Spatial distribution and conservation hotspots of mammals in Canada
Abstract
High-latitude countries often contain the polar range edge of species that are common farther south, potentially focusing national conservation efforts toward range-edge populations. The global conservation value of edge populations is controversial, but if they occur where biodiversity is high, there need not be trade-offs in protecting them. Using 152 of 158 terrestrial mammal species in Canada, we tested how species’ distributions relate to their national conservation status and total mammal richness. We found that half of “Canadian” mammals had <20% of their global range in Canada. National threat status was strongly associated with range area; mammals considered “at risk” had 42% smaller Canadian ranges than mammals considered secure. However, after accounting for range area, taxa with smaller proportions of their global range in Canada were not more likely to be considered at risk, suggesting edge populations are not inherently more vulnerable. When we calculated mammal diversity across Canada (100 × 100 km grid cells), we found that hotspots of at-risk or range-edge mammals were twice as species rich as nonhotspot cells, containing up to 44% of Canadian mammal diversity per grid cell. Our results suggest that protecting areas with the most at-risk or range-edge mammals would simultaneously protect habitat for many species currently deemed secure.
Introduction
Large polar countries often contain the high-latitude range edge of species that are widely distributed beyond their borders (Cheffings and Farrell 2005; Gibson et al. 2009; Rassi et al. 2010; ArtDatabanken 2015). Such “peripheral” species will tend to have smaller national ranges, and range-edge populations are often predicted to be smaller, more isolated, or less productive than more central populations (Brown et al. 1996; Sagarin and Gaines 2002; Yakimowski and Eckert 2007), although this pattern is far from universal (Samis and Eckert 2009; Pironon et al. 2017; Hargreaves and Eckert 2019). Small national ranges and unstable populations may make peripheral species more likely to be deemed nationally at risk, focusing conservation efforts toward edge populations of taxa that may be globally secure (Hunter and Hutchinson 1994; Lesica and Allendorf 1995).
The value and practicality of conserving range-edge populations are contentious. Ethically, some argue that countries have the greatest obligations to their most endemic taxa, and that taxa are not truly at risk if they are globally secure (Hunter and Hutchinson 1994). Practically, edge populations may be difficult to conserve if they are inherently unstable due to small numbers, low genetic diversity, or poor habitat (Hunter and Hutchinson 1994), although again these patterns are not universal (Eckert et al. 2008). On the other hand, if edge populations occupy unusual habitats they can be important for diversification and range expansion via local adaptation (Van Rossum et al. 2003; Mimura and Aitken 2010; Hargreaves and Eckert 2019). Populations at species’ polar range edge are also geographically poised to initiate poleward range shifts in response to climate warming (Gibson et al. 2009). This geographic “head start” is especially important when species’ dispersal ability is low compared with the pace of climate change, as is true for many terrestrial mammals (Thomas et al. 2004; Schloss et al. 2012).
Evaluating the relative conservation merits of peripheral species would be less important if protecting edge populations involved few conservation trade-offs. One important conservation strategy is establishing protected areas, which will be most effective if multiple types of biodiversity co-occur (Prendergast et al. 1993; Myers et al. 2000; Ricketts et al. 2005). Previous studies have not found significant co-occurrence of rare or threatened taxa and overall species richness, either at a global scale for vertebrates (Orme et al. 2005; Ceballos and Ehrlich 2006; Jenkins et al. 2013), or in the UK for invertebrates, plants, and birds (Prendergast et al. 1993). However, nationally threatened taxa and overall richness may be more likely to co-occur in large high-latitude countries if peripheral taxa are often nationally at risk, since peripheral taxa and biodiversity will concentrate toward the equatorward border (Buckley et al. 2010).
