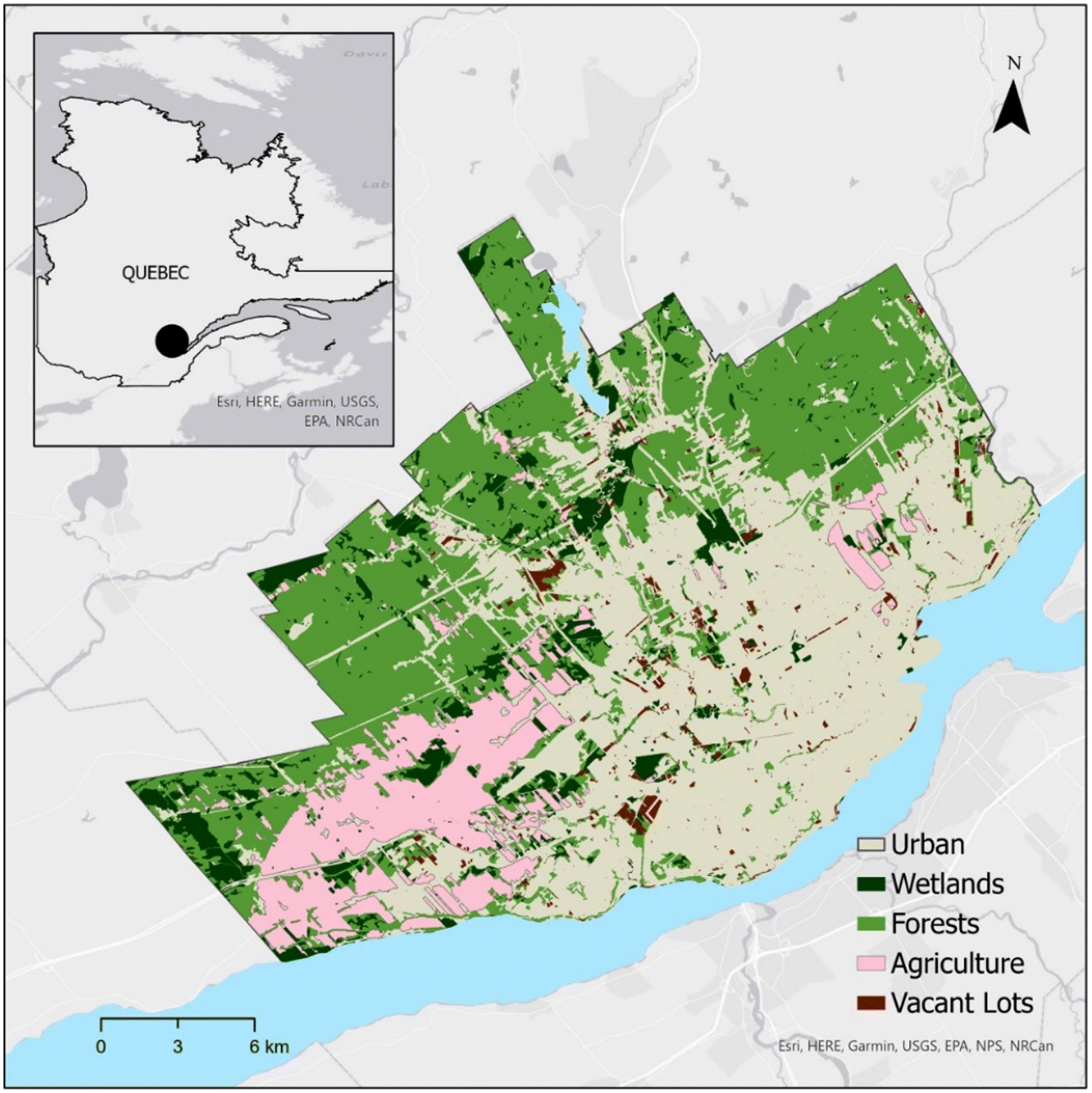
Fig. 5.

Ensuring equitable access and distribution of benefits to different groups of beneficiaries
Ensuring that interventions to conserve ES benefits for one group are not detrimental to others is a major concern emerging in ES SCP studies. Addressing this issue involves recognizing that different groups of people may either have inequitable access to ES benefits or that their needs may have a heterogeneous spatiotemporal distribution (Villarreal-Rosas et al. 2020). Beneficiary disaggregation may represent a step forward in understanding the disparities in the benefits certain groups derive from services (Daw et al. 2011; Grima et al. 2019; Villarreal-Rosas et al. 2020). For example, in the north shore region of Quebec (Canada), moose hunting is practiced by two main beneficiary groups: (i) members of Indigenous communities, who engage in subsistence hunting within their ancestral territory, and (ii) recreational hunters, who are mostly concentrated along the southern edge of this area because of limited road access and infrastructures (see Cimon-Morin et al. 2014, 2016b). Even though the two groups seek the same benefits (i.e., moose meat), combining these two beneficiary groups in a prioritization approach could have resulted in suboptimal conservation decisions with potential negative outcomes for one or both. Several solutions could be explored to address beneficiary disaggregation in SCP approaches.
