Community-led non-invasive polar bear monitoring in the Eeyou Marine Region of James Bay, Canada: insights on distribution and body condition during the ice-free season
Abstract
Wildlife conservation is informed by detailed understanding of species demographics, habitat use, and interactions with environmental drivers. Challenges to collecting this information, particularly in remote places and on widely ranging species, can contribute to data deficiencies that detract from conservation status assessment and the effectiveness of management actions. Polar bears in James Bay face rapidly changing environmental conditions at the southern edge of their global range, but studying their ecology has been limited by community concerns about the methods typically used in polar bear research. Using a community-led and non-invasive approach, we deployed hair snare and camera trap sampling stations across 400 km of the Eeyou Marine Region in eastern James Bay. Stations collected >100 hair samples and thousands of photographs in one eight-week period that allowed for a novel investigation of this population’s distribution and body condition during the ice-free season. Polar bears were in average to above average body condition, and model selection of detections at stations revealed distance to mainland as a significant predictor of polar bear presence. Given its high potential, we suggest community-based monitoring using this method become a standard protocol to expand the scope and local leadership of polar bear research across the North.
Graphical Abstract

Introduction
Conservation of wildlife populations is informed by detailed information on demographic parameters such as population size, age structure, sex ratio, birth and death rates, as well as information on movement patterns, habitat use, and interactions with environmental drivers. For many wildlife populations, however, the available quantitative information is insufficient to ensure effective management and conservation (IUCN 2019). Thorough wildlife monitoring over large spatiotemporal scales is logistically challenging and can be prohibitively expensive, leaving many wildlife populations un- or under-studied (Gompper et al. 2006). This is especially true in remote places that are likely to provide critical key wildlife habitat but where low human density and lack of infrastructure can make it challenging to assess and quantify their habitat value. The Arctic and subarctic are examples of such systems where remote locations and high costs of field work are widely recognized barriers to acquiring necessary data required for well-informed conservation planning (Mallory et al. 2018).
Historically, wildlife research in remote places has been centered around short periods of intense sampling where scientists travel to field sites to conduct surveys, live-capture animals, and deploy biologging devices (Gearheard and Shirley 2007; Provencher et al. 2013; Barber et al. 2014). This provides highly detailed data across the spatial and temporal extents defined by the study design that would be difficult or impossible to obtain otherwise. However, these approaches require expensive equipment and complex logistics (Laforge et al. 2017), typically involve invasive and disruptive research methods (Jewell 2013), limit field research involvement primarily to those deploying the devices, and provide few opportunities for community participation in the research process (Wong et al. 2020). Concerns about the ethics of chemical immobilization and handling animals for research purposes are also often raised by Indigenous communities. Development and use of new methods responsive to these concerns can contribute to respectful collaboration while also ensuring continuity and improvement in the collection of information needed to support conservation (Semple et al. 2000; Lunn et al. 2010; Peacock et al. 2011; Wong et al. 2020).
Community-led research focuses on locally prioritized questions using locally accepted methods in a manner that contributes to knowledge co-production and weaves different perspectives, knowledges, and experiences together to advance understanding and inform shared priorities (Berkes 2009; Ban et al. 2018). Non-invasive monitoring methods, including the use of camera traps (Gysel & Davis Jr. 1956), snow tracking (Erlinge 1967), drone surveys (Jagielski et al. 2022), hair snares (Woods et al. 1999), scat sampling (Litvaitis 2000), and harvest-based monitoring (Bell and Harwood 2012) are well suited to address the challenge of studying wildlife while maximizing community involvement. Non-invasive methods provide opportunities to monitor animals at varied spatiotemporal scales with little impact on the studied individuals and can allow for the collection of large number of samples in a cost-efficient way (Gompper et al. 2006). These methods also facilitate the involvement of community members in gathering a wide array of ecological information as they generally use less specialized and more widely available field equipment than many invasive methods. Community-led research approaches often combine well with non-invasive methods as community expertise and on-the-ground field support enable the deployment of user-friendly passive sampling equipment across large spatiotemporal scales in remote places that would be challenging to access otherwise. Furthermore, non-invasive sampling methods facilitate the distribution of financial resources among many communities and individuals, rather than in support of a small, highly specialized survey team, in a manner that can enhance both local benefits and local support for wildlife research. Despite the many potential benefits of community-led non-invasive monitoring, such methods are still underutilized in Arctic and northern wildlife research.
Monitoring polar bear populations is a local and global conservation priority that can be advanced through community-led research with non-invasive methods. Polar bears are globally threatened by climate-related sea ice declines that reduce access to the platform required to acquire food and mates (Stirling and Derocher 1993). Polar bears are classified and managed as 19 subpopulations defined according to genetics, movement patterns, and site fidelity (Bethke et al. 1996; Paetkau et al. 1999; Mauritzen et al. 2002). Currently, 16 of 19 polar bear subpopulations are listed as “data deficient” with insufficient information available to evaluate long-term population changes by circumpolar monitoring bodies (Polar Bear Range States 2015; IUCN/SSC Polar Bear Specialist Group 2021). As climate change rapidly alters Arctic systems, there is a need for more recent, more widespread, and more locally informed data on polar bear subpopulation demographics, trophic ecology, and habitat use (Vongraven et al. 2012; Hamilton and Derocher 2019). Polar bear monitoring is typically conducted through mark–recapture or aerial surveys (Mauritzen et al. 2002; Stirling et al. 2004; Obbard et al. 2018) with accepted gaps of 10–15 years between survey periods (Hamilton and Derocher 2019). Community leadership and involvement in polar bear research has the potential to complement and improve traditional monitoring programs by contributing additional data, finer-scale information, and local knowledge.
Non-invasive hair sampling coupled with camera traps is a widely used method to study grizzly and black bear distribution and population structure in North America (Woods et al. 1999; Gardner et al. 2010; Lamb et al. 2016). However, only two studies have applied this approach to polar bear research. A first study used a hair snare to assess local use of polar bears at subsistence-hunted whale carcasses (Herreman and Peacock 2013) and a second initiated a capture–recapture population assessment in a region of the M’Clintock Channel subpopulation (Van Coeverden De Groot et al. 2013). Despite clear potential benefits for collecting population and diet information on polar bears, the method has not become more widely employed in the decade following these descriptions. Possible barriers to the widespread use of these methods are the vastness and inaccessibility of polar bear range, the time and local expertise needed for ice, water, and(or) land-based travel to sampling locations, and concerns about the quality and specificity of information derived from passive sampling techniques.
Eastern James Bay Cree communities, located in north-eastern Canada at the southern margins of Hudson Bay in the Eeyou Marine Region (EMR), have observed changing polar bear abundance and distribution in the last decade, raising questions about polar bear population size, diet, and habitat use in James Bay (EMRWB 2019; EMRWB, unpublished data). Polar bears are not traditionally harvested by communities in the EMR but are occasionally killed in defense of life. Increasing human–polar bear encounters and conflicts around hunting sites and camps are of high concern in this region (Laforest et al. 2018). In 2018 consultations, communities in the EMR identified studying polar bears as a research priority while specifying that wildlife research in the region be conducted using non-invasive methods that avoid live-capturing animals (EMRWB 2019). Polar bears in James Bay exist at the southern edge of global polar bear range and already face warming conditions expected for populations farther north (Intergovernmental Panel on Climate Change 2021). They are designated as part of the Southern Hudson Bay subpopulation management unit that is predicted to face future declines due to reduced body condition linked to climate change-related habitat loss (Obbard et al. 2015, 2016, 2018). Little information is available on James Bay polar bear ecology, and few field-based studies have taken place in the region, particularly in the EMR on the eastern coast, due to low polar bear densities compared to other areas in the Arctic, coastal terrain that is ill-suited to seeking and capturing individuals, and, most importantly, the ethical concerns of Cree communities regarding the chemical immobilization methods commonly used in polar bear research (EMRWB 2019). However, it has been suggested that the James Bay polar bears may form a genetically distinct population due to limited spatial overlap with Hudson Bay bears during the breeding season (Crompton et al. 2008; Viengkone et al. 2018), and further investigation of their ecology in collaboration with local wildlife management organizations is needed to ensure effective conservation.