We explore the co-occurrence of at-risk taxa, peripheral taxa, and overall richness using terrestrial mammals in Canada. Canada is the world’s second largest country, but as a northern country Canada’s most biodiverse ecosystems (e.g., Carolinian forest) are concentrated at its southern border (Boyle 1991). As such, many species considered at risk in Canada occur here as range-edge populations and are widely distributed elsewhere (Gibson et al. 2009; Caissy et al. 2020). How Canada protects such edge populations matters not only for species already in Canada but also for those south of the Canadian border, who may be forced by climate change to shift their distributions northward. While it has long been acknowledged that many nationally at-risk species are peripheral in Canada (Fraser 1999), it is unclear whether this is simply because most taxa in Canada are peripheral, because peripheral taxa have smaller national ranges, or because edge populations are inherently more at risk. Terrestrial mammals are useful for answering these questions as they are well mapped (IUCN 2018), so we can quantify the area and proportion of each species’ range in Canada and compare these directly between at-risk and non-at-risk species.
Using range maps from the International Union for the Conservation of Nature (IUCN) and Canadian conservation statuses from the Committee on the Status of Endangered Wildlife in Canada (COSEWIC), we asked two questions. Question 1: How do range area in Canada and the percentage of global range in Canada (lower = more peripheral) relate to national conservation status? Since national range area is one of several criteria COSEWIC uses to evaluate risk, we expected that at-risk taxa will have smaller Canadian ranges (model a). Given predictions that range-edge populations are less demographically stable and (or) more threatened due to their proximity to high human land-use (Coristine and Kerr 2011; Caissy et al. 2020), we predicted that at-risk taxa will have less of their range in Canada (model b) and that species with less of their range in Canada will be more likely to be considered at risk in Canada even after controlling for range area (model c). We then divided Canada into 100 × 100 km grid-cells, and asked Question 2: Do cells with the most at-risk or range-edge mammals (hotspots) occur in regions of high overall mammal richness? If range-edge taxa are over-represented among at-risk taxa (Question 1), we predict that hotspots will have higher total mammal richness than nonhotspot cells since edge populations and species richness both cluster toward Canada’s low-latitude border.
Methods
We recorded the Canadian conservation status for each land mammal taxon assessed by COSEWIC. COSEWIC is Canada’s conservation assessment body, mandated to provide a scientifically based assessment of flora and fauna at risk of going extinct in Canada. COSEWIC classifications are based on the size, stability, and vulnerability of populations in Canada, but COSEWIC may lower a species by one threat category if there is evidence of immigration from outside Canada (i.e., a rescue effect; COSEWIC 2018). We used COSEWIC assessments rather than official status under Canada’s Species at-Risk Act (SARA) as COSEWIC addresses only conservation risk, whereas SARA also considers the economic impact of a given listing. Taxa per threat category were: 10 Special concern, 5 Threatened, 7 Endangered, and 8 assessed as Not at Risk (Fig. S1). The remaining 123 mammal taxa had not been assessed. As COSEWIC assesses species in order of perceived risk, we assume that those that have not been assessed in the 41 years of COSEWIC assessments are relatively secure. Given the low sample sizes within at-risk categories, we binned taxa as either at risk (Special Concern, Threatened, or Endangered) or secure (assessed as Not at Risk or not assessed).
We obtained distribution maps in the form of spatial polygons from the IUCN (2018). We first selected the 158 terrestrial mammal species whose distribution polygon overlapped a polygon map of Canada (Natural Earth 2018). IUCN generally maps (and assesses) taxa at the species level, but COSEWIC sometimes provides distinct assessments for subspecies or populations within species. We retained subspecies and populations if we could subset the IUCN polygon to create a distribution map similar to the map in their COSEWIC assessment. This was possible for two bison subspecies (Bison bison ssp. athabascae and bison) and one caribou population (Rangifer tarandus Newfoundland population), whose distributions each corresponded to a specific island. We excluded six at-risk taxa whose IUCN polygons did not match the geographic scale of their COSEWIC assessments (subspecies or populations of Canis lupus, Martes americana, Mustela erminea, Reithrodontomys megalotis, Sylvilagus nuttallii, Taxidea taxus). This yielded 153 taxa: 150 species, 2 subspecies, 1 population (152 unique species in total; Fig. 1; full list of taxa, COSEWIC status, and inclusion is in our archived data available at zenodo.org/record/3972324). Each map was cropped to land only by overlapping it with a world boundary map (Natural Earth 2018) using the “sf” package (version 0.7-3, Pebesma 2018) in the statistical platform R (version 3.5.1, R Core Team 2018). As we were interested in range area, polygons were projected into Albers equal area projection (Gibson et al. 2009).