One solution involves the use of a serviceshed-based approach, which has been used as a spatial unit to account for social inequities in how management interventions affect the benefits local people derive from ES (Mandle et al. 2015; Tallis et al. 2015). By including factors influencing human access to benefits, such as infrastructures, accessibility, and other socioeconomic factors, delineating a serviceshed enables identification of which providing areas are important for delivering ES benefits to specific groups of beneficiaries (Mandle et al. 2015; Tallis et al. 2015). Once these providing areas have been identified, they can be separated into two distinct features. Continuing with our example above, actual providing areas for moose hunting could be categorized as (i) actual providing areas for Indigenous communities and (or) (ii) actual providing areas for recreational hunters (Cimon-Morin et al. 2014, 2016b). Then, different conservation/restoration targets could be set for the capacity and demand of each of these two features, to ensure equitable distribution and access to benefits for both groups of beneficiaries.
Another solution would consist of weighting ES demand during mapping to further reflect the values, needs, and vulnerabilities associated with the livelihood of different beneficiary groups (Villarreal-Rosas et al. 2020). For example, when quantifying demand specifically for the recreational moose hunting group, Cimon-Morin et al. (2014, 2016b) attributed greater importance to providing areas located near outfitters and vacation leases, which are highly dependent on hunting-related tourism. The serviceshed approach could very well be combined with weighting ES demand to include a second level of disaggregation, for example, promoting conservation near outfitters and vacation leases for actual providing areas for recreational hunters and near hunting camps for actual providing areas within the ancestral territory of Indigenous communities.
Accounting for delays in the recovery of ES supply after restoration
In our approach, ES capacity is included in restoration planning as a predicted value toward which sites should tend if restoration actions are entirely successful (Goyette et al. 2021). Nevertheless, restoration success is often unpredictable, as the recovery rate can be much lower than expected (Moreno-Mateos et al. 2012), and the “full recovery” of altered ecosystems may often take decades (Benayas et al. 2009; Moreno-Mateos et al. 2012; Moreno-Mateos et al. 2017; Lira et al. 2019; Sherren et al. 2020). A solution would be to consider recovery time lag as a “return on investments” (Possingham et al. 2015). Consequently, sites with predicted slower recovery time or those less likely to fully recover would be associated in SCP with greater management costs than those more likely to provide ES more rapidly.
Integrating the maintenance and restoration of ES flow
Continuous land-use changes may result in the shrinkage or disruption of flow connections between providing and benefiting areas (see Figs. 2a and 2c). For example, considering upstream wetland capacity to mitigate pulses of downstream floods, any modification of the main channel course and conditions such as dredging or straightening as well as the loss of natural floodplains or riparian habitats could (i) impair the hydrological connections between areas of capacity and demand (even if their individual values remain constant), (ii) alter the flow, and ultimately (iii) result in a decrease of actual benefits to society. Considering connecting areas is a necessary step for the development of a comprehensive ES spatial planning framework (Syrbe and Walz 2012). On the one hand, actions to protect connecting areas may be a prerequisite for maintaining the delivery of certain ES benefits such as those modulated by passive biophysical flow through processes in air, water, and soil (e.g., flood control, water provisioning, etc.) and flow mediated by species movements (e.g., migratory birds hunting, etc.; Schröter et al. 2018; Kleemann et al. 2020). On the other hand, actions to restore connecting areas may successfully contribute to expanding the flow area and connect providing areas to new potential benefiting areas. For instance, restoration actions could include the creation of green corridors and network infrastructures or the removal of barriers to ES flow (Metzger et al. 2021). Integrating the management of ES flow in our spatial planning approach involves identifying the providing area where the flow area originates (or will be expanded) as well as the appropriate management interventions. This analysis could be conducted simultaneously with the type 1 benefit-gap assessment. Then, the cost of the management interventions for the connecting area could be added to the management cost of the corresponding providing area. Once again, separate analyses can be carried out to isolate the impact of flow management on the overall efficiency of the network (i.e., increase in demand fulfilled compared to the cost of the network), for example, to assess the impact of choosing between the sole protection of a providing area (e.g., for provision of the other ES it already supplies) or both its protection and the restoration of a portion of its flow area to connect an additional benefiting area. Nevertheless, a new challenge arises when the connecting area is also a providing area for other services; the choice to restore or protect may become more difficult in such a case. We believe that there is still much scope for further development of strategies to include connecting areas in SCP.
Increasing the contribution of production areas to ES provision
In addition to conservation actions, optimizing working landscape multifunctionality also requires the implementation of alternative land-use practices more favorable to the maintenance of sustainable ES provision in production areas. For example, shifting from a strict and intensive clear-cutting harvest regime to a reduced-impact management system based on selective harvesting in certain parts of a forested landscape could contribute to the maintenance of multiple ES capacity, such as nontimber forest products, recreation, aesthetics and regulation of water flow and quality (Pohjanmies et al. 2017). Similarly, widening certain riparian buffer strips and managing their vegetation cover (i.e., afforestation with fast growing species) in agricultural landscapes could help increase their capacity to mitigate diffuse pollution, sequester carbon, and provide aesthetic and cultural values (Cole et al. 2020). Despite the potential contribution that alternative land use could bring to ES provision, large-scale application of such land use could be particularly challenging in working landscapes, as they generally involve (i) an increase in implementation and ongoing management costs or (ii) potential trade-offs with production-centered objectives (e.g., such as yield losses per unit area or land taken out of production). Therefore, spatial planning is no longer just a matter of identifying where to invest in conservation, but it must now also address alternative strategies in what and where to invest (Watts et al. 2009; Wilson et al. 2010; Law et al. 2017).
