Electronic cigarettes—A review of the physiological health effects
Abstract
Introduction

Methods
Results and discussion
Composition of the EC device
Composition of the EC liquid
Composition of unflavoured EC aerosols
Composition of flavoured EC liquids and aerosols
How manufacturing can affect the composition of EC liquids and aerosols
Cellular level effects
Reference | Cell type(s) and (or) organism type(s) | In vitro and (or) in vivo | Exposure | Methods | Results |
---|---|---|---|---|---|
Bahl et al. (2012) | hESC, mNSC, hPF | In vitro | EC liquid over 48 h | Test 35 EC liquids for toxicity with MTT colorimetric assay | Varying cytotoxicity among different brands/flavours though hESCs were almost always more sensitive than mNSC or hPF. |
Cervellati et al. (2014) | A549, HaCaT | In vitro | 50 min exposure to EC vapour, sample periodically over 24 h | Vacuum pump for exposure, trypan blue and LDH assay for viability | EC without flavour or nicotine: no effect. EC with flavour and EC with nicotine decreased viability and increased cell damage, similar to CCs. |
Farsalinos et al. (2013) | H9c2 | In vitro | 2.5 and 4 s puffs of vapour every 60 s for high and regular voltage EC devices, until consumption of 200 mg of EC liquid: H9c2 cells soaked in liquid for 24 h | Test 20 EC liquids for toxicity with MTT colorimetric assay | Varying cytotoxicity among different brands/flavours, more pronounced effects at higher concentrations, cell survival was not associated with nicotine concentration of EC liquids, viability reduced for EC extracts produced by high-voltage EC devices. |
Garcia-Arcos et al. (2016) | NHBE, live mice | In vitro and in vivo | NHBE cells: puffs of vapour every 30 s for a total of 36 puffs | In vivo: Histological and immuno-fluorescence analysis of lung tissue, TUNEL cell viability test, qPCR for gene expression | In vivo: nicotine-containing EC vapour increased cytokine and protease expression, airway hyperreactivity, lung tissue destruction, mucin production whereas nicotine-free EC vapour did not. |
Mice: 1 h of aerosol per day for four months | In vitro: LDH release for viability, histological analysis | In vitro: nicotine-containing EC vapour impaired ciliary beat frequency, airway surface liquid volume, cystic fibrosis transmembrane regulator, and ATP-stimulated K+ ion conductance of NHBE cells. | |||
Higham et al. (2016) | Human neutrophils | In vitro | 10 s puffs of vapour every 30 s for a total of 13 min | Expression of CD11b and CD66b measured by flow cytometry, MMP-9 and CXCL8 by ELISA | Vapours increased expression of CD11b and CD66b (markers of neutrophil activation) and increased neutrophil elastase and MMP-9 activity (both causing tissue destruction and leading to emphysema). |
Husari et al. (2016) | A549, live mice | In vitro and in vivo | A549 cells: EC condensate added to culture media, media tested at various concentrations of aerosol extracts | In vitro: cell titer blue assay for viability | No albumin leak, no cell death, and low levels of lung damage found in ECs when compared with CCs. Significant increase in IL-1β for ECs and in all three mediators (1β, IL-6, TNF-α) for CC use. |
Mice: 6 h of aerosol per day for 3 d | In vivo: albumin level, qPCR for inflammatory mediators IL-1β, IL-6, TNF-α, tested oxidative stress and cell death | ||||
Leigh et al. (2016) | H292 | In vitro | 3 s puffs of vapour every 30 s for a total of 30 min | Neutral red test for metabolic activity, trypan blue assay for viability, measured cytokine release with ELISA | Vapours decreased metabolic activity and cell viability and increased release of various cytokines, CCs had more detrimental effects on cell viability and metabolic activity, metabolic activity and viability reduced further for EC extracts produced by higher voltage EC devices (4 and 4.8 V vs. 3.3 V), cytotoxicity varied depending on the type of flavour used. |