Fig. 1.

Question 1: How do range area in Canada and range percentage in Canada relate to national conservation status?
We determined each taxon’s range area and range percentage in Canada using the “sf” package. We determined range area in Canada (km2) by overlapping each species’ map with a boundary map of Canada (Natural Earth 2018), then measuring the area of overlap. Canadian range area varied from 2 km2 (Evening bat Nycticeius humeralis) to >9 million km2 (North American river otter Lontra canadensis), with mean = 1 678 850 km2 and median = 460 130 km2. Due to the large spread of range areas, we ln-transformed Canadian range area for analyses (see below). We quantified each taxon’s global range area by measuring the area of overlap between its range map and a global land map. Global range area varied from 2075 km2 (Vancouver Island marmot Marmota vancouverensis) to >22 million km2 (Cougar Puma concolor), with mean = 4 449 710 km2 and median = 3 263 110 km2. We calculated the percentage of each taxon’s global range that occurs in Canada (i.e., the inverse of peripherality in Canada) as Canadian range area or Global range area × 100; this varied from <0.001% (Evening bat) to 100% (Ogilvie Mountains collared lemming Dicrostonyx nunatakensis and Vancouver Island marmot).
We tested the relationships between range area in Canada, range percentage in Canada, and conservation status in Canada using three models. We tested whether range area (ln(range area), Gaussian response; model a) or percentage (proportion response; model b) in Canada differed between taxa deemed at risk or secure (conservation status = binomial predictor), using generalized linear models (GLMs) with a Gaussian and binomial error structure, respectively (glm command, base R; ln(range area) or percentage ∼ conservation status). For comparison, Fig. S2 shows results for mammals grouped by global conservation status rather than conservation status in Canada. Next, using a binomial GLM we tested whether the probability of a taxa being at risk (conservation status =binomial response) varied with range percentage in Canada (proportion predictor) even after accounting for range size (continuous predictor; model c): conservation status ∼ ln(range area) +range percentage (Venables and Ripley 2002). We assessed the significance of predictors using likelihood ratio χ2 tests (Anova type = III command, car package).
Question 2: Do hotspots of at-risk or range-edge taxa coincide with high overall richness?
To quantify the spatial distribution of mammal diversity, we overlaid a grid of 100 × 100 km cells on the Canadian equal area map. This produced a map of Canada divided into 1285 cells (not all equal in area as the Canadian border bisected some cells; Fig. S3). We overlaid the range map polygons of all terrestrial mammals on the grid map, then counted the total mammal species, at-risk species, and range-edge species in each cell. We defined range-edge species as taxa with ≤20% of their global range in Canada (as per Caissy et al. 2020); using a threshold of 10% reduced the number of range-edge taxa from 76 to 53 but did not alter conclusions to Questions 1 and 2 (Table S1). Following Prendergast et al. (1993) and Reid (1998), we identified cells with the highest richness (hotspots) of at-risk and of range-edge taxa up to a maximum of 5% of the total grid cells. We use hotspot sensu Prendergast et al. (1993) to refer to areas with high biodiversity, rather than sensu Myers (1988) as areas of high diversity and high extrinsic threat. This yielded 47 hotspot cells for at-risk taxa (5–6 at-risk taxa/cell; 3.6% of total grid cells; Fig. 2a), and 47 hotspot cells for range-edge taxa (17–29 range-edge taxa/cell; Fig. 2b). Cells containing 4 at-risk or 16 range-edge taxa were not counted as the number of hotspot cells would have exceeded 5% of total cells. This method of identifying cells of high richness creates richness indices that are reasonably independent of total species richness (Reid 1998).
Fig. 2.