To gather information on the coastal ecology of an understudied polar bear population and demonstrate how community-led, non-invasive monitoring can advance research in remote places, we launched a non-invasive research program using an array of hair snare and camera trap sampling stations deployed across 400 km of the EMR. The program was co-developed with the Cree communities of Waskaganish, Eastmain, Wemindji, and Chisasibi, and regional wildlife management organizations, the Eeyou Marine Region Wildlife Board (EMRWB) and Cree Trappers’ Association (CTA), to assess polar bear distribution and body condition in the EMR, gather hair samples for future investigation of polar bear population structure and trophic ecology, and to support opportunities for community members to lead polar bear research in the region. Here, we report on the success of community-led, non-invasive sampling applied to polar bear research and use data gathered in an initial field season to describe the summer distribution and body condition of polar bears in the EMR of eastern James Bay. We demonstrate that combining non-invasive sampling methods and community-led research provides a synergistic opportunity to co-develop knowledge and redistribute scientific resources to address both northern community and scientific conservation priorities.
Positionality and contributions
In this statement, we present the background, expertise, and contributions of the author team to share the context of this paper and its authorship with the reader. Our team consists of Indigenous and non-Indigenous wildlife scientists and land stewards who have come together to better understand polar bear ecology in the EMR of eastern James Bay. Four authors are Local Officers with the Eeyou Marine Region Department of the Cree Trappers’ Association (CTA-EMR), all of whom self-identify as Cree and Indigenous, and provide local support for wildlife data collection and monitoring in the four coastal Cree communities in eastern James Bay (Waskaganish: Sanford Diamond [SD], Eastmain: Derek Okimaw [DO], Wemindji: George Natawapineskum [GN], and Chisasibi: John Lameboy [JL]). Three authors (Angela Coxon [AC] the EMRWB Director, Stephanie Varty [SV], and Felix Boulanger [FB]) are wildlife biologists at the EMRWB, the Cree wildlife management organization in the EMR, an Institute of Public Government created under the Eeyou Marine Region Land Claims Agreement that is responsible for making wildlife management decisions, identifying research priorities, and supporting wildlife research in the EMR. Natasha Louttit [NL], who self-identifies as Cree and Indigenous, is the CTA-EMR Wildlife Liaison Officer and is responsible for the coordination of wildlife research and monitoring activities between the EMRWB and CTA-EMR Local Officers. Anderson Jolly [AJ] is the Cree tallyman responsible for land stewardship and wildlife monitoring on Charlton Island and has been observing polar bears in the region for the last six decades. Two authors are academic scholars: Murray Humphries [MH] is a professor and wildlife biologist with experience supporting collaborative research with communities in northern Canada and Alexandra Langwieder [AL] is a graduate student who has been building the project with this team in the EMR since June 2020.
All authors contributed knowledge, expertise, and effort that allowed this work to be possible. Local Cree Knowledge of the eastern James Bay system (AJ, SD, GN, JL, DO, NL), knowledge of polar bear ecology and conservation (all), and knowledge of non-invasive wildlife sampling methods (AL, MH) facilitated the launch of this research program and the work presented here. All authors contributed substantially to the study design and reviewing the manuscript. AL, GN, JL, DO, AJ, SD, FB and SV collected data in the field and coordinated fieldwork activities in each community. AL and MH led data analysis and manuscript writing, while AC, SV, and FB provided edits and confirmation of support for the research on behalf of the EMRWB. Securing project funding was led by MH, AL, and AC.
Methods
This project was developed with the EMRWB and CTA to address EMR community research priorities. The project and field sampling design described here were developed over the 12-month period between June 2020 and June 2021 and received formal support from the EMRWB, the CTA, the Chief and Council of the four communities involved (Waskaganish, Eastmain, Wemindji, and Chisasibi), as well as the Cree tallymen responsible for land management and traditional harvesting activities where the sampling stations were deployed. Hair sample collection methods were approved by McGill Animal Care Committee (Animal Use Protocol #2021-8217).
In June and July 2021, we deployed 37 sampling stations across a 400 km north–south gradient (53.008°N–54.000°N) of the EMR (Fig. 1). The EMR contains 1328 islands in James Bay ranging in area from <1 to 300 km2: 95% are <1 km2, 4% are 1–20 km2, and 1% are 20–300 km2 (Natural Resources Canada 2016). The largest 1% is limited to three islands: Charlton Island (315 km2), North Twin Island (158 km2), and South Twin Island (77 km2). Along south-to-north and nearshore-to-offshore gradients, vegetation communities transition from boreal forest in the south and nearshore to tundra and barren lands in the north and offshore. In James Bay, polar bears have access to Arctic-associated food sources, such as ringed seals (Pusa hispida), bearded seals (Erignathus barbatus), beluga (Delphinapterus leucas), caribou (Rangifer terrandus), and seabird colonies (e.g., Somateria mollissima and Sterna paradisaea) (Stewart and Lockhart 2005) but also to potential boreal food sources such as beaver (Castor canadensis), moose (Alces alces), black bear (Ursus americanus), and certain fish species (e.g., Coregonus artedi) (Humphries et al. 2019).
Fig. 1.
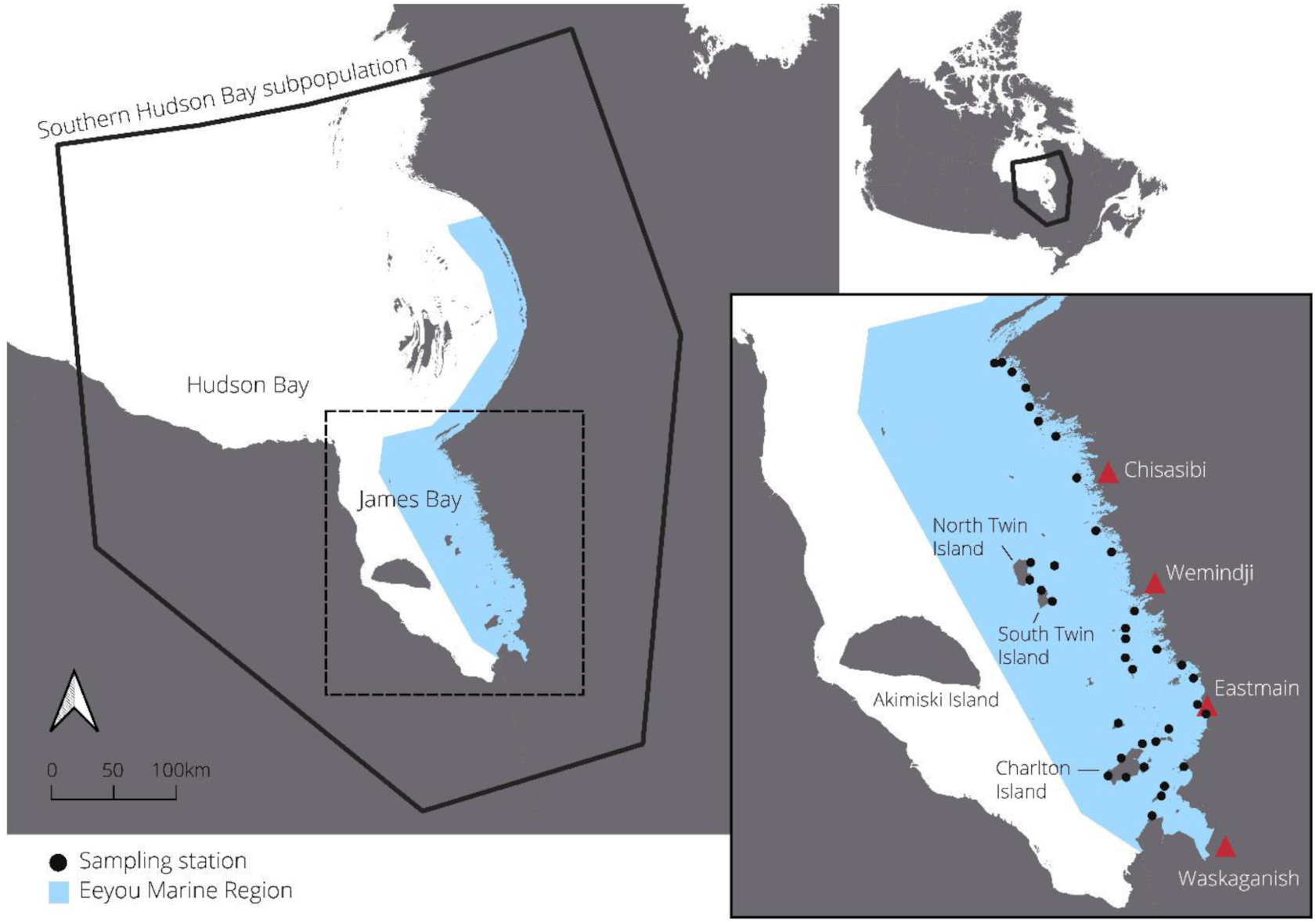
We deployed sampling stations on 29 offshore and nearshore islands and at 4 locations on the mainland. Mainland locations were not selected in Wemindji and Chisasibi territories because of high hunting camp densities and concerns about the proximity of sampling stations to places of human activity. All sites were selected with Cree experts based on past polar bear observations, distance from communities and camps, and accessibility by boat. We deployed sampling stations on islands of differing sizes and distances to shore while respecting the feasibility of accessing these remote places. To facilitate training and standardization among the four community field teams, we staggered station deployment over four weeks rather than attempt to deploy from each community simultaneously. We accessed sites with two boats captained by local land users and revisited the sites every two weeks to collect hair samples (Lamb et al. 2016), refresh the bait, and change camera trap memory cards. Weather prevented field teams from revisiting two stations deployed from Wemindji and two stations deployed from Waskaganish for a period of one month. Field teams surveyed islands for polar bears and evidence of polar bear dens while travelling on repeated boat paths to sampling stations. One or two observers scanned islands from each side of the boats and recorded polar bear locations and body condition as well as any observed den locations.