SCP offers the possibility of including a variety of alternative land-use strategies in the spatial prioritization process. In Marxan with zones (i.e., an SCP software) for instance, any parcel of land can be allocated to a specific zone, where each zone is defined by its own management actions, objectives, constraints and contribution toward achieving conservation targets (Watts et al. 2009). The contribution of a zone is defined as the level of protection offered to each conservation feature (e.g., ES capacity). For example, recreation capacity in a planning unit, if present, could be considered fully represented if allocated to a zone with a protection status, partially represented by a partial-harvest zone, and not represented by a clear-cutting zone (Watts et al. 2009). In a study conducted in East Kalimantan (Indonesian Borneo), Wilson et al. (2010) considered eight land-use zones for biodiversity conservation, three of which were classified as “forestry production zones” associated to different forest cover retention thresholds (i.e., reduced-impact logging practices) with different contributions to species representation. They found that accounting for the contribution of reduced-impact forestry toward meeting their conservation objectives substantially lowered the area required to be under strict protection as well as total cost compared with a scenario relying only on protected areas (Wilson et al. 2010). In summary, by considering a range of land-use options, SCP enables optimization of the efficiency of ES provision conservation across entire landscapes.
Conclusion
We have described an integrated systematic conservation planning approach that seeks to optimize ES provision to attain benefit-centered conservation objectives. In its current form, this approach has already yielded promising results when applied to working landscapes, such as in regions subject to forestry and mining (Cimon-Morin et al. 2014, 2016a) as well as in agricultural settings (Goyette et al. 2021) and in an urban context (Cimon-Morin and Poulin 2018). The ResNet project has offered us the opportunity to consolidate our previous ideas on how to deal with challenges and pitfalls related to ES conservation planning (Cimon-Morin et al. 2013, 2018) into a practical framework. This framework is an important step in synthesizing best practices to guarantee ES conservation plans that meet beneficiaries’ needs, sustain ES provision, and maintain service providers.
Documentation of the use of SCP as a decision support system to identify optimally designed ES conservation networks is only beginning to appear in the literature, and there is a considerable need for testing its effectiveness under different scenarios (Egoh et al. 2007; Cimon-Morin et al. 2013). We have proposed solutions to deal with the two main challenges, representing both ES capacity and demand and simultaneously considering restoration and protection objectives in SCP. We advocate for setting targets for both ES capacity and demand using SCP, since our results suggest that targeting only capacity or a provision index leads to network underperformance (see Fig. 5 and Box 2). To simultaneously address restoration and protection targets, we propose the inclusion of additional mapping steps such as (i) accessing site suitability for ES restoration; (ii) identifying ES benefit gaps, areas with demand but low or no ES capacity; and (iii) predicting capacity after ES restoration. In the example shown in Box 3, four possible management strategies for ES restoration were proposed for the specific context of an urban and peri-urban area. However, other types of management may be included depending on regional context. In riverine landscapes, for example, types of management could include riverbank soil stabilization for erosion control, phosphorus filtration for pollution control, and ecological restoration of riparian vegetation.
Proposing strategies to guarantee the sustainable provision of ES for human society is a challenging task. Methods such as SCP are unquestionably important for developing such strategies (Margules and Pressey 2000). However, there is still room to improve our knowledge of methods for decision-based support to promote ES conservation. The objective of this framework is to build a collection of best practices that aim to include ES in a SCP approach in a way that can be applied in a variety of landscapes, as well as at different spatiotemporal scales. The success of the application of our framework in different landscapes, such as areas dominated by forestry, fisheries, or agriculture, requires on establishing clear conservation objectives, understanding the context-relevant flow of ES, and proposing strategic management practices for ES restoration. We encourage researchers and conservation practitioners to consider the importance of including all three components of ES provision (capacity, demand, and flow) when planning for ES protection as well as restoration scenarios when the regional context is characterized by ES benefit gaps.
Acknowledgements
The authors would like to thank H. Thierry, J.P. Metzger, and one anonymous reviewer for their comments on the manuscript. We also wish to thank K. Grislis for stylistic revision. We acknowledge the support of the Natural Sciences and Engineering Research Council of Canada (NSERC), [funding reference number NSERC NETGP 523374-18]. Cette recherche a été financée par le Conseil de recherches en sciences naturelles et en génie du Canada (CRSNG), [numéro de référence NSERC NETGP 523374-18].
References
Albert C, Aronson J, Fürst C, and Opdam P. 2014. Integrating ecosystem services in landscape planning: requirements, approaches, and impacts. Landscape Ecology, 29: 1277–1285.
Anderson EC, and Minor ES. 2017. Vacant lots: An underexplored resource for ecological and social benefits in cities. Urban Forestry and Urban Greening, 21: 146–152.
Ang F, and Van Passel S. 2012. Beyond the Environmentalist’s Paradox and the Debate on Weak versus Strong Sustainability. BioScience, 62: 251–259.
Bagstad KJ, Johnson GW, Voigt B, and Villa F. 2013. Spatial dynamics of ecosystem service flows: A comprehensive approach to quantifying actual services. Ecosystem Services, 4: 117–125.
Bagstad K, Villa J, Batker D, Harrison-Cox J, Voigt B, and Johnson GW. 2014. From theoretical to actual ecosystem services: mapping beneficiaries and spatial flows in ecosystem service assessments. Ecology and Society, 19: 14.