Whereas regression models assume data points are independent, we detected significant spatial autocorrelation; i.e., hotspot cells for at-risk taxa occurred closer to each other than expected by chance (Moran’s I ± SD = 0.0628 ± 0.00137, p < 0.001) as did range-edge hotspots (Moran’s I ± SD =0.116 ± 0.00137, p < 0.001). We accounted for this spatial autocorrelation by incorporating the spatial configuration of data via a fixed-effect covariate (s), using the Spatial Eigenvector Mapping method (Griffith and Peres-Neto 2006; Dormann et al. 2007; Thayn and Simanis 2013). We selected the neighborhood size of the spatial configuration covariate using the value that maximized model fit based on its Akaike Information Criterion value (Fig. S4, Augustin et al. 1996; Dormann et al. 2007). Including s as a fixed-effect corrected the autocorrelation in residuals and improved model fits (Table S1).
We tested whether total mammal richness was higher in at-risk hotspots than other cells using a GLM with binomial error structure. Each cell contributed one data point. The cells’ at-risk hotspot status (at-risk hotspot or not) was a binomial response, and total mammal richness and the spatial covariate s were fixed effects (at-risk hotspot ∼ total richness + s). Significance of predictors was determined using likelihood ratio χ2tests as for Question 1. This approach is consistent with previous studies comparing at-risk versus total richness (Prendergast et al. 1993, Orme et al. 2005, Ceballos and Ehrlich 2006), but we recognize two complicating issues: first, that the response and predictors are not fully independent as an increase in at-risk richness automatically increases total richness and second, that smaller cells (bisected by the Canadian border) may contain fewer species due to sampling error. We ran two alternate GLMs to test whether our results were sensitive to either issue, one with richness of secure mammals as the predictor (at-risk hotspot ∼ secure mammal richness + s; Table S1), and one including cell area as a fixed effect (Table S1). Both models reached the same conclusions as our original model, so we present analyses using total richness and not including cell size to facilitate comparison with previous studies.
Similarly, we tested whether total mammal richness was higher in hotspots of range-edge taxa richness than other cells (range-edge hotspot ∼ total richness + s). Results using richness of non-range-edge mammals instead of total richness or including cell area as a covariate were again similar (Table S1).
Extracted data and R code for analyses are available at zenodo.org/record/3972324.
Results
In Canada, mammal richness is highest toward the south and along the western mountains (Figs. 1a, 2a and 2b), although this pattern was not apparent for the 22 taxa deemed at-risk (Fig. 1b). Half (50%) of all “Canadian” mammals had less than 20% of their global range in Canada.
Question 1: How do range area in Canada and range percentage in Canada relate to national conservation status?
Range area in Canada differed significantly between nationally at-risk and nationally secure mammals, but peripherality did not (Fig. 1). As predicted, mammals at risk of extinction in Canada had smaller Canadian ranges than mammals considered secure (model a: X2 df=1 = 6.28, p = 0.012; Fig. 1c). Interestingly, mammals considered globally at risk also had smaller ranges in Canada than those considered globally secure (Fig. S2). Contrary to our predictions, at-risk mammals did not have significantly smaller fractions of their global ranges in Canada (model b: X2 df=1 = 0.39, p = 0.53; Fig. 1d). The probability of being at risk in Canada increased as Canadian range area decreased (area: X2 df=1 = 4.54, p = 0.033), but was not affected by range percentage in Canada after controlling for range area (percentage: X2 df=1 = 0.06, p = 0.85, model c).
Question 2: Do hotspots of at-risk or range-edge taxa coincide with high overall richness?
Of the 1285 100 × 100 km grid cells in Canada, the 47 cells with the highest richness of at-risk mammals (at-risk hotspots) had significantly higher total mammal richness than the other 1238 cells (X2 df=1 = 140.3, p < 0.0001, Fig. 2c). At-risk hotspots also had significantly higher richness of secure mammals (Table S1), so this result was not driven by at-risk species. At-risk hotspot cells each contained 37–67 mammal species (24%–44% of Canada’s mammal richness; Fig. 2c), and together were home to 80% of the 153 Canadian taxa in our data. Hotspot cells clustered spatially (Fig. 2a). The three southern clusters coincided with areas known to host many at-risk species (e.g., the Carolinian forest in southwestern Ontario and St. Lawrence lowlands in southern Quebec). The two northwestern clusters were less expected; both contain four Special Concern taxa (Collared pika, Wolverine, Grizzly bear, Bison ssp athabascae) and the endangered Little brown bat, and the British Columbia cluster contains the endangered Northern long-eared bat as well.