Polar bear hair samples were collected on barbed wire strung between posts around a scented bait, following a modified corral-style hair snare configuration described by Woods et al. (1999). We placed three steel t-posts in a 10 m triangle and pounded each post 0.6 m into the substrate (Fig. 2). We strung and tightened a single strand of 12-gauge barbed wire between the posts at 0.5 m from the ground. In the center of the triangle, we baited the snare using a 2:1 mixture of 188 mL of fryer grease with 95 mL fish-scented commercial black bear lure (Mixours, Ferme Monette Quebec) that we poured on rocks and covered with additional rocks to protect from weathering. The bait did not provide any food reward to the bears. We attached a camera trap at 0.75 m from the ground on a fourth t-post placed 2 m from the point of the triangle facing the water (Fig. 2). We used Reconyx HyperFire 2 Professional Covert IR Cameras protected by metal security enclosures (Reconyx Inc., Holmen, WI). Cameras were set to medium–high sensitivity and programmed to take three photos per trigger with no delay between photos. We positioned all stations on the shore and above the high tide line.
Fig. 2.
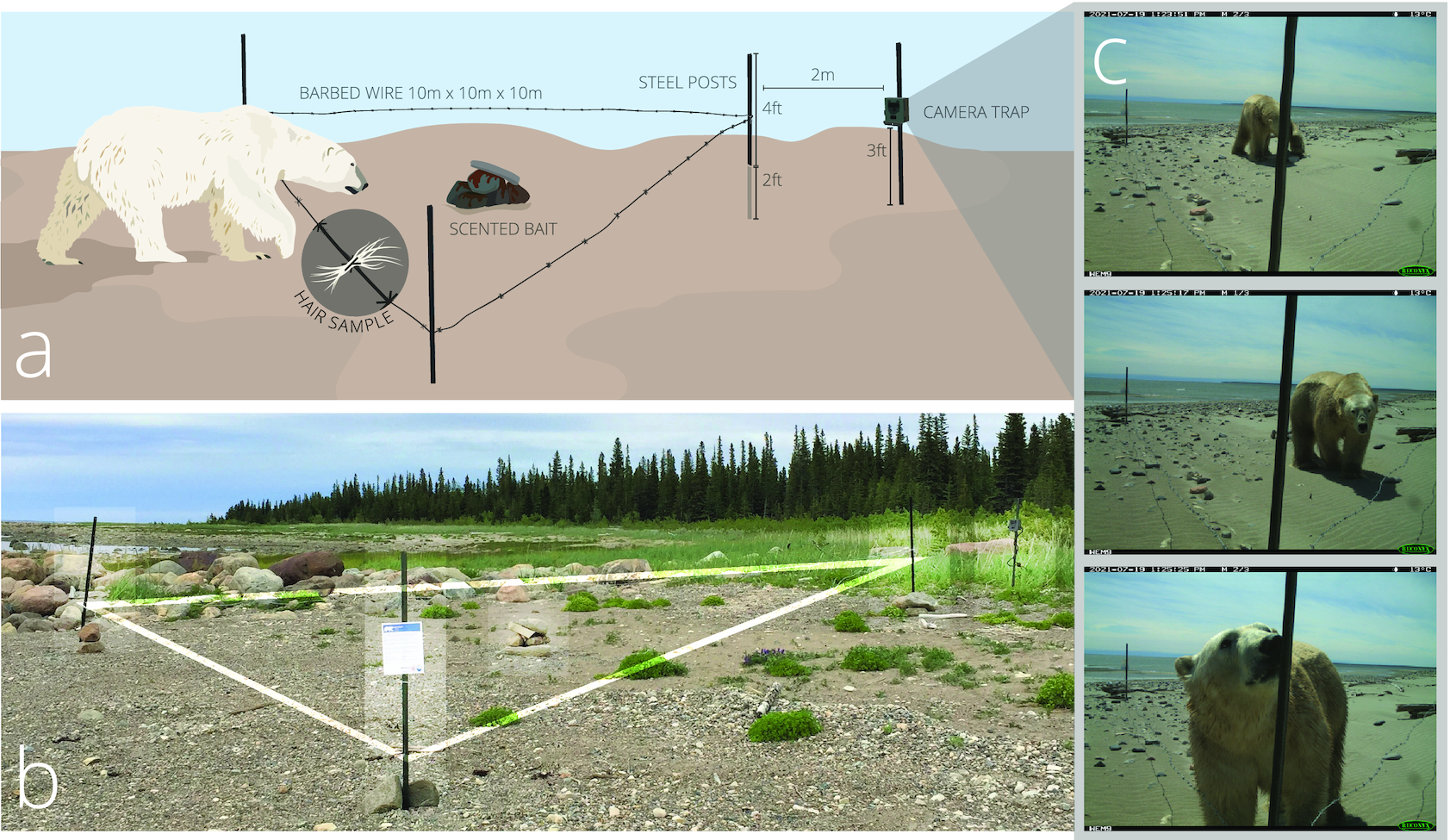
This study focused on methodological development for future community-based polar bear work, and genetic and stable isotope analyses of hair samples are not reported here. A detailed description of our sampling station design, including subsequent revisions and improvements to the design introduced after completion of the field work described here, is provided in Supplementary material.
Photos were classified as a new detection event when more than 15 min elapsed since the last polar bear photo unless distinct facial or body markings allowed us to identify the individual as a returning bear. To account for variation in station deployment duration, we divided the number of detections by the number of sampling weeks to generate a detections per week index of polar bear relative abundance.
To explore the influence of island characteristics on detection rate, we first characterized each island and mainland sampling location. We measured island size and distance to mainland using QGIS and classified land cover using Landsat land class data at 30 m resolution (Natural Resources Canada 2015). We combined land classes into six groups based on vegetation height: low (barren lands, lichens, and mosses), wet low (wetlands), low-intermediate (grasslands), intermediate vegetation (shrubland), high deciduous (deciduous and mixed forests), and high needleleaf (needleleaf forests). We then performed a Principal Component Analysis to synthesize those variables (R Core Team 2021). The resulting first principal component (PC1) explained 39% of the variation and was associated to vegetation height, ranging from a low PC1 score for barren land and moss to a high PC1 score for forests. The second principal component (PC2) explained 20% of the variation and was associated to low-to-intermediate vegetation cover type, with low PC2 scores corresponding to shrubland and high PC2 scores corresponding to grasslands (Supplementary material 1, Schlesinger et al. 1990; Alvarez et al. 2012). We used these two principal components (PC1 and PC2) as land cover variables.
Polar bear detections were analysed as a response variable, island ID as a random effect to account for multiple sampling stations deployed on three of the largest islands, and island size and distance, land cover, and latitude as fixed effects. We constructed eight a priori models and ranked them using Akaike’s Information Criterion (AIC), considering models with an AIC < 2 as equivalent (Burnham and Anderson 2002).