Bahadur KCK, Fraser E, Dieleman CM, Green AG, Turetsky M, and Bysouth D. 2020. Opportunities for expanding agriculture in Canada’s North and their potential ecosystem tradeoffs. FACETS, This issue.
Ball I., Possingham H, and Watts M. 2009. Marxan and relatives: software for spatial conservation prioritization. In Spatial conservation prioritization: quantitative methods and computational tools. Edited by A Moilanen, K Wilson, and H Possingham. Oxford University Press, Oxford.
Barbosa A, Martín B, Hermoso V, Arévalo-Torres J, Barbière J, Martínez-López J, et al. 2019. Cost-effective restoration and conservation planning in Green and Blue Infrastructure designs. A case study on the Intercontinental Biosphere Reserve of the Mediterranean: Andalusia (Spain) – Morocco. Science of The Total Environment, 652: 1463–1473.
Benayas JMR, Newton AC, Diaz A, and Bullock JM. 2009. Enhancement of biodiversity and ecosystem services by ecological restoration: a meta-analysis. Science, 325: 1121.
Bennett EM, Baird J, Baulch H, Chaplin-Kramer R, Fraser E, Loring P, et al. 2021. Chapter One - Ecosystem services and the resilience of agricultural landscapes. In Advances in Ecological Research. Editors DA Bohan and AJ Vanbergen. Academic Press. pp. 1–43.
Bennett EM, Cramer W, Begossi A, Cundill G, Díaz S, Egoh BN, et al. 2015. Linking biodiversity, ecosystem services, and human well-being: three challenges for designing research for sustainability. Current Opinion in Environmental Sustainability, 14: 76–85.
Bennett EM, Peterson GD, and Gordon LJ. 2009. Understanding relationships among multiple ecosystem services. Ecology Letters, 12: 1394–1404.
Boerema A, Rebelo AJ, Bodi MB, Esler KJ, and Meire P. 2017. Are ecosystem services adequately quantified? Journal of Applied Ecology, 54: 358–370.
Boesing AL, Prist PR, Barreto J, Hohlenwerger C, Maron M, Rhodes JR, et al. 2020. Ecosystem services at risk: Integrating spatiotemporal dynamics of supply and demand to promote long-term provision. One Earth, 3: 704–713.
Butchart SHM, Scharlemann JPW, Evans MI, Quader S, Aricò S, Arinaitwe J, et al. 2012. Protecting important sites for biodiversity contributes to meeting global conservation targets. PloS ONE, 7: e32529.
Canavire-Bacarreza G, and Hanauer MM. 2013. Estimating the impacts of bolivia’s protected areas on poverty. World Development, 41: 265–285.
Castro AJ, Martín-López B, López E, Plieninger T, Alcaraz-Segura D, Vaughn CC, and Cabello J. 2015. Do protected areas networks ensure the supply of ecosystem services? Spatial patterns of two nature reserve systems in semi-arid Spain. Applied Geography, 60: 1–9.
Chan KMA, Shaw MR, Cameron DR, Underwood EC, and Daily GC. 2006. Conservation planning for ecosystem services. PLOS Biology, 4: 2138–2152.
Chaplin-Kramer R, Sharp RP, Weil C, Bennett EM, Pascual U, Arkema KK. et al. 2019. Global modeling of nature’s contributions to people. Science, 366: 255.
Chazdon RL. 2008. Beyond deforestation: restoring forests and ecosystem services on degraded lands. Science, 320: 1458.
Ciccarese L, Mattsson A, and Pettenella D. 2012. Ecosystem services from forest restoration: thinking ahead. New Forests, 43: 543–560.
Cimon-Morin J, Darveau M, and Poulin M. 2013. Fostering synergies between ecosystem services and biodiversity in conservation planning: A review. Biological Conservation, 166: 144–154.
Cimon-Morin J, Darveau M, and Poulin M. 2014. Towards systematic conservation planning adapted to the local flow of ecosystem services. Global Ecology and Conservation, 2: 11–23.
Cimon-Morin J, Darveau M, and Poulin M. 2016a. Consequences of delaying conservation of ecosystem services in remote landscapes prone to natural resource exploitation. Landscape Ecology, 31: 825–842.
Cimon-Morin J, Darveau M, and Poulin M. 2016b. Site complementarity between biodiversity and ecosystem services in conservation planning of sparsely-populated regions. Environmental Conservation, 43: 56–68.
Cimon-Morin J, Darveau M, and Poulin M. 2018. Conservation Biogeography of Ecosystem Services. In Encyclopedia of the Anthropocene. Edited by DA Dellasala and MI Goldstein. Elsevier. pp. 25–30.
Cimon-Morin J, and Poulin M. 2018. Setting conservation priorities in cities: approaches, targets and planning units adapted to wetland biodiversity and ecosystem services. Landscape Ecology, 33: 1975–1995.
Cole LJ, Stockan J, and Helliwell R. 2020. Managing riparian buffer strips to optimise ecosystem services: A review. Agriculture, Ecosystems and Environment, 296: 106891.
Daw TIM, Brown K, Rosendo S, and Pomeroy R. 2011. Applying the ecosystem services concept to poverty alleviation: the need to disaggregate human well-being. Environmental Conservation, 38: 370–379.
de Groot RS, Alkemade R, Braat L, Hein L, and Willemen L. 2010. Challenges in integrating the concept of ecosystem services and values in landscape planning, management and decision making. Ecological Complexity, 7: 260–272.