Hotspots of range-edge species, i.e., species only present in Canada in ≤20% of their range, also had significantly higher total mammal richness than other cells (X2 df=1 = 266.8, p < 0.0001; Fig. 2d). As with at-risk hotspots, richness of non-edge mammals was also higher in hotspot cells, so this result was not driven purely by edge species (Table S1). Range-edge hotspot cells each contained 41–67 mammal species (27%–44% of Canada’s mammal richness), and together were home to 81% of the 153 mammal taxa in our data.
Notably, the two types of hotspot partially coincided with each other. Almost a third of at-risk hotspot cells were also range-edge hotspots and vice versa (15 of 79 hotspot cells overlapped; Fig. 2).
Discussion
Our quantitative analyses of mammal distributions in Canada revealed three main findings. First, our results showed that Canada is a land of edge populations; half the mammals that live in Canada only do so at the northernmost 20% or less of their global range (Fig. 1d). Second, we showed that mammals with smaller Canadian ranges are more likely to be nationally at risk (as expected since range area is considered during risk assessment; Fig. 1a), but that being more peripheral in Canada was not associated with higher likelihood of being at risk in Canada (Fig. 1d and model c). Thus, we found no evidence that range-edge populations are more vulnerable due to their demography (e.g., putatively small or isolated populations) or coincidence with areas of high human population density. Third, we found significant spatial overlap between high mammal diversity and hotspots of both at-risk mammals and range-edge mammals (Fig. 2). This result is particularly exciting, as it suggests that protecting habitat for at-risk mammals could have significant co-benefits to mammals considered secure and that protecting range-edge populations need not involve a trade-off with protecting Canada’s overall mammal diversity.
Our finding of high spatial overlap between total mammal richness and at-risk mammal richness (Fig. 2c) contrasts results from other areas and taxa. Globally, hotspots of at-risk mammals do not strongly overlap areas of high total mammal richness (10% overlap; Ceballos and Ehrlich 2006), nor do hotspots of at-risk birds strongly overlap with hotspots of total bird richness (4.7% overlap; Orme et al. 2005). Diversity is driven largely by energy availability and biogeographic history (Gaston 2000), whereas threats to wildlife are driven by human impacts (Schipper et al. 2008; Szabo et al. 2012). Poor global congruence between at-risk and total diversity suggests these drivers have different global distributions (Orme et al. 2005). In Canada however, mammal richness concentrates in the south and west (Fig. 1), as does the intensity of human land conversion (Kerr and Cihlar 2004; Kerr and Deguise 2004). To the extent that diversity and human impacts co-occur in other high-latitude countries, high overlap between at-risk and total diversity may be more common at regional than global scales.
Of course, habitat protection generally happens on a much smaller scale than our 100 ×100 km grid cells, and species rarely occupy all land area within their occurrence polygon. Protecting habitat within a hotspot may not capture all the species whose ranges overlap with the larger grid cell. Nevertheless, identifying hotspots at a coarse scale is still useful for conservation planning; the hotspots identified here should now be priorities for finer-scale assessments (Rodrigues et al. 2004). This could not be more timely, as Canada has committed to doubling its protected area network to meet United Nations guidelines (Coristine et al. 2018). Further, we are in an era of species on the move, as species shift their geographic distributions in response to anthropogenic change (Chen et al. 2011; Freeman et al. 2018). Protecting habitat in high diversity areas provides habitat options that nearby species may well use in the future, even if they don’t use them currently.
