Understanding multifunctional Bay of Fundy dykelands and tidal wetlands using ecosystem services—a baseline
Abstract
We review what is known about ecosystem service (ES) delivery from agricultural dykelands and tidal wetlands around the dynamic Bay of Fundy in the face of climate change and sea-level rise, at the outset of the national NSERC ResNet project. Agricultural dykelands are areas of drained tidal wetland that have been converted to agricultural lands and protected using dykes and aboiteaux (one-way drains or sluices), first introduced by early French settlers (Acadians). Today, Nova Scotia’s 242 km system of dykes protect 17,364 ha of increasingly diverse land uses—including residential, industrial, and commercial uses as well as significant tourism, recreational, and cultural amenities—and is undergoing system modernization and adaptation. Different ES are provided by drained and undrained landscapes such as agriculture from dykelands and regulating services from wetlands, but more complex dynamics exist when beneficiaries are differentiated. This review reveals many knowledge gaps about ES delivery and dynamics, including around net greenhouse gas implications, storm protection, water quality, fish stocks, pollination processes, sense of place, and aesthetics, some of which may reveal shared ES or synergies instead of trade-offs. We emphasize the need to be open to adapting ES concepts and categorizations to fully understand Indigenous implications of these land use decisions.
1. Introduction
In the last few decades, North America has seen increased efforts to conserve and restore coastal habitats (e.g., Zedler 2000; Choi et al. 2008; van Proosdij et al. 2010). The northeastern North American tidal wetland area, including that of the Bay of Fundy system, has been in decline for centuries from dyking, drainage, construction, and erosion (Gedan and Silliman 2009). Agricultural dykelands (also called agricultural marshlands) are areas of drained tidal wetland converted to agriculture using dykes and aboiteaux (one-way drains or sluices). In the 1600s, French settlers (called Acadians) to the Bay of Fundy used this technique to develop high-quality farmland in the Canadian provinces of New Brunswick (NB) and Nova Scotia (NS) (Butzer 2002). Currently, dykes protect agricultural production and a wide range of residential, industrial, and commercial uses owing to farmland abandonment and suburbanization as well as significant tourism and recreation (Sherren et al. 2016).
In many areas, current dyke dimensions are inadequate to protect these coastal land uses, given rising sea levels and storm surges expected with climate change (Lieske 2015; van Proosdij et al. 2018b). Such change and land-use dynamics have removed much of the remnant foreshore tidal wetlands (intact wetlands in front of dykes) that once served to protect the dykes themselves (Fig. 1), a process called coastal squeeze. Moreover, starved of rich sediments from the Bay for so long, many dykelands have subsided, making drainage on them increasingly difficult. The decision-makers that manage the dykes must decide where to reinforce, realign (shorten and pull back), or abandon dykes and related infrastructure. Realignment and abandonment of dykes provides opportunities to restore tidal wetland (Singh et al. 2007). Raising dykes to withstand sea-level rise widens footings, exacerbating coastal squeeze, so it cannot be paired with tidal wetland restoration and may in fact further reduce the extant amount. Early discussions of proposed modifications to individual tracts of dykelands have revealed tensions among governments, landowners, and other citizens, as well as challenges to policy coordination among the many government departments whose responsibilities overlap in these coastal landscapes (Rahman et al. 2019).
Fig. 1.

Reinforcing dykes and aboiteaux to maintain dykelands and restoring wetlands both affect the provision of ecosystem services (ES)—akin to what the Intergovernmental Panel on Biodiversity and Ecosystem Services now calls Nature’s Contributions to People (sensu Díaz et al. 2018)—and, in some cases, result in disservices or unwanted consequences for society. ES are categorized as provisioning/material (e.g., food production), regulating (e.g., water filtration), and cultural/nonmaterial (e.g., recreation), all of which are built upon various amounts of ecosystem processes (supporting ES) and anthropogenic inputs (human and built capitals) (Rist et al. 2014). Disservices, in contrast, comprise reduction in any of these categories of services (Friess et al. 2020). Understanding ES dynamics is critical to informing site-based management decisions to reinforce dykelands or restore wetlands, as each involves trade-offs in services and disservices that often differ by beneficiary.