Lerner et al. (2015b) | H292, HFL-1, live mice | In vitro and in vivo | H292 cells: 4 s puffs of vapour every 30 s for 5, 10, or 15 min | In vitro: tested ROS generation and IL-8 release | In vitro: ROS generated by device, humectants, and complete liquids, IL-8 release correlated with nicotine. |
HFL-1 cells: soaked in liquid for 24 h | In vivo: tested macrophage and IL release | In vivo: vapours caused increased macrophages and IL in lungs. | |||
Mice: 5 h of aerosol per day for 3 d | |||||
Lim and Kim (2014) | Live mice | In vivo | Inject EC liquid into trachea twice weekly for 10 weeks, tested 24 h after last dose | Measure airway inflammation and airway hyperresponsiveness | EC increased hyperresponsiveness and attracted leukocytes and eosinophils (indicating inflammation and allergies). |
Misra et al. (2014) | A549 | In vitro | 2 s puffs of vapour every 30 s or soaked in EC liquid, tested 24 h after exposure | Neutral red test for cytotoxicity, measured cytokine release with ELISA, Ames and micronucleus assay for mutagenicity | No effect besides IL-8 release at very high dose of EC liquid. |
Neilson et al. (2015) | Human tracheobronchial epithelium: EpiAirway™ | In vitro | 3 s puffs of vapour every 30 s for a total of 30 min | Test for toxicity with MTT colorimetric assay | No decreased cell viability with EC vapour, 12% decrease for CC smoke. |
Palpant et al. (2015) | hESC, live zebrafish embryos | In vitro and in vivo | Vapour drawn over culture media for cells and embryos, tested 24, 48, and 72 h after exposure | In vitro: tested gene expression | In vitro: vapours reduced expression of sarcomeres. |
In vivo: observed cardiac development | In vivo: vapours caused cardiac defects (decreased contractile and junctional proteins) unrelated to nicotine, no effect on heart beat. | ||||
Romagna et al. (2013) | BALB/3T3 | In vitro | 2 s puffs every 60 s, tested 24 h after exposure | MTT assay for viability of 21 EC liquids at different concentrations | Only one “coffee” liquid was cytotoxic and only at the highest concentration tested. |
Scheffler et al. (2015a) | NHBE, A549 | In vitro | 2 s puffs every 10 s, tested 24 h after exposure | Cell titer blue assay for viability, ROS-Glo H2O2 assay for oxidative stress | Vapours decreased viability unrelated to nicotine, bronchial cells more sensitive than A549, bronchial cells showed oxidative stress, not A549. |
Scheffler et al. (2015b) | NHBE | In vitro | 2 s puffs, 200 puffs, tested 24 h after exposure | Cell titer blue assay for viability, ROS-Glo H2O2 assay for oxidative stress | EC vapour, propylene glycol, and glycerol reduced viability and increased oxidative stress unrelated to nicotine. |
Schweitzer et al. (2015) | Rat lung epithelial cells, NHBE, primary mouse lung endothelial cells, primary human lung microvascular cells, live mice | In vitro and in vivo | In vitro: 125 μL of EC condensate added to culture media | MTT assay for viability, tested oxidative stress, tested protein expression | In vitro: vapours caused decreased viability and growth unrelated to nicotine and increased oxidative stress (increased ROS). |
In vivo: 2 doses (1 μg each) of vapour, tested 30 min or 24 h after exposure | In vivo: vapours caused oxidative stress (increased in 8-OHdG) and increased polymorphonuclear cells in lungs (inflammation). | ||||