Cells that were hotspots for both at-risk and range-edge taxa are particularly interesting for long-term conservation. Since the highest densities of mammals deemed at risk in Canada occur in the United States (Fig. 1b), one effective conservation strategy for many at-risk taxa in Canada may be to maintain habitat connections to US populations. This would both enable natural immigration and population replenishment and provide bridges if or when species begin to shift northward in response to warming, both of which could bolster Canadian populations. Indeed, given worldwide range shifts to higher latitudes (Chen 2011), Canada’s current peripheral species may become much less peripheral in the coming decades, and much of Canada’s future biodiversity may be on our doorstep. Protecting habitat in the “double hotspots” is a win-win-win, protecting high numbers of mammals overall, protecting the at-risk species to whom Canada deems it has a national responsibility, and protecting Canada’s future biodiversity in terms of the wildlife climate-change refugees coming our way.
Acknowledgements
We thank Pascale Caissy for help with QGIS. Funds for this work were provided by NSERC (Discovery grant to ALH) and the E. Gordon Edward fund in support of undergraduate research in biology at McGill University.
References
ArtDatabanken SLU. 2015. The 2015 red list of Swedish species. Uppsala, Sweden. Available from artdatabanken.se/en/the-red-list/the-2015-red-list---summary/.
Augustin N, Mugglestone M, and Buckland S. 1996. An autologistic model for the spatial distribution of wildlife. The Journal of Applied Ecology, 33(2): 339–347.
Boyle TJB. 1991. Biodiversity of Canadian forests: current status and future challenges. Forestry Chronicle, 68(4): 444–453.
Brown JH, Stevens GC, and Kaufman DM. 1996. The geographic range: size, shape, boundaries, and internal structure. Ecology, 27(1): 597–623.
Buckley LB, Jonathan Davies T, Ackerly DD, Kraft NJB, Harrison SP, Anacker BL, et al. 2010. Phylogeny, niche conservatism and the latitudinal diversity gradient in mammals. Proceedings of the Royal Society B: Biological Sciences, 277(1691): 2131–2138.
Caissy P, Klemet-N’Guessan S, Jackiw R, Eckert CG, and Hargreaves AL. 2020. High conservation priority of range-edge plant populations not matched by habitat protection or research effort. Biological Conservation, 249: 108732.
Ceballos G, and Ehrlich PR. 2006. Global mammal distributions, biodiversity hotspots, and conservation. Proceedings of the National Academy of Sciences of the United States of America, 103(51): 19374–19379.
Cheffings CM, and Farrell L. 2005. Species status no.7. The vascular plant red data list for Great Britain. Joint Nature Conservation Committee, Peterborough, UK.
Chen IC, Hill JK, Ohlemüller R, Roy DB, and Thomas CD. 2011. Rapid range shifts of species associated with high levels of climate warming. Science, 333(6045): 1024–1026.
Coristine LE, and Kerr JT. 2011. Habitat loss, climate change, and emerging conservation challenges in Canada. Canadian Journal of Zoology, 89(5): 435–451.
Coristine LE, Jacob AL, Schuster R, Otto SP, Baron NE, Bennett NJ, et al. 2018. Informing Canada’s commitment to biodiversity conservation: a science-based framework to help guide protected areas designation through Target 1 and beyond. Facets, 3(1): 531–562.
COSEWIC. 2018. Committee on the status of endangered wildlife in Canada [online]: Available from cosewic.ca/index.php.
Dormann C, McPherson J, Araújo J, Bivand R, Bolliger J, Carl G, et al. 2007. Methods to account for spatial autocorrelation in the analysis of species distributional data: a review. Ecography, 30(5): 609–628.
Eckert CG, Samis KE, and Lougheed SC. 2008. Genetic variation across species’ geographical ranges: the central-marginal hypothesis and beyond. Molecular Ecology, 17(5): 1170–1188.
Fraser D. 1999. Species at the edge: the case for listing of “peripheral” species. In Proceedings of a Conference on the Biology and Management of Species and Habitats at Risk, Kamloops, British Columbia. Vol. 1, pp. 49–54.