To evaluate body condition, a single observer (AL) scored bears using a standardized polar bear Fatness Index (FI) (Stirling et al. 1989; 2008) based on field observations and camera trap photos. Each polar bear was assigned an FI ranging from 1 to 5 with 1 representing the thinnest and 5 representing the fattest (Stirling et al. 1989; 2008). Based on body size inferred using posts and accompanying bears as a height reference, individuals were also assigned an approximate development age/size class: cub (dependent offspring, 0–2 years old), subadult (2–4 years old), adult (>4 years), or unknown. Individuals less than one-third the size of a typical adult bear were classified as cubs, individuals between one-third and two-thirds were considered subadults, and individuals over two-thirds were classified as adults. We did not attempt to sex bears from visual observations. Given sexual dimorphism in polar bears (Derocher et al. 2005), this size-based age estimate method is likely to misclassify some male subadults as adult bears and possibly some small adult females without cubs as subadults. Bear age class and body condition were classified as “unknown” when they were detected but only a small portion of the bear was visible in the photo or the bear was too far away to assess their size and condition.
Results
From 1 July 2021 to 31 August 2021, we recorded 108 polar bear camera trap observations (Fig. 3) and collected 118 hair samples. Bears were detected and hair samples collected at 14 of the 37 sampling stations, with the other 23 stations yielding no bear photos or hair samples. Sampling stations remained in good condition for the duration of the sampling period. After investigating the bait, bears interacted with each component of the sampling station by smelling the posts and wire and sometimes bending the posts. There was one incident of broken barbed wire during the sampling period. We did not find evidence in the field or with camera traps to suggest the wire interrupted the movements of polar bears or other wildlife that we detected. Once within the sampling station, polar bears had ample space to move freely, even with multiple individuals inside the station at the same time. Occasionally, polar bears disturbed camera traps after entering the station, and camera traps at the 14 stations with polar bear activity remained functional (upright and directed towards the hair sampling station) for 62% of sampling days on average. Minimum camera coverage was 8 days (19% of sampling period) and maximum was 45 days (100% of sampling period; see Supplementary material 2). In addition to polar bears, stations captured samples and photos of black bears (U. americanus), red foxes (Vulpes vulpes), wolves (Canis lupus), caribou (R. terrandus), and geese (Branta canadensis).
Fig. 3.
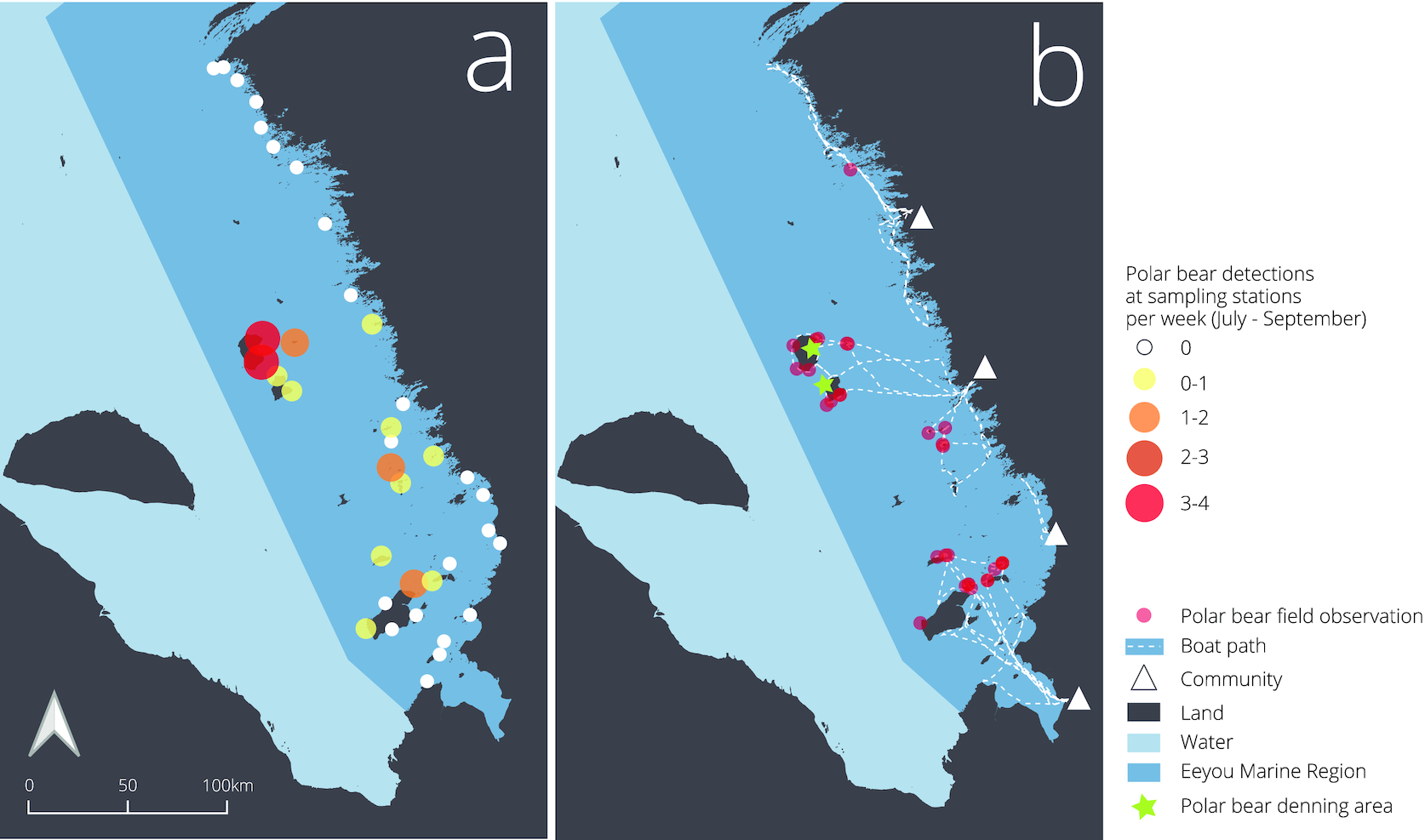
Field teams recorded 30 additional opportunistic polar bear observations during this period while travelling by boat to and from sampling stations (Fig. 3). Combining camera trap photos and field observations, we observed 94 occurrences of adults, 5 of subadults, 12 of cubs, and 27 bears of unknown age class. Among the 94 adult sightings, we observed 11 occurrences of females with cubs. Most observations (96%), both from camera traps and in the field, were of single bears. We were able to score polar bear body condition for 89 of 138 observations.
Most polar bear detections occurred at sampling stations far from the mainland with 2–4 times more detections on offshore islands than nearshore islands or mainland habitats (Fig. 4). Two large islands in the center of James Bay, North and South Twin Island, accounted for 43% of all sampling station observations (n = 47) and 30% of visual field observations (n = 9). Analyzing polar bear detections in relation to distance from mainland, island size, land cover (vegetation height and soil moisture), and latitude using candidate models revealed distance from the mainland as the only significant predictor (Table 1). Model selection resulted in two models, including distance to mainland being competitive, but the best-supported model was distance to mainland alone, with a second model that also included island size being less supported (Table 1). Using this model, we found the number of detections per week increased by 1 with each 30 km increase from the mainland (Fig. 4; β [95% CI] = 0.03 [0.02, 0.05]).
Fig. 4.

Table 1.
Model | K | AICc | ΔAICc | AICc Wt. |
---|---|---|---|---|
Distance from mainland | 4 | 73.62 | 0 | 0.68 |
Distance from mainland + size | 5 | 75.61 | 1.99 | 0.25 |
Distance from mainland + size + latitude | 6 | 78.4 | 4.77 | 0.06 |
Distance from mainland + size + latitude + land cover (PC1 + PC2) | 8 | 83.89 | 10.26 | 0 |
Null | 3 | 84.83 | 11.21 | 0 |
Size | 4 | 86.48 | 12.86 | 0 |
Latitude | 4 | 86.83 | 13.20 | 0 |
Land cover (PC1 + PC2) | 5 | 88.70 | 15.07 | 0 |
Field surveys revealed two polar bear denning areas on the Twin Islands. On North Twin Island, 19 dens were found along a 6 km span of gravel ridge on the northeast coast. Of these dens, six appeared to be recently used with fresh piles of sand and minimal vegetation growth at the entrance compared to overgrown and slumping dens that were likely older and not in use (see photos in Supplementary material 3). On South Twin Island, two dens were found on the northern coast but did not appear to be recently used.