Sussan et al. (2015) | Live mice | In vivo | 2 s puffs every 10 s for 1.5 h twice a day for two weeks, tested 24 h after exposure | Measured IL levels, susceptibility to bacterial and viral infections | Vapours caused oxidative stress (but no IL release), macrophage infiltration, increased susceptibility to bacterial infections (and macrophage phagocytosis was impaired), and viral infections (more infected cells and death than in the control). |
Tartell (2015) | A549 | In vitro | Soaked in liquid for up to 120 min | LDH release for viability, tested protein expression | Liquid was cytotoxic above 5%, caused release of NF Kappa genes which stimulate inflammation. |
Teasdale et al. (2016) | HCAEC | In vitro | EC condensate added to culture media with a 350 ng/mL nicotine concentration | qPCR for expression of regulatory genes, immuno-chemistry to detect oxidant-stress sensing transcription factor NFR2 | Oxidative stress pathway activated and cytochrome p450 upregulated for CC smoke condensate, but not EC condensate. |
Willershausen et al. (2014) | Human periodontal ligament fibroblasts | In vitro | Soaked in liquid for up to 96 h | Presto Blue cell proliferation assay for viability, tested cell migration | Only menthol flavoured liquid decreased viability (not hazelnut, lime, or pure nicotine), only menthol reduced migration. |
Wu et al. (2014) | hTBE | In vitro | Soaked in liquid for up to 48 h | LDH release for viability, tested IL levels, tested response to viral infection | No cytotoxicity, liquids caused IL-6 release, more so when it contained nicotine, liquid increased number of virally infected cells and decreased SPLUNCI (virus defense molecule) levels for nicotine and non-nicotine liquids. |
Yu et al. (2016) | HaCAT, hNSCC | In vitro | Vapour absorbed by media used to culture cells, replaced every 3 d for eight weeks (HaCaT) or one week (hNSCC) | Trypan blue assay for viability, detected double-stranded DNA breaks by detecting γ-H2AX, detected cells undergoing apoptosis | HaCat: 1.5 and 3 times more double-strand breaks for non-nicotine and nicotine vapour, respectively, 0.53 to 0.68 times more necrosis, 1.2 to 2 times more apoptosis than in the control. |
hNSCC: 1.5 and 2 times more double-strand breaks for non-nicotine and nicotine vapour, respectively, 0.2 to 1.34 times more necrosis, 1.21 to 2.58 times more apoptosis than in the control. | |||||
Both cells: 5 and 10 times more cell death for non-nicotine and nicotine vapours, respectively. |
Note: EC, electronic cigarette; hESC, human embryonic stem cells; mNSC, mouse neural stem cells; hPF, human pulmonary fibroblasts; A549, human lung epithelial cells; HaCaT, human keratinocytes; H9c2, human cardiomyocytes; NHBE, human bronchial epithelial cells; H292, human bronchial epithelial cells; HFL-1, human fetal lung fibroblasts; BALB/3T3, mouse embryo fibroblasts; HCAEC, human coronary artery endothelial cells; hTBE, human tracheobronchial epithelial cells; HaCAT, human keratinocytes; hNSCC, head and neck squamous cell carcinoma; ROS, reactive oxygen species; CC, conventional cigarette.
Overall physiological effects
Delivery of nicotine to the blood by EC devices
Effects of ECs on cardiovascular function
Effects of ECs on pulmonary function
Effects of ECs on metabolism and development
Effects of ECs on cognitive function, anxiety, and dependence
Acute adverse effects caused by EC use
Conclusions
Acknowledgements
References
Information & Authors
Information
Published In
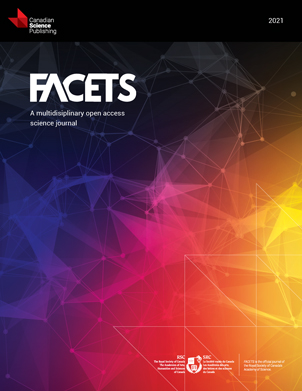
History
Copyright
Data Availability Statement
Key Words
Sections
Subjects
Plain Language Summary
Authors
Author Contributions
Competing Interests
Metrics & Citations
Metrics
Other Metrics
Citations
Cite As
Export Citations
If you have the appropriate software installed, you can download article citation data to the citation manager of your choice. Simply select your manager software from the list below and click Download.