Freeman BG, Lee-Yaw JA, Sunday JM, and Hargreaves AL. 2018. Expanding, shifting and shrinking: the impact of global warming on species’ elevational distributions. Global Ecology and Biogeography, 27(11): 1268–1276
Gaston KJ. 2000. Global patterns in biodiversity. Nature, 405(6783): 220–227.
Gibson S, Van der Marel R, and Starzomski B. 2009. Climate change and conservation of leading-edge peripheral populations. Conservation Biology, 23(6): 1369–1373.
Griffith D, and Peres-Neto P. 2006. Spatial modeling in ecology: the flexibility of eigenfunction spatial analyses. Ecological Society of America, 87(10): 2603–2613.
Hargreaves AL, and Eckert CG. 2019. Local adaptation primes cold-edge populations for range expansion but not warming-induced range shifts. Ecology Letters, 22(1): 78–88.
Hunter ML, and Hutchinson A. 1994. The virtues and shortcomings of parochialism: conserving species that are locally rare, but globally common. Conservation Biology, 8(4): 1163–1165.
IUCN. 2018. The IUCN red list of threatened species. Version 2018-1. IUCN, Gland, Switzerland.
Jenkins CN, Pimm SL, and Joppa LN. 2013. Global patterns of terrestrial vertebrate diversity and conservation. Proceedings of the National Academy of Sciences of the United States of America, 110(28): E2603–E2610.
Kerr JT, and Cihlar J. 2004. Patterns and causes of species endangerment in Canada. Ecological Applications, 14(3): 743–753.
Kerr JT, and Deguise I. 2004. Habitat loss and the limits to endangered species recovery. Ecology Letters, 7(12): 1163–1169.
Lesica P, and Allendorf FW. 1995. When are peripheral populations valuable for conservation? Conservation Biology, 9(4): 753–760.
Mimura M, and Aitken SN. 2010. Local adaptation at the range peripheries of Sitka spruce. Journal of Evolutionary Biology, 23(2): 249–258.
Myers N. 1988. Threatened biotas: “Hot spots” in tropical forests. The Environmentalist, 8(3): 187–208.
Myers N, Mittermeier RA, Mittermeier CG, Fonseca GAB, and Kent J. 2000. Biodiversity hotspots for conservation priorities. Nature, 403(6772): 853–858.
Natural Earth. 2018. Natural earth vector countries. Version 4.1.0 [online]: Available from naturalearthdata.com.
Orme CDL, Davies RG, Burgess M, Eigenbrod F, Pickup N, Olson VA, et al. 2005. Global hotspots of species richness are not congruent with endemism or threat. Nature, 436(7053): 1016–1019.
Pebesma E. 2018. Simple features for R: standardized support for spatial vector data. R Journal, 10(1): 439–446.
Pironon S, Papuga G, Villellas J, Angert AL, García MB, and Thompson JD. 2017. Geographic variation in genetic and demographic performance: new insights from an old biogeographical paradigm. Biological Reviews, 92(4): 1877–1909.
Prendergast JR, Quinn RM, Lawton JH, Eversham BC, and Gibbons DW. 1993. Rare species, the coincidence of diversity hotspots and conservation strategies. Nature, 365(6444): 335–337.
R Core Team. 2018. R: a language and environment for statistical computing.
Rassi P, Hyvärinen E, Juslen A, and Mannerkoski I. 2010. The 2010 red list of finnish species. Ministry of the Environment, Helsinki, Finland.
Reid WV. 1998. Biodiversity hotspots. Trends in Ecology & Evolution, 13(7): 275–280.
Ricketts TH, Dinerstein E, Boucher T, Brooks TM, Butchart SHM, Hoffmann M, et al. 2005. Pinpointing and preventing imminent extinctions. Proceedings of the National Academy of Sciences, 102(51): 18497–18501.
Rodrigues ASL, Akçakaya HR, Andelman SJ, Bakarr MI, Boitani L, Brooks TM, et al. 2004. Global gap analysis: priority regions for expanding the global protected-area network. BioScience, 54(12): 1092.
Sagarin RD, and Gaines SD. 2002. The “abundant centre” distribution: to what extent is it a biogeographical rule? Ecology Letters, 5(1): 137–147.