Doerr VAJ, Barrett T, and Doerr ED. 2011. Connectivity, dispersal behaviour and conservation under climate change: a response to Hodgson et al. Journal of Applied Ecology, 48: 143–147.
Drupp MA. 2018. Limits to substitution between ecosystem services and manufactured goods and implications for social discounting. Environmental and Resource Economics, 69: 135–158.
Duraiappah AK. 2011. Ecosystem services and human well-being: do global findings make any sense? BioScience, 61: 7–8.
Durán AP, Casalegno S, Marquet PA, and Gaston KJ. 2013. Representation of ecosystem services by terrestrial protected areas: Chile as a case study. PloS ONE, 8: e82643.
Edenius L, and Mikusinski G. 2006. Utility of habitat suitability models as biodiversity assessment tools in forest management. Scandinavian Journal of Forest Research, 21: 62–72.
Egoh B, Rouget M, Reyers B, Knight AT, Cowling RM, van Jaarsveld AS, and Welz A. 2007. Integrating ecosystem services into conservation assessments: A review. Ecological Economics, 63: 714–721.
Eigenbrod F, Anderson BJ, Armsworth PR, Heinemeyer A, Gillings S, Roy DB, et al. 2010. Representation of ecosystem services by tiered conservation strategies. Conservation Letters, 3: 184–191.
Eigenbrod F, Anderson BJ, Armsworth PR, Heinemeyer A, Jackson SF, Parnell M. et al. 2009. Ecosystem service benefits of contrasting conservation strategies in a human-dominated region. Proceedings of the Royal Society B: Biological Sciences, 276: 2903–2911.
Fenichel EP, and Zhao J. 2015. Sustainability and Substitutability. Bulletin of Mathematical Biology, 77: 348–367.
Ferraro PJ, and Hanauer MM. 2014. Quantifying causal mechanisms to determine how protected areas affect poverty through changes in ecosystem services and infrastructure. Proceedings of the National Academy of Sciences, 111: 4332.
Ferraro PJ, Hanauer MM, and Sims KRE. 2011. Conditions associated with protected area success in conservation and poverty reduction. Proceedings of the National Academy of Sciences, 108: 13913.
Foley JA, DeFries R, Asner GP, Barford C, Bonan G, Carpenter SR. et al. 2005. Global consequences of land use. Science, 309: 570.
García Márquez JR, Krueger T, Páez CA, Ruiz-Agudelo CA, Bejarano P, Muto T, and Arjona F. 2017. Effectiveness of conservation areas for protecting biodiversity and ecosystem services: a multi-criteria approach. International Journal of Biodiversity Science, Ecosystem Services and Management, 13: 1–13.
Gollier C. 2019. Valuation of natural capital under uncertain substitutability. Journal of Environmental Economics and Management, 94: 54–66.
Gouvernement du Québec. 2016. Décret de population pour 2017—Municipalite´s locales, arrondissements, villages nordiques et territoires non organise´s. Gazette officielle du Québec, 28 décembre 2016 Décret 1099–2016, 21 décembre 2016. [online]: Available from mamrot.gouv.qc.ca/organisation-municipale/decret-de-population/.
Goyette J-O, Cimon-Morin J, Mendes P, Pellerin S, and Poulin M. 2021. Planning wetland protection and restoration for the safeguard of ecosystem service flows to beneficiaries. Landscape Ecology, 36: 2691–2706.
Grima N, Fisher B, Ricketts TH, and Sonter LJ. 2019. Who benefits from ecosystem services? Analysing recreational moose hunting in Vermont, USA. Oryx, 53: 707–715.
Guerry AD, Polasky S, Lubchenco J, Chaplin-Kramer R, Daily GC, Griffin R, et al. 2015. Natural capital and ecosystem services informing decisions: From promise to practice. Proceedings of the National Academy of Sciences, 112: 7348.
Hanna DEL, Raudsepp-Hearne C, and Bennett EM. 2020. Effects of land use, cover, and protection on stream and riparian ecosystem services and biodiversity. Conservation Biology, 34: 244–255.
Hodder KH, Newton AC, Cantarello E, and Perrella L. 2014. Does landscape-scale conservation management enhance the provision of ecosystem services? International Journal of Biodiversity Science, Ecosystem Services and Management, 10: 71–83.
Honey-Rosés J., Schneider DW, and Brozović N. 2014. Changing ecosystem service values following technological change. Environmental Management, 53: 1146–1157.
Juutinen A, Saarimaa M, Ojanen P, Sarkkola S, Haara A, Karhu J, et al. 2019. Trade-offs between economic returns, biodiversity, and ecosystem services in the selection of energy peat production sites. Ecosystem Services, 40: 101027.
Kleemann J, Schröter M, Bagstad KJ, Kuhlicke C, Kastner T, Fridman D, et al. 2020. Quantifying interregional flows of multiple ecosystem services – A case study for Germany. Global Environmental Change, 61: 102051.
Kontogianni A, Luck GW, and Skourtos M. 2010. Valuing ecosystem services on the basis of service-providing units: A potential approach to address the ‘endpoint problem’ and improve stated preference methods. Ecological Economics, 69: 1479–1487.
Kukkala A S, and Moilanen A. 2017. Ecosystem services and connectivity in spatial conservation prioritization. Landscape Ecology, 32: 5–14.