Bears of all age classes were primarily in average to above-average body condition with 52% and 25% of classified observations scored as FI = 3 (average) and FI = 4 (fat), respectively (Fig. 5). Of 76 classified adult bear observations, 32% were scored FI = 4, 65% were scored FI = 3, and 4% were scored FI = 2 (thin). Of the classified subadult observations (n = 3), one was scored as FI = 4 and two as FI = 3. Of the classified cub observations (n = 10), six were scored FI = 4 and four were scored as FI = 3 (Fig. 5). Body condition did not appear to decline or increase over time during the July–August sampling period (see Supplementary materials).
Fig. 5.
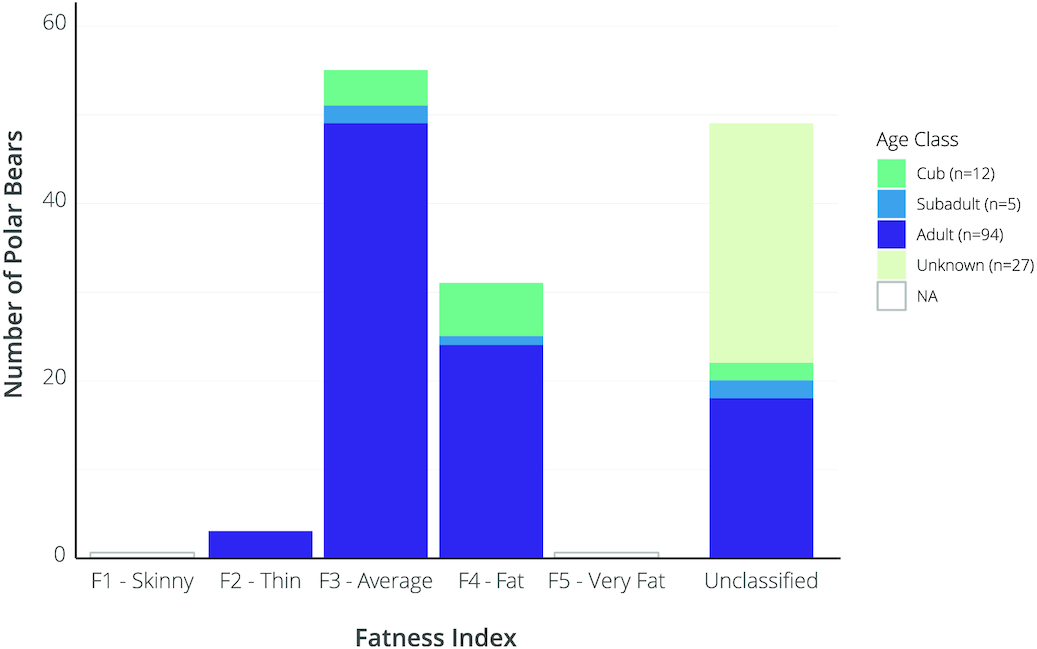
Discussion
Non-invasive methods such as hair snares and camera traps provide an opportunity for communities to co-develop and participate in polar bear research while adding to our understanding of polar bear ecology. In this study, we found that hair snare and camera trap sampling stations are effective community-led research tools for gathering data on polar bear space use and body condition. Stations successfully collected polar bear photos, which can be used to assess relative abundance, body condition, and size/age classes, and polar bear hair samples, which can provide information about population genetics (Herreman and Peacock 2013), diet (Boucher et al. 2019), contamination exposure (Lippold et al. 2022), and hormones (Bechshøft et al. 2013), all of which can be used to better inform conservation plans and efforts.
Polar bears have been the focus of intensive research efforts due to their urgent and precarious conservation status related to climate change-induced habitat loss, and ensuring that detailed information is current and available on all subpopulations is an ongoing priority of conservation advisory groups (COSEWIC 2018; IUCN/SSC Polar Bear Specialist Group 2021). However, polar bear field research is expensive and logistically challenging, particularly when it involves live capture, aerial surveys, or biopsy darting (Laforge et al. 2017), and while these approaches provide essential information about individuals and population status, they may be less conducive to the widespread participation and support of northern communities in research (Semple et al. 2000; Peacock et al. 2011). This can result in communities being excluded from and unsupportive of polar bear research, leading to data gaps in places where studies using unsupported methods cannot take place. For instance, research efforts in eastern James Bay have been limited due to ethical concerns regarding capture techniques (EMRWB 2019), and the government of Nunavut, a territory covering most of the Canadian Arctic, has withdrawn their support of invasive polar bear research methods except in certain circumstances (Government of Nunavut 2020). Non-invasive methods can also be expensive, but by including more community members in the research and avoiding the need to seek, capture, or handle bears, they may be better aligned with community support and priorities, leading to more opportunities for data collection and the participation of community members in project development, data collection, and result interpretation (Wong et al. 2020).
To our knowledge, two studies previously attempted to use hair snares for polar bear research. The first, by Herreman and Peacock (2013), examined local polar bear use of subsistence-hunted whale carcasses in AK by deploying one hair snare around whale carcasses and collecting samples weekly. A second study by Van Coeverden De Groot et al. (2013) deployed hair snares across a larger geographic area on the sea ice but had challenges related to sampling station stability and accessing stations. When sampling locations are accessible, hair snares can provide highly detailed information about polar bear genetics and foraging behaviour (Herreman and Peacock 2013). However, this can be difficult to achieve across large geographic scales in remote places where resources and infrastructure are often limited. The hair snare design we present here allowed for high sampling station stability and sampling efficiency across a large study area, with only one instance of wire falling. By centering Cree community knowledge and field expertise in the study design and field work, we successfully deployed 37 hair snare and camera trap sampling stations distributed across a large spatial scale (400 km south to north and 65 km coast to offshore, representing 26 000 km2 total area) to collect >100 polar bear hair samples and thousands of photographs in a single summer. While information from live capturing and deploying biologging equipment provides additional individual and multi-season information, we demonstrate that this approach facilitates broad population monitoring in a cost-effective way. We estimate field costs at approximately $60 000–$70 000 CAD with one-third of this directed to community member field support and one-third accounting for a one-time investment of materials (see cost summary in Supplementary material).
Determining polar bear distribution across space and time contributes to Cree land user safety and acts to reduce human–bear conflict, particularly at times of year when land users are active in James Bay. For communities, this fine-scale distribution information may be more locally relevant than more spatially extensive surveys. Consistent with past studies (Obbard et al. 2015; Laforest et al. 2018) and community consultations (EMRWB, unpublished report), we found evidence of more intensive use of offshore islands than nearshore or mainland habitats by polar bears in the EMR. Despite two large and distant offshore islands (North and South Twin Islands) accounting for a high proportion of polar bear observations, removing these islands from analyses did not eliminate distance from the coast as a strong predictor of polar bear detection rate. This distribution pattern could be related to the spatial progression of sea ice melt in James Bay that commences along the coast and then spreads offshore (Stewart and Lockhart 2005), possibly leading bears to follow the retreating ice to offshore islands before it disappears completely. Preferential use of offshore islands may also reflect greater availability of food resources, but assessing this possibility requires more information about summer diets and the distribution and accessibility of preferred summer food.
We did not find an effect of land cover type, latitude, or island size on polar bear detections at sampling stations in the EMR despite these characteristics being found to influence polar bear distribution in other systems (Stirling et al. 2004; Iverson et al. 2014; Rogers et al. 2015; Laidre et al. 2018). Age and sex class are influences on polar bear habitat selection (Derocher and Stirling 1990; Freitas et al. 2012), and while we did not have enough data on age and sex classes to perform analyses, we observed mothers with cubs concentrated around Charlton Island in the south of our study area and North Twin Island in the north. In western Hudson Bay, where polar bears are similarly confined to land for the ice-free season, different demographic groups select habitats based on land cover type, related to preferred den sites, temperature exposure, and possible food sources (Stirling et al. 2004). Polar bears in the Nunavut archipelago and Beaufort Sea prolong spring hunting at the ice floe edge into the summer season by moving north with the retreating ice (Rogers et al. 2015). Large islands may provide more resources through coastal scavenging opportunities, which has been found to be an important foraging behaviour for landfast bears (Iverson et al. 2014; Laidre et al. 2018).