Wetlands help regulate climate, water storage, erosion, natural hazards, soil formation and nutrient cycles (Mitsch and Gosselink 2000; Mitsch et al. 2013). Mature coastal wetland restoration may provide more effective protection than hard infrastructure (e.g., dykes) against sea-level rise and storm surges and create more resilient coastal communities (Temmerman et al. 2013; Narayan et al. 2017; Van Coppenolle and Temmerman 2019). However, trade-offs with human well-being exist (e.g., dependent on adjacent land management; Chmura et al. 2016), as well as a lag before tidal wetlands deliver the full range of ES for which their restoration is endorsed (Chmura et al. 2012; Smith et al. 2017). Nonmaterial benefits, such as education and recreation, can accrue from wetlands as well (Chen et al. 2020), including for First Nations, whose historical and cultural practices may be particularly linked to such threatened ecosystems (Berkes and Davidson-Hunt 2006).
Alternatively, dykelands can be maintained and (or) raised, allowing for the provision of a suite of ES not limited to agriculture. Despite the natural buffering value of tidal wetlands, many citizens perceive dykes as providing the best storm protection and a range of other anthropogenic services (sensu Rist et al. 2014) that require some human input to achieve (e.g., food provisioning, recreation) (Sherren et al. 2016). The capacity of NS to augment its food supply using dykelands is often discussed in the context of dyke overtopping and resultant flooding of the low-lying Chignecto Isthmus (much of it dykeland) through which most food arrives in the province (Rapaport et al. 2017). The dykelands, dykes, and aboiteaux are still strongly associated with the Acadian culture, which persists in the region despite their forced deportation in the mid-1700s (Desplanque 1982). The region is also an Important Bird Area and a Western Hemisphere Shorebird Reserve Network site, providing an important stopping ground for migratory ducks and shorebirds, the latter declining in number (Rosenberg et al. 2019). While most of the birds are attracted to tidal wetlands, dykes provide access for watching them. Such everyday uses of and attachments to dykes and dykelands drive local resistance to their loss (Sherren et al. 2016).
A new five-year Canadian research network called NSERC ResNet has been initiated for monitoring, modelling, and managing Canadian ES. The project explores the utility of ES thinking for sustainably managing working landscapes—those used to produce food, energy or fibre—given competing land uses and human values. The Nova Scotian Bay of Fundy coast is one of its six case study landscapes. NSERC ResNet will provide our team an opportunity to: (i) fill knowledge gaps about the implications of specific land-use decisions to ecosystem integrity and human well-being, (ii) inform dykeland and tidal wetland decision-making, and (iii) contribute to national ES assessments and techniques. This paper represents a baseline synthesis of our incomplete understanding of point (i) as we initiate our collaboration: what ES are provided by dykelands and tidal wetlands in the Bay of Fundy? Nothing comparable currently exists to inform our work. The complexity of the above research question excluded the possibility of a systematic review. For instance, most of the work we draw upon does not employ ES language and would be missed if we used such keywords to collect relevant material. Instead, we leveraged the shared expertise of a diverse team to develop this narrative review. Research gaps we have identified in the region mean that much of what we present is necessarily inferred from literature from other geographies. Finally, we present a conceptual assessment of ES knowledge and trade-offs; the literature cannot yet support a robust quantitative analysis nor would we consider one especially informative for the full range of services being considered.
We begin with an introduction to the Bay of Fundy dykeland context before summarizing what we know and do not yet know about delivery of final ES along three categories: material (provisioning), regulating, and nonmaterial (cultural). We conclude with a discussion of the more complex dynamics potentially at play in dykeland–wetland decisions, foreshadowing the work of this case study landscape within ResNet. Throughout, we focus on the NS side of the Bay of Fundy where most of our empirical experience and expertise lies; processes and lessons may be applicable elsewhere.