Law EA, Bryan BA, Meijaard E, Mallawaarachchi T, Struebig MJ, Watts ME, and Wilson K. A. 2017. Mixed policies give more options in multifunctional tropical forest landscapes. Journal of Applied Ecology, 54: 51–60.
Le Saout S, Hoffmann M, Shi Y, Hughes A, Bernard C, Brooks TM, et al. 2013. Protected areas and effective biodiversity conservation. Science, 342: 803.
Lecina-Diaz J, Alvarez A, De Cáceres M, Herrando S, Vayreda J, and Retana J. 2019. Are protected areas preserving ecosystem services and biodiversity? Insights from Mediterranean forests and shrublands. Landscape Ecology, 34: 2307–2321.
Lewis E, MacSharry B, Juffe-Bignoli D, Harris N, Burrows G, Kingston N, and Burgess ND. 2019. Dynamics in the global protected-area estate since 2004. Conservation Biology, 33: 570–579.
Lindenmayer DB, and Noss RF. 2006. Salvage logging, ecosystem processes, and biodiversity conservation. Conservation Biology, 20: 949–958.
Lira PK, de Souza Leite M, and Metzger JP. 2019. Temporal Lag in Ecological Responses to Landscape Change: Where Are We Now? Current Landscape Ecology Reports, 4: 70–82.
Lonsdorf E, Kremen C, Ricketts T, Winfree R, Williams N, and Greenleaf S. 2009. Modelling pollination services across agricultural landscapes. Annals of botany, 103: 1589–1600.
Luck G, Chan K, and Klien C. 2012. Identifying spatial priorities for protecting ecosystem services. F1000Research, 1.
Luck GW, Harrington R, Harrison PA, Kremen C, Berry PM, Bugter R, et al. 2009. Quantifying the contribution of organisms to the provision of ecosystem services. BioScience, 59: 223–235.
Mander Ü, Helming K, and Wiggering H. 2007. Multifunctional land use: meeting future demands for landscape goods and services. In Ü. Mander, H. Wiggering, and K. Helming. Multifunctional land use: Meeting future demands for landscape goods and services. Springer Berlin Heidelberg, Berlin, Heidelberg. pp 1–13.
Mandle L, Tallis H, Sotomayor L, and Vogl AL. 2015. Who loses? Tracking ecosystem service redistribution from road development and mitigation in the Peruvian Amazon. Frontiers in Ecology and the Environment, 13: 309–315.
Manhães AP, Mazzochini GG, Ary Oliveira-Filho T., Ganade G, and Carvalho AR. 2016. Spatial associations of ecosystem services and biodiversity as a baseline for systematic conservation planning. Diversity and Distributions, 22: 932–943.
Margules C, and Sarkar S. 2007. Systematic Conservation Planning. Ecology, Biodiversity and Conservation. Cambridge University Press, Cambridge, UK.
Margules CR, and Pressey RL. 2000. Systematic conservation planning. Nature, 405: 243–253.
Martínez-Harms MJ, and Balvanera P. 2012. Methods for mapping ecosystem service supply: a review. International Journal of Biodiversity Science, Ecosystem Services and Management, 80: 17–25.
McIntosh EJ, McKinnon MC, Pressey RL, and Grenyer R. 2016. What is the extent and distribution of evidence on effectiveness of systematic conservation planning around the globe? A systematic map protocol. Environmental Evidence, 5: 15.
Metzger JP, Fidelman P, Sattler C, Schröter B, Maron M, Eigenbrod F, et al. 2021. Connecting governance interventions to ecosystem services provision: A social-ecological network approach. People and Nature, 3: 266–280.
Millennium Ecosystem Assessment. 2005. Ecosystems and Human Well-being: Synthesis. Island Press.
Miller JR., and Hobbs RJ. 2007. Habitat restoration—Do we know what we’re doing? Restoration Ecology, 15: 382–390.
Minnes S, Gaspard V, Loring PA, Baulch H, and Breen S-P. 2020. Transforming conflict over natural resources: A socio-ecological systems analysis of agricultural drainage. FACETS, 5(1). This issue.
Mitchell MGE, Schuster R, Jacob AL, Hanna DEL, Dallaire CO, Raudsepp-Hearne C, et al. 2021. Identifying key ecosystem service providing areas to inform national-scale conservation planning. Environmental Research Letters 16: 014038.
Moreno-Mateos D, Barbier EB, Jones PC, Jones HP, Aronson J, Lopez-Lopez JA, et al. 2017. Anthropogenic ecosystem disturbance and the recovery debt. Nature Communications, 8: 1–6.
Moreno-Mateos D, Power ME, Comín FA, and Yockteng R. 2012. Structural and functional loss in restored wetland ecosystems. PLOS Biology, 10: e1001247.
Myers SS, Gaffikin L, Golden CD, Ostfeld RS, Redford KH, Ricketts TH, et al. 2013. Human health impacts of ecosystem alteration. Proceedings of the National Academy of Sciences of the United States of America, 110: 18753–18760.
Naidoo R, Balmford A, Ferraro PJ, Polasky S, Ricketts TH, and Rouget M. 2006. Integrating economic costs into conservation planning. Trends Ecol Evol, 21: 681–687.