Suitable maternal denning habitat is critical for polar bear populations (Linnell et al. 2000). Our observations of polar bear denning on the Twin Islands in 2021, together with earlier observations made by Doutt (1967), Obbard and Middel (2012), and Obbard et al. (2015, 2018), indicate the continued importance of the Twin Islands as maternal denning habitat. The presence of these dens in high concentrations along steep, east-facing banks is consistent with findings across the Arctic, where polar bears select denning habitat based on snow accumulation patterns in early winter (Durner et al. 2003; Derocher et al. 2011; Merkel et al. 2020). On the Twin Islands, prevailing northwesterly winds cause snow to accumulate on these eastern banks, providing suitable maternal denning habitat.
Body condition decline is among the first indicators of climate change-related habitat loss in polar bears and can play a determining role in future reproduction and survival (Stirling and Parkinson 2006). In James Bay, polar bears are constrained to land for four to five months as they wait for sea ice to freeze, exposing them to one of the longest ice-free fasting periods across polar bear range (Stern and Laidre 2016). The ice-free season in southern Hudson Bay and James Bay has increased by over 30 days from 1980 to 2012, and body condition in bears captured on the Ontario Hudson Bay coast has concurrently decreased (Obbard et al. 2016). These body condition declines have not yet been found to impact survival or population size (Obbard et al. 2015, but see Obbard et al. 2018). We found that polar bears observed in the EMR in July and August 2021 were characterized by average to above-average body condition, with three observations classified as thin. Polar bears lose up to 1 kg of mass per day of fasting (Pilfold et al. 2016), which, after one month, can be sufficient to shift an individual from one FI category to the category below (Stirling et al. 2008). We did not find evidence of body condition declining during our summer sampling period. According to regional ice analyses from the Canadian Ice Service (Environment and Climate Change Canada 2021), sea ice retreated from nearshore areas in the EMR in the week of 14 June 2021, followed by the offshore areas two weeks later, around 28 June. Ice remained along the northern shore of Akimiski Island until the week of 5 July. James Bay was then ice-free throughout the summer and autumn until ice reformed in the weeks of 22 November and 20 December for the nearshore and offshore areas, respectively. Thus, our July and August observations of polar bear body condition occurred 4–7 weeks after bears could have last been on the sea ice and when they had an additional 15–20 weeks on land until the ice reformed in the nearshore and offshore areas. Given bears were less than a third of the way through the ice-free season when we observed them, their relatively high body condition may not be reflective of body condition later in the ice-free season. Accordingly, observations later in the year and a better understanding of the diet and energy balance of James Bay polar bears during the ice-free season are key knowledge gaps required to better interpret and assess the potential demographic implications of the body condition observations reported here.
A study investigating polar bear summer diet on North and South Twin Islands found that birds, vegetation, and mammals are most represented in polar bear scat during the summer (58%, 32%, and 4% by volume, respectively), and it has been suggested by both researchers and Cree knowledge holders that the bears in eastern James Bay benefit from its turbid, shallow water that enables effective polar bear harvest of birds resting in shallow water during the ice-free season (Russell 1975; EMRWB, unpublished data). In western Hudson Bay, where high-quality terrestrial resources are similarly abundant to James Bay and where polar bears have been well documented foraging on these resources, survival rates and population size have decreased with increasing time spent on land (Regehr et al. 2007). It is unknown how access to coastal boreal species, such as beaver, black bear, and moose, which are absent from more northerly Arctic regions, might contribute to the diet and body condition of polar bears in James Bay. More information about summer diets and energy balance of polar bears during the ice-free season, in James Bay and elsewhere, will contribute to our understanding of the impacts of lengthening ice-free seasons on polar bear body condition and population viability.
Given we could not identify individuals in this study, the number of detections is likely to reflect a combination of unique individuals and repeated visits by the same individual. Accordingly, the number of detections is not representative of actual polar bear density. Bear movement between islands and coastal areas during the ice free season may be rare due to the high energetic costs of swimming (Pilfold et al. 2017; Griffen 2018), and incomplete detection of polar bears related to disturbed camera traps following a detection event may have led to fewer bears being detected than were present. Modifications to the camera and barbed wire attachment points combined with guy lines on t-posts supported by buried sandbags and height markers on t-posts are planned for subsequent field seasons (guide to modified design specified in the Supplementary material). Future steps in this research involve combining genetic information from hair samples with detections at sampling stations, which offer additional opportunities to estimate actual abundance and gather information about island fidelity and among-island movements. Nonetheless, results to date provide strong evidence that polar bear presence is not equally distributed across island or mainland habitats and indicate clear areas of high polar bear activity.
Non-invasive, community-led polar bear monitoring using hair snares and camera traps is a promising direction for polar bear field research that can complement ongoing research programs by providing opportunities for communities to be more directly involved in and responsible for collecting data. Given the relatively low cost and high potential of this method, we believe hair snare and camera trap sampling stations could become a standard sampling protocol in many locations that can be accessed from communities, camps, and research sites in a manner that could expand the coverage of polar bear monitoring across northern Canada and the circumpolar North. Assessing and improving the compatibility of these community-inclusive monitoring approaches with standard or next-generation mark–recapture approaches and population estimation techniques is an important research challenge requiring critical consideration and innovation. We found that polar bears in the EMR during the ice-free season were distributed on distant offshore islands more than on nearshore islands or the mainland and had average to above-average body condition. Future studies on the genetics, diet, and behaviour of polar bears in James Bay are needed to refine our understanding of the abundance, distribution, movements, and body condition of this uniquely southern polar bear population. As this and other subarctic and Arctic regions undergo rapid environmental change, sustained, non-invasive, community-led polar bear monitoring can provide critically needed genetic, diet, body condition, and habitat use information required for conserving this important marine predator.
Acknowledgements
We are grateful for the field teams in each community for their expertise and support in the field (Waskaganish: Harry Erless, Dinah Hester, Bernard Diamond, Stephanie Salt. Eastmain: Wilfred Cheezo and Russel Cheezo. Wemindji: Henry Stewart, Ernie Hughboy and Cody Mark. Chisasibi: Elmer Bobbish, Stephen Bobbish and Irvin Matches). We would also like to acknowledge the logistical support of Manuelle Landry-Cuerrier and Catherine Geoffroy. Thank you to Frederic Dulude-de Broin, Melanie Louise Leblanc, Catherine Geoffroy, and Manuelle Landry-Cuerrier for helpful comments on early drafts of this work. Gregory Thiemann and Martyn Obbard provided critical reviews that helped us improve an earlier version of this manuscript. This research was supported by the Eeyou Marine Region Wildlife Board Research Fund [grant #92785], the National Research and Engineering Council of Canada [grant #85940, 87751], Polar Knowledge Canada [grant #18897], Institut Nordique du Quebec [grant #87074], the Weston Family Foundation, and Ouranos Inc. [grant #79901].
References
Alvarez L.J., Epstein H.E., Li J., Okin G.S. 2012. Aeolian process effects on vegetation communities in an arid grassland ecosystem. Ecology and Evolution, 2: 809–821.
Ban N.C., Frid A., Reid M., Edgar B., Shaw D., Siwallace P. 2018. Incorporate Indigenous perspectives for impactful research and effective management. Nature Ecology and Evolution, 2: 1680–1683.
Barber P.H., Ablan-Lagman M.C.A., Ambariyanto A., Berlinck R.G.S., Cahyani D., Crandall E.D., et al. 2014. Advancing biodiversity research in developing countries: the need for changing paradigms. Bulletin of Marine Science, 90: 187–210.
Bechshøft T., Sonne C., Rigét F.F., Letcher R.J., Novak M.A., Henchey E., et al. 2013. Polar bear stress hormone cortisol fluctuates with the North Atlantic Oscillation climate index. Polar Biology, 36: 1525–1529.
Bell R.K., Harwood L.A. 2012. Harvest-based monitoring in the Inuvialuit Settlement Region: steps for success. Arctic, 65: 421–432.
Berkes F. 2009. Evolution of co-management: role of knowledge generation, bridging organizations and social learning. Journal of Environment Management, 90: 1692–1702.
