Survey of rice (Oryza sativa L.) production ecosystems in northern Ghana confirms low risk of exposure to potential toxic elements from local grain consumption
Abstract
1. Introduction
2. Method
2.1. Study sites
2.2. Sample collection
2.3. Sample preparation and analysis
2.3.1. Acid digestion of water samples
2.3.2. Digestion of soil samples
2.3.3. Digestion of rice samples
2.3.4. Determination of elemental concentrations
2.3.5. Quality control
As | Cd | Pb | ||
---|---|---|---|---|
ISE Sample 999 | Certified value (mg/kg) | 0.772 ± 0.344 | 0.040 ± 0.020 | 4.710 ± 1.157 |
Measured value (mg/kg) | 0.852 ± 0.223 | 0.048 ± 0.031 | 4.907 ± 0.235 | |
Mean recovery (%) | 95.3 | 99.6 | 98.7 | |
NIST SRM 1547 | Certified value (mg/kg) | 0.062 ± 0.014 | 0.0261 ± 0.0022 | 0.869 ± 0.018 |
Measured value (mg/kg) | 0.066 ± 0.014 | 0.0203 ± 0.0021 | 0.877 ± 0.021 | |
Mean recovery (%) | 97.6 | 99.3 | 99.6 |
2.4. Data analysis
3. Results
3.1. Soil Physicochemical properties
Rice production system | |||||
---|---|---|---|---|---|
Soil property | Region | Rainfed upland (n = 3) | Rainfed lowland (n = 3) | Irrigated lowland (n = 3) | P-value |
pH | Northern | 5.6 ± 0.3 | 6.1 ± 0.7 | 5.4 ± 0.3 | 0.248 |
Upper East | 5.3 ± 0.2 | 5.2 ± 0.2 | 5.1 ± 0.1 | 0.387 | |
EC (µScm−1) | Northern | 55.0 ± 7.4 | 76.7 ± 41.8 | 85.3 ± 42.3 | 0.572 |
Upper East | 127.8 ± 73.3 | 55.8 ± 29.2 | 61.8 ± 15.6 | 0.190 | |
% Sand | Northern | 18.0 ± 0.6a | 16.3 ± 2.5a | 58.7 ± 8.4b | < 0.001 |
Upper East | 23.7 ± 3.1 | 28 ± 5.3 | 26 ± 1.0 | 0.391 | |
% Silt | Northern | 73.0 ± 0.6a | 74.7 ± 2.9a | 33.0 ± 8.7b | < 0.001 |
Upper East | 47 ± 3.0 | 43.3 ± 3.1 | 44.3 ± 2.9 | 0.361 | |
% Clay | Northern | 9.0 ± 1.2 | 9.0 ± 1.7 | 8.3 ± 0.6 | 0.761 |
Upper East | 29.3 ± 2.5 | 28.7 ± 2.3 | 29.7 ± 2.1 | 0.867 | |
Texture | Northern | Silt loam | Silt loam | Sandy loam | – |
Upper East | Clay loam | Clay loam | Clay loam | – |
Note: Means in the same row with the same superscript are not statistically different at P ≤ 0.05. EC, electrical conductivity.
3.2. Elemental concentrations in water samples
Sampling site | Region | As (mg/L) | Cd (mg/L) | Pb (mg/L) |
---|---|---|---|---|
Golinga (n = 3) | Northern | 0.005 ± 0.001 | 0.008 ± 0.003 | BD |
Bontanga (n = 3) | Northern | 0.005 ± 0.001 | 0.009 ± 0.004 | BD |
Tono (n = 3) | Upper East | 0.006 ± 0.003 | BD | 0.011 ± 0.019 |
Navrongo Borehole (n = 2) | Upper East | 0.004 ± 0.002 | BD | BD |
P-value | 0.070 | – | – |
Note: BD, below detection; As, arsenic; Cd, cadmium; Pb, lead.
3.3. Elemental concentrations in rice field soils
Soil PTE concentration in mg/kg | |||||
---|---|---|---|---|---|
Element | Region | Rainfed upland | Rainfed lowland | Irrigated lowland | P-value |
As | Northern | 0.003 ± 0.001 | 0.006 ± 0.004 | 0.016 ± 0.012 | 0.458 |
Upper East | 0.053 ± 0.003 | 0.053 ± 0.005 | 0.053 ± 0.003 | 0.985 | |
Cd | Northern | 0.019 ± 0.010 | 0.014 ± 0.003 | 0.042 ± 0.019 | 0.309 |
Upper East | BD | BD | BD | – | |
Pb | Northern | 0.394 ± 0.156 | 0.843 ± 0.087 | 0.600 ± 0.268 | 0.282 |
Upper East | 0.193 ± 0.159 | 0.614 ± 0.236 | 0.432 ± 0.138 | 0.270 |
Note: PTE, potential toxic element; BD, below detection; As, arsenic; Cd, cadmium; Pb, lead.
3.4. Elemental concentrations in rice grain samples
Rice production system | |||||
---|---|---|---|---|---|
Region | Rainfed upland | Rainfed lowland | Irrigated lowland | P-value | |
Grain As (mg/kg) | Northern | 0.005 ± 0.001 | 0.004 ± 0.002 | 0.004 ± 0.000 | 0.580 |
Upper East | 0.061 ± 0.002 | 0.063 ± 0.003 | 0.064 ± 0.006 | 0.699 | |
Grain Cd (mg/kg) | Northern | 0.001 ± 0.001 | 0.011 ± 0.006 | 0.023 ± 0.003 | 0.013 |
Upper East | BD | BD | BD | – | |
Grain Pb (mg/kg) | Northern | 0.083 ± 0.015 | 0.092 ± 0.020 | 0.104 ± 0.041 | 0.671 |
Upper East | 0.024 ± 0.022 | 0.077 ± 0.045 | 0.025 ± 0.022 | 0.141 |
Note: BD, below detection; As, arsenic; Cd, cadmium; Pb, lead.
4. Discussion
5. Conclusion
Acknowledgements
References
Information & Authors
Information
Published In
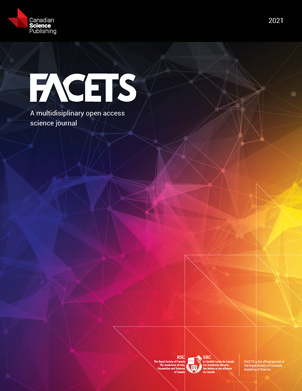
History
Copyright
Data Availability Statement
Key Words
Sections
Subjects
Plain Language Summary
Authors
Author Contributions
Competing Interests
Metrics & Citations
Metrics
Other Metrics
Citations
Cite As
Export Citations
If you have the appropriate software installed, you can download article citation data to the citation manager of your choice. Simply select your manager software from the list below and click Download.
There are no citations for this item