Samis KE, and Eckert CG. 2009. Ecological correlates of fitness across the northern geographic range limit of a pacific coast dune plant. Ecology, 90(11): 3051–3061.
Schipper J, Chanson JS, Chiozza F, Cox N, Hoffmann M, Katariya V, et al. 2008. The status of the world’s land. Science 322(5899): 225–230.
Schloss CA, Nunez TA, and Lawler JJ. 2012. Dispersal will limit ability of mammals to track climate change in the Western Hemisphere. Proceedings of the National Academy of Sciences, 109(22): 8606–8611
Szabo JK, Khwaja N, Garnett ST, and Butchart SHM. 2012. Global patterns and drivers of avian extinctions at the species and subspecies level. PLoS ONE, 7(10): e47080–e47089.
Thayn JB, and Simanis JM. 2013. Accounting for spatial autocorrelation in linear regression models using spatial filtering with eigenvectors. Annals of the Association of American Geographers, 103: 47–66
Thomas C, Cameron A, Green R, Bakkenes M, Beaumont L, Collingham Y, et al. 2004. Extinction risk from climate change. Nature, 427(6970): 145–148.
Van Rossum F, Vekemans X, Gratia E, and Meerts P. 2003. A comparative study of allozyme variation of peripheral and central populations of Silene nutans L. (Caryophyllaceae) from Western Europe: implications for conservation. Plant Systematics and Evolution, 242(1–4): 49–61.
Venables W, and Ripley B. 2002. Modern applied statistics with S., 4th edition. Springer, New York, New York.
Yakimowski SB, and Eckert CG. 2007. Threatened peripheral populations in context: Geographical variation in population frequency and size and sexual reproduction in a clonal woody shrub. Conservation Biology, 21(3): 811–822.
Supplementary material
Supplementary Material 1 (DOCX / 2.33 MB)
- Download
- 2.33 MB
Information & Authors
Information
Published In
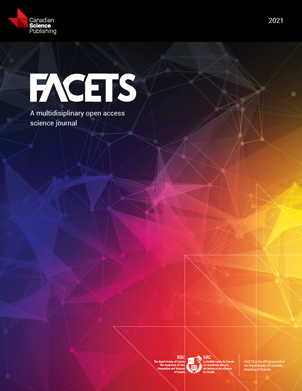
FACETS
Volume 5 • Number 1 • January 2020
Pages: 692 - 703
Editor: Mark Mallory
History
Received: 5 March 2020
Accepted: 26 June 2020
Version of record online: 3 September 2020
Copyright
© 2020 Cameron and Hargreaves. This work is licensed under a Creative Commons Attribution 4.0 International License (CC BY 4.0), which permits unrestricted use, distribution, and reproduction in any medium, provided the original author(s) and source are credited.
Data Availability Statement
Supporting Methods and Results are available in the Supplementary Material. Data extracted from IUCN maps and code for all analyses and Figures are archived online at zenodo.org/record/3972324.
Key Words
Sections
Subjects
Authors
Author Contributions
All conceived and designed the study.
VC collected and analyzed the data.
All interpreted the data and wrote the manuscript.
Competing Interests
The authors have declared that no competing interests exist.
Metrics & Citations
Metrics
Other Metrics
Citations
Cite As
Victor Cameron and Anna L. Hargreaves. 2020. Spatial distribution and conservation hotspots of mammals in Canada. FACETS.
5(1): 692-703. https://doi.org/10.1139/facets-2020-0018
Export Citations
If you have the appropriate software installed, you can download article citation data to the citation manager of your choice. Simply select your manager software from the list below and click Download.
Cited by
1. High conservation importance of range-edge populations of Hooded Vultures (Necrosyrtes monachus)
2. Mapping nationally and globally at-risk species to identify hotspots for (and gaps in) conservation
3. Protecting boreal caribou habitat can help conserve biodiversity and safeguard large quantities of soil carbon in Canada
4. Ten bridges on the road to recovering Canada’s endangered species
5. High conservation priority of range-edge plant populations not matched by habitat protection or research effort