Bethke R., Taylor M.K., Amstrup S.C., Messier F. 1996. Population delineation of polar bears using satellite collar data. Ecological Applications, 6: 311–317.
Boucher N.P., Derocher A.E., Richardson E.S. 2019. Space use patterns affect stable isotopes of polar bears (Ursus maritimus) in the Beaufort Sea. Polar Biology, 42: 1581–1593.
Burnham K., Anderson D. 2002. Model selection and multi-model inference: a practical information-theoretical approach. Springer, New York.
COSEWIC. 2018. COSEWIC assessment and status report on the polar bear (Ursus maritimus). Committee on the Status of Endangered Wildlife in Canada.
Crompton A.E., Obbard M.E., Petersen S.D., Wilson P.J. 2008. Population genetic structure in polar bears (Ursus maritimus) from Hudson Bay, Canada: implications of future climate change. Biological Conservation, 141: 2528–2539.
Derocher A.E., Stirling I. 1990. Distribution of polar bears (Ursus maritimus) during the ice-free period in western Hudson Bay. Canadian Journal of Zoology, 68: 1395–1403.
Derocher A.E., Andersen M., Wiig Ø. 2005. Sexual dimorphism of polar bears. Journal of Mammalogy, 86: 895–901.
Derocher A.E., Andersen M., Wiig Ø., Aars J., Hansen E., Biuw M. 2011. Sea ice and polar bear den ecology at Hopen Island. Svalbard, 441: 273–279.
Doutt J.K. 1967. Polar bear dens on the Twin Islands, James Bay, Canada. Journal of Mammalogy, 48(3): 468–471.
Durner G.M., Amstrup S.C., Fischbach A.S. 2003. Habitat characteristics of polar bear terrestrial maternal den sites in Northern Alaska. Arctic, 56: 55–62.
Eeyou Marine Region Wildlife Board (EMRWB). 2019. Eeyou Istchee coastal community voices on wildlife research priorities for the Eeyou Marine Region. Waskaganish, QC.
Environment and Climate Change Canada. 2021. Canadian Ice Service Archive. Available from https://www.canada.ca/en/environment-climate-change/services/ice-forecasts-observations/latest-conditions/archive-overview.html.
Erlinge S. 1967. Home range of the otter Lutra lutra L. in Southern Sweden. Oikos, 18: 186–209.
Freitas C., Kovacs K.M., Andersen M., Aars J., Sandven S., Skern-Mauritzen M., et al. 2012. Importance of fast ice and glacier fronts for female polar bears and their cubs during spring in Svalbard, Norway. Marine Ecology Progress Series, 447: 289–304.
Gardner B., Royle J.A., Wegan M.T., Rainbolt R.E., Curtis P.D. 2010. Estimating black bear density using DNA data from hair snares. Journal of Wildlife Management, 74: 318–325.
Gearheard S., Shirley J. 2007. Challenges in community-research relationships : learning from natural science in Nunavut. Arctic, 60: 62–74.
Gompper M.E., Kays R.W., Ray J.C., Lapoint S.D., Bogan D.A., Cryan J.R. 2006. A comparison of noninvasive techniques to survey carnivore communities in northeastern North America. Wildlife Society Bulletin, 34: 1142–1151.
Government of Nunavat. 2020. Nunavut polar bear management plan.
Griffen B.D. 2018. Modeling the metabolic costs of swimming in polar bears (Ursus maritimus). Polar Biology, 41: 491–503.
Gysel L., Davis E.M. Jr. 1956. A Simple Automatic Photographic Unit for Wildlife Research. The Journal of Wildlife Management, 20: 451–453.
Hamilton S.G., Derocher A.E. 2019. Assessment of global polar bear abundance and vulnerability. Animal Conservation, 22: 83–95.
Herreman J., Peacock E. 2013. Polar bear use of a persistent food subsidy: insights from non-invasive genetic sampling in Alaska. Ursus, 24: 148–163.
Humphries M.M., Samson J., Milligan H.E. 2019. The mammals of Wemindji in time, space, and ways of knowing. In Caring for Eeyou Istchee. Edited by M.E. Mulrennan, C.H. Scott, K. Scott. UBC Press, Vancouver. pp. 220–246.
Intergovernmental Panel on Climate Change. 2021. Climate change 2021: the physical science basis. IPCC.
IUCN. 2019. International Union for Conservation of Nature annual report 2019. Gland, Switzerland.
IUCN/SSC Polar Bear Specialist Group. 2021. Status report on the world’s polar bear subpopulations. July 2021 Status Report.
Iverson S.A., Gilchrist H.G., Smith P.A., Gaston A.J., Forbes M.R. 2014. Longer ice-free seasons increase the risk of nest depredation by polar bears for colonial breeding birds in the Canadian Arctic. Proceedings of the Royal Society Biological Sciences, 281:20133128.
Jagielski P., Barnas A., Gilchrist H.G., Richardson E., Love O., Semeniuk C. 2022. The utility of drones for studying polar bear behaviour in the Canadian Arctic: opportunities and recommendations. Drone Systems and Applications, 10: 97–110.
Jewell Z. 2013. Effect of monitoring technique on quality of conservation science. Conservation Biology, 27: 501–508.
Laforest B.J., Hébert J.S., Obbard M.E., Thiemann G.W. 2018. Traditional ecological knowledge of polar bears in the northern Eeyou marine region, Québec, Canada. Arctic, 71: 40–58.
Laforge M.P., Clark D.A., Schmidt A.L., Lankshear J.L., Kowalchuk S., Brook R.K. 2017. Temporal aspects of polar bear (Ursus maritimus) occurrences at field camps in Wapusk National Park. Polar Biology, 40: 1661–1670.
Laidre K.L., Stirling I., Estes J.A., Kochnev A., Roberts J. 2018. Historical and potential future importance of large whales as food for polar bears. Frontiers in Ecology and the Environment, 16: 515–524.
Lamb C.T., Walsh D.A., Mowat G. 2016. Factors influencing detection of grizzly bears at genetic sampling sites. Ursus, 27: 31–44.
Linnell J.D.C., Swenson J.E., Andersen R., Barnes B. 2000. How vulnerable are denning bears to disturbance? Wildlife Society Bulletin, 28: 400–413.
Lippold A., Boltunov A., Aars J., Andersen M., Blanchet M.A., Dietz R., et al. 2022. Spatial variation in mercury concentrations in polar bear (Ursus maritimus) hair from the Norwegian and Russian Arctic. Science of the Total Environment, 822: 153572.
Litvaitis J.A. 2000. Investigating food habits of terrestrial vertebrates. In Research techniques in animal ecology: controversies and consequences. Edited by L. Boitani, T.K. Fuller. Columbia University Press, New York. pp. 165–190.
Lunn N.J., Branigan M., Carpenter L., Justus J., Hedman D., Larsen D., et al. 2010. Polar bear management in Canada, 2005–2008. In Proceedings of the 15th Working Meeting of the IUCN. SSC Polar Bear Specialist Group. pp. 87–113.
Mallory M.L., Gilchrist H.G., Janssen M., Major H.L., Merkel F., Provencher J.F., Strøm H. 2018. Financial costs of conducting science in the Arctic: examples from seabird research. Arctic Science, 4: 624–633.
Mauritzen M., Derocher A.E., Wiig Ø., Belikov S., Boltunov A., Hansen E., Garner G.W. 2002. Using satellite telemetry to define spatial population structure in polar bears in the Norwegian and western Russian Arctic. Journal of Applied Ecology, 39: 79–90.
Merkel B., Aars J., Liston G.E. 2020. Modelling polar bear maternity den habitat in east Svalbard. Polar Research, 39: 1–9.
Natural Resources Canada. 2015. Canada Centre for Remote Sensing: 2015 Land Cover of Canada. Available from https://open.canada.ca/data/en/dataset/4e615eae-b90c-420b-adee-2ca35896caf6.
Natural Resources Canada. 2016. CanVec.
Obbard M.E., Middel K.R. 2012. Bounding the Southern Hudson Bay polar bear subpopulation. Ursus, 23: 134–144.
Obbard M.E., Cattet M.R.L., Howe E.J., Middel K.R., Newton E.J., Kolenosky G.B., et al. 2016. Trends in body condition in polar bears (Ursus maritimus) from the Southern Hudson Bay subpopulation in relation to changes in sea ice. Arctic Science, 2: 15–32.