Naughton-Treves L, Alix-Garcia J, and Chapman CA. 2011. Lessons about parks and poverty from a decade of forest loss and economic growth around Kibale National Park, Uganda. Proceedings of the National Academy of Sciences, 108: 13919.
Neugarten RA, Moull K, Martinez NA, Andriamaro L, Bernard C, Bonham C, et al. 2020. Trends in protected area representation of biodiversity and ecosystem services in five tropical countries. Ecosystem Services, 42: 101078.
Nhancale BA, and Smith RJ. 2011. The influence of planning unit characteristics on the efficiency and spatial pattern of systematic conservation planning assessments. Biodiversity and Conservation, 20: 1821–1835.
Perennes M, Diekötter T, Groß J, and Burkhard B. 2021. A hierarchical framework for mapping pollination ecosystem service potential at the local scale. Ecological Modelling, 444: 109484.
Pohjanmies T, Triviño M, Le Tortorec E, Mazziotta A, Snäll T, and Mönkkönen M. 2017. Impacts of forestry on boreal forests: An ecosystem services perspective. Ambio, 46: 743–755.
Polasky S, Nelson E, Lonsdorf E, Fackler P, and Starfield A. 2005. Conserving species in a working landscape: Land use with biological and economic objectives. Ecological Applications 15: 1387–1401.
Possingham HP, Bode M, and Klein CJ. 2015. Optimal Conservation Outcomes Require Both Restoration and Protection. PLOS Biology, 13: e1002052.
Pressey RL, and Bottrill MC. 2008. Opportunism, threats, and the evolution of systematic conservation planning. Conservation Biology, 22: 1340–1345.
Raudsepp-Hearne C, Peterson GD, Tengö M, Bennett EM, Holland T, Benessaiah K, et al. 2010. Untangling the environmentalist’s paradox: Why is human well-being increasing as ecosystem services degrade? BioScience, 60: 576–589.
Resende FM, Cimon-Morin J, Poulin M, Meyer L, Joner DC, and Loyola R. 2021. The importance of protected areas and Indigenous lands in securing ecosystem services and biodiversity in the Cerrado. Ecosystem Services, 49: 101282.
Rieb JT, Chaplin-Kramer R, Daily GC, Armsworth PR, Böhning-Gaese K, Bonn A, et al. 2017. When, where, and how nature matters for ecosystem services: challenges for the next generation of ecosystem service models. BioScience, 67: 820–833.
Rodrigues ASL, Andelman SJ, Bakarr MI, Boitani L, Brooks TM, Cowling RM, et al. 2004. Effectiveness of the global protected area network in representing species diversity. Nature, 428: 640–643.
Sarkar S, and Illoldi-Rangel P. 2010. Systematic conservation planning: an updated protocol. Natureza and Conservacao, 8: 19–26.
Schröter M, Barton DN, Remme RP, and Hein L. 2014. Accounting for capacity and flow of ecosystem services: A conceptual model and a case study for Telemark, Norway. Ecological Indicators, 36: 539–551.
Schröter M, Koellner T, Alkemade R, Arnhold S, Bagstad KJ, Erb K-H, et al. 2018. Interregional flows of ecosystem services: Concepts, typology and four cases. Ecosystem Services, 31: 231–241.
Schwartz MW, Cook CN, Pressey RL, Pullin AS, Runge MC, Salafsky N, et al. 2018. Decision support frameworks and tools for conservation. Conservation Letters, 11: e12385.
Seppelt R, Lautenbach S, and Volk M. 2013. Identifying trade-offs between ecosystem services, land use, and biodiversity: A plea for combining scenario analysis and optimization on different spatial scales. Current Opinion in Environmental Sustainability, 5: 458–463.
Serna-Chavez HM, Schulp CJE, van Bodegom PM, Bouten W, Verburg PH, and Davidson MD. 2014. A quantitative framework for assessing spatial flows of ecosystem services. Ecological Indicators, 39: 24–33.
Sherren K, Ellis K, Guimond JA, Kurylyk B, LeRoux N, Lundholm J, et al. 2020. Understanding multifunctional Bay of Fundy dykelands and tidal wetlands using ecosystem services – a baseline. FACETS, This issue.
Shifley SR, Thompson FR, Dijak WD, Larson MA, and Millspaugh JJ. 2006. Simulated effects of forest management alternatives on landscape structure and habitat suitability in the Midwestern United States. Forest Ecology and Management, 229: 361–377.
Summers JK, Smith, LM, Case JL, and Linthurst RA. 2012. A review of the elements of human well-being with an emphasis on the contribution of ecosystem services. Ambio, 41: 327–340.
Syrbe R-U, and Walz U. 2012. Spatial indicators for the assessment of ecosystem services: Providing, benefiting and connecting areas and landscape metrics. Ecological Indicators, 21: 80–88.
Tallis H, Kennedy CM, Ruckelshaus M, Goldstein J, and Kiesecker JM. 2015. Mitigation for one & all: An integrated framework for mitigation of development impacts on biodiversity and ecosystem services. Environmental Impact Assessment Review, 55: 21–34.
United Nations. 2019. World Population Prospects 2019 – Data Booklet. Department of Economic and Social Affairs, Population Division.
Verhagen W, Kukkala AS, Moilanen A, van Teeffelen AJA, and Verburg PH. 2017. Use of demand for and spatial flow of ecosystem services to identify priority areas. Conservation Biology, 31: 860–871.