Obbard M.E., Stapleton S., Middel K.R., Thibault I., Brodeur V., Jutras C. 2015. Estimating the abundance of the Southern Hudson Bay polar bear subpopulation with aerial surveys. Polar Biology, 38: 1713–1725.
Obbard M.E., Stapleton S., Szor G., Middel K.R., Jutras C., Dyck M. 2018. Re-assessing abundance of Southern Hudson Bay polar bears by aerial survey: effects of climate change at the southern edge of the range. Arctic Science, 4: 634–655.
Paetkau D., Amstrup S.C., Born E.W., Calvert W., Derocher A.E., Garner G.W., et al. 1999. Genetic structure of the world’s polar bear populations. Molecular Ecology, 8: 1571–1584.
Peacock E., Derocher A.E., Thiemann G.W., Stirling I. 2011. Conservation and management of Canada’s polar bears (Ursus maritimus) in a changing Arctic. Canadian Journal of Zoology, 89: 371–385.
Pilfold N.W., Hedman D., Stirling I., Derocher A.E., Lunn N.J., Richardson E. 2016. Mass loss rates of fasting polar bears. Physiological and Biochemical Zoology, 89: 377–388.
Pilfold N.W., Mccall A., Derocher A.E., Lunn N.J., Richardson E. 2017. Migratory response of polar bears to sea ice loss: to swim or not to swim. Ecography, 40: 189–199.
Polar Bear Range States. 2015. Circumpolar action plan: conservation strategy for polar bears. A product of the representatives of the parties to the 1973 Agreement on the Conservation of Polar Bears.
Provencher J.F., McEwan M., Mallory M.L., Braune B.M., Carpenter J., Harms N.J., et al. 2013. How wildlife research can be used to promote wider community participation in the North. Arctic, 66: 237–243.
R Core Team. 2021. R: A language and environment for statistical computing. R Foundation for Statistical Computing, Vienna, Austria. Available from https://www.R-project.org/.
Regehr E.V., Lunn N.J., Amstrup S.C., Stirling I. 2007. Effects of earlier sea ice breakup on survival and population size of polar bears in Western Hudson Bay. J. Wildlife Management, 71: 2673–2683.
Rogers M.C., Peacock E., Simac K., O'Dell M.B., Welker J.M. 2015. Diet of female polar bears in the southern Beaufort Sea of Alaska: evidence for an emerging alternative foraging strategy in response to environmental change. Polar Biology, 38: 1035–1047.
Russell R. 1975. The food habits of polar bears of James Bay and Southwest Hudson Bay in summer and autumn. Arctic. 28(2): 117–129.
Schlesinger W.H., Reynolds J.F., Cunningham G.L., Huenneke L.F., Jarrell W.M., Virginia R.A., Whitford W.G. 1990. Biological feedbacks in global desertification. Science, 247: 1043–1048.
Semple H.A., Gorecki D.K.J., Farley S.D., Ramsay M.A. 2000. Pharmacokinetics and tissue residues of Telazol® in free-ranging polar bears. Journal of Wildlife Diseases, 36: 653–662.
Statistics Canada. 2011. Boundary files, 2011 census. Catalogue no. 92-160-X.
Stern H.L., Laidre K.L. 2016. Sea-ice indicators of polar bear habitat. The Cryosphere, 10: 2027–2041.
Stewart D.B., Lockhart W.L. 2005. An overview of the Hudson Bay marine ecosystem. Canadian Technical Report of Fisheries and Aquatic Sciences 2586. Winnipeg, MB.
Stirling I., Derocher A.E. 1993. Possible impacts of climatic warming on polar bears. Arctic, 46: 240–245.
Stirling I., Parkinson C.L. 2006. Possible effects of climate warming on selected populations of polar bears (Ursus maritimus) in the Canadian Arctic. Arctic, 3: 261–275.
Stirling I., Lunn N.J., Iacozza J., Elliott C., Obbard M. 2004. Polar bear distribution and abundance on the southwestern Hudson Bay coast during open water season in relation to population trends and annual ice patterns. Arctic, 57: 15–26.
Stirling I., Spencer C., Andriashek D. 1989. Immobilization of polar bears (Ursus maritimus) with Telazol in the Canadian Arctic. Journal of Wildlife Diseases, 25: 159–168.
Stirling I., Thiemann G.W., Richardson E. 2008. Quantitative support for a subjective fatness index for immobilized polar bears. Journal of Wildlife Management, 72: 568–574.
Van Coeverden De Groot P., Wong P.B.Y., Harris C., Dyck M.G., Kamookak L., Pagès M., Michaux J., Boag P.T. 2013. Toward a non-invasive inuit polar bear survey: Genetic data from polar bear hair snags. Wildlife Society Bulletin. 37(2): 394–401.
Viengkone M., Derocher A.E., Richardson E.S., Obbard M.E., Dyck M.G., Lunn N.J., et al. 2018. Assessing spatial discreteness of Hudson Bay polar bear populations using telemetry and genetics. Ecosphere, 9: e02364.
Vongraven D., Aars J., Amstrup S., Atkinson S.N., Belikov S., Born E.W., et al. 2012. A circumpolar monitoring framework for polar bears. Ursus, 23: 1–66.
Wong C., Ballegooyen K., Ignace L., (Gùdia) Johnson M.J., Swanson H. 2020. Towards reconciliation: 10 calls to action to natural scientists working in Canada. FACETS, 5: 769–783.
Woods J.G., Paetkau D., Lewis D., McLellan B.N., Proctor M., Strobeck C. 1999. Genetic tagging of free-ranging black and brown bears. Wildlife Society Bulletin, 27: 616–627.
Supplementary material
Supplementary Material 1 (DOCX / 5.16 MB).
- Download
- 5.16 MB
Information & Authors
Information
Published In
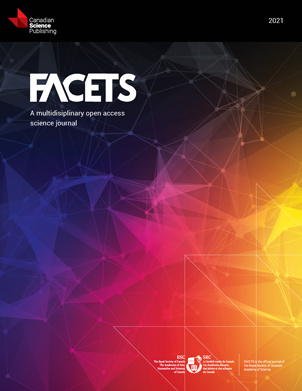
FACETS
Volume 8 • January 2023
Pages: 1 - 12
Editor: Mark Mallory
History
Received: 28 October 2022
Accepted: 3 April 2023
Version of record online: 27 July 2023
Copyright
© 2023 The Author(s). This work is licensed under a Creative Commons Attribution 4.0 International License (CC BY 4.0), which permits unrestricted use, distribution, and reproduction in any medium, provided the original author(s) and source are credited.
Key Words
Sections
Subjects
Plain Language Summary
Using hair samples and photos to study polar bears with Cree communities in the Eeyou Marine Region of James Bay
Authors
Author Contributions
Conceptualization: AL, AC, AJ, MMH
Formal analysis: AL
Funding acquisition: AL, AC, NL, MMH
Investigation: AL, SV, FB, SD, JL, AJ, GN, DO
Methodology: AL, AJ, MMH
Project administration: AC, NL, SV, FB, SD, JL, GN, DO
Resources: AC, NL, SV, FB, SD, JL, GN, DO
Supervision: MMH
Validation: AJ
Visualization: AL, MMH
Writing – original draft: AL, MMH
Writing – review & editing: AC, NL, SV, FB, SD, JL, AJ, GN, DO, MMH
Competing Interests
The authors declare that we have no competing interests.
Metrics & Citations
Metrics
Other Metrics
Citations
Cite As
Alexandra Langwieder, Angela Coxon, Natasha Louttit, Stephanie Varty, Felix Boulanger, Sanford Diamond, John Lameboy, Anderson Jolly, George Natawapineskum, Derek Okimaw, and Murray M. Humphries. 2023. Community-led non-invasive polar bear monitoring in the Eeyou Marine Region of James Bay, Canada: insights on distribution and body condition during the ice-free season. FACETS.
8: 1-12.
https://doi.org/10.1139/facets-2022-0226
Export Citations
If you have the appropriate software installed, you can download article citation data to the citation manager of your choice. Simply select your manager software from the list below and click Download.
Cited by
1. Coexistence between people and polar bears supports Indigenous knowledge mobilization in wildlife management and research
2. “Bears are like family”: Indigenous knowledge of human-polar bear coexistence amidst rapid social-ecological change