Villamagna A, and Giesecke C. 2014. Adapting human well-being frameworks for ecosystem service assessments across diverse landscapes. Ecology and Society, 19(1): 11.
Villamagna AM, Angermeier PL, and Bennett EM. 2013. Capacity, pressure, demand, and flow: A conceptual framework for analyzing ecosystem service provision and delivery. Ecological Complexity, 15: 114–121.
Villarreal-Rosas J, Sonter LJ, Runting RK, Lopez-Cubillos S, Dade MC, Possingham HP, and Rhodes JR. 2020. Advancing Systematic Conservation Planning for Ecosystem Services. Trends in Ecology and Evolution, 35: 1129–1139.
Wallington TJ, Hobbs RJ, and Moore SA. 2005. Implications of current ecological thinking for biodiversity conservation: a review of the salient issues. Ecology and Society, 10(1): 16.
Wang Y, Yang F, Hua C, Hu J, Wang J, Zhou J, et al. 2021. Comparing the efficiencies of individual-scoring and complementarity-based algorithms in prioritizing existing protected areas. Ecological Indicators, 125: 107539.
Watson KB, Galford GL, Sonter LJ, Koh I, and Ricketts TH. 2019. Effects of human demand on conservation planning for biodiversity and ecosystem services. Conservation Biology, 33: 942–952.
Watts ME, Ball IR, Stewart RS, Klein CJ, Wilson K, Steinback C, et al. 2009. Marxan with Zones: Software for optimal conservation based land- and sea-use zoning. Environmental Modelling and Software, 24: 1513–1521.
Wei F, Wang S, Fu B, and Liu Y. 2020. Representation of biodiversity and ecosystem services in East Africa’s protected area network. Ambio, 49: 245–257.
Wilson KA, Meijaard E, Drummond S, Grantham HS, Boitani L, Catullo G, et al. 2010. Conserving biodiversity in production landscapes. Ecological Applications, 20: 1721–1732.
Winkler KJ, Dade MC, and Rieb JT. 2021. Mismatches in the ecosystem services literature—a review of spatial, temporal, and functional-conceptual mismatches. Current Landscape Ecology Reports, 6: 23–34
Wohl E, Lane SN, and Wilcox AC. 2015. The science and practice of river restoration. Water Resources Research, 51: 5974–5997.
Wolff S, Schulp CJE, and Verburg PH. 2015. Mapping ecosystem services demand: A review of current research and future perspectives. Ecological Indicators, 55: 159–171.
Wu J. 2013. Landscape sustainability science: Ecosystem services and human well-being in changing landscapes. Landscape Ecology, 28: 999–1023.
Xu W, Xiao Y, Zhang J, Yang W, Zhang L, Hull V, et al. 2017. Strengthening protected areas for biodiversity and ecosystem services in China. Proceedings of the National Academy of Sciences, 114: 1601.
Ziter C. 2016. The biodiversity–ecosystem service relationship in urban areas: A quantitative review. Oikos, 125: 761–768.
Supplementary material
Supplementary Material 1 (DOCX / 172 KB)
- Download
- 172.16 KB
Information & Authors
Information
Published In
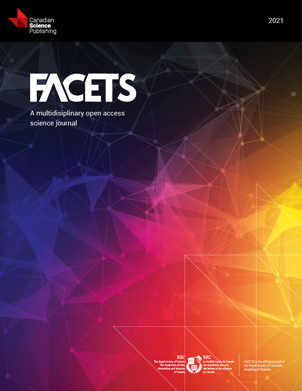
FACETS
Volume 6 • Number 1 • January 2021
Pages: 1570 - 1600
Editor: Evan Fraser
History
Received: 4 November 2020
Accepted: 20 June 2021
Version of record online: 16 September 2021
Notes
This paper is part of a Collection titled “Food, Fiber, Fuel, and Function: Pathways to manage ecosystem services in Canada’s working landscapes”.
Copyright
© 2021 Cimon-Morin et al. This work is licensed under a Creative Commons Attribution 4.0 International License (CC BY 4.0), which permits unrestricted use, distribution, and reproduction in any medium, provided the original author(s) and source are credited.
Data Availability Statement
All relevant data are within the paper and in the Supplementary Material.
Key Words
Sections
Subjects
Authors
Author Contributions
JC-M, SP, and MP conceived and designed the study.
JC-M, J-OG, and PM performed the experiments/collected the data.
JC-M, J-OG, and PM analyzed and interpreted the data.
JC-M and MP contributed resources.
All drafted or revised the manuscript.
Competing Interests
The authors have declared that no competing interests exist.
Metrics & Citations
Metrics
Other Metrics
Citations
Cite As
Jérôme Cimon-Morin, Jean-Olivier Goyette, Poliana Mendes, Stéphanie Pellerin, and Monique Poulin. 2021. A systematic conservation planning approach to maintaining ecosystem service provision in working landscapes. FACETS.
6(): 1570-1600. https://doi.org/10.1139/facets-2020-0100
Export Citations
If you have the appropriate software installed, you can download article citation data to the citation manager of your choice. Simply select your manager software from the list below and click Download.
Cited by
1. Tradeoffs and synergies in wetland multifunctionality: A scaling issue
2. Managing Canada’s land- and seascapes for multiple ecosystem services in the Anthropocene: introduction to the Food, Fiber, Fuel, and Function collection
3. Facing the challenges of using place-based social-ecological research to support ecosystem service governance at multiple scales