2. The Bay of Fundy dykeland–wetland context
2.1. Land-use history to today’s challenges
Nova Scotia’s coasts have long been occupied. The Mi’kmaq (First Nations) relied on coastal settlements for fishing (Hornborg 2008). Records of early contact with European explorers and fishers along the Atlantic coast date back to the 16th century, but it was in the 1600s that permanent French settlers, later called Acadians, arrived in the Bay of Fundy. The Acadians constructed dykes and aboiteaux to drain coastal wetlands around the Bay of Fundy for agriculture instead of clearing upland forest where soils are relatively shallow (Butzer 2002). Stories exist of the Mi’kmaq helping Acadians with dyke construction (Gerald Gloade Sr., Mi’kmawey Debert Cultural Centre, personal communication 2018), although this may have been to ensure the dyke locations avoided important marsh and creek locations for Mi’kmaq and the ES those sites provided. After the English eventually won the mainland in 1713, many Acadians were deported. The Treaties of Peace and Friendship that the Mi’kmaq signed with the English did not involve the ceding of land rights, but Mi’kmaq were forced onto 13 reserves, many inland (Paul 2006).
The farming and expansion of the rich dykelands were undertaken by the Canadian English, Loyalist New Englanders, who relocated to British territory after the American War of Independence, and later settlers from the European continent. Responsibility for the integrity of the dyke system shifted from landowner cooperatives to government in the mid-20th century through the federal Maritime Marshland Rehabilitation Act of 1948, with responsibility subsequently passed on to the provinces in 1970 (Sherren et al. 2016). Under the Nova Scotia Agricultural Marshland Conservation Act 2000, c. 22, s. 1., the Minister of Agriculture has the powers to: “Reconstruct, repair, operate and maintain any works for the protection, drainage and improvement of marshland for agricultural purposes” (Government of Nova Scotia 2000). These agricultural dykelands remain some of the highest quality farmland in the region. The Nova Scotia Department of Agriculture (NSDA) owns and maintains the dykes and aboiteaux but generally not the land behind. The landowners within each of the 81 administrative units of Nova Scotia dykelands (Fig. 2) are members of Marsh Bodies, incorporated to manage each system in partnership with the NSDA, including deliberating and voting on management changes. Originally built to protect agricultural land and to support farming practices, dykes today are increasingly protecting landscapes that are agriculturally inactive (13.4%) or that are being used for nonagricultural purposes (16.2%) (NSDA 2019).
Fig. 2.

It has been estimated that the Atlantic region has lost over 69% of its coastal tidal wetlands in the Bay of Fundy largely due to dyking (Hanson and Calkins 1996) and construction of causeways and associated tide gates (van Proosdij and Page 2012). In November 2019, there were 242 km of dykes, 252 aboiteaux, and 17,364 hectares and 7473 distinct properties of dykelands in Nova Scotia (NSDA 2019); only 70% of that area remained in agricultural production. The towns of Truro, Amherst, Windsor, and Wolfville are at least partially protected by agricultural dykes. In many coastal communities, the dykes provide flood protection and routes for coastal access, including by walkers, runners, cyclists, birdwatchers, hunters, and fishers; such uses can unintentionally damage dyke infrastructure (Chen et al. 2020). Acadians originally designed agricultural dykes to allow for “tiding”: tidal inundation every few years to avoid subsidence. Modern dykes are not designed this way, so overtopping can threaten the integrity of the protected agricultural lands and infrastructure. NSDA has developed engineering principles for dykeland construction and maintenance in the face of climate futures, as well as a dyke classification based on “consequence of failure” to aid (along with flood mapping) in the prioritization process for dykeland decision-making. The Bay of Fundy dykelands are multifunctional landscapes set within an already dynamic, mega-tidal natural system that is facing increasing challenges as well as demands from a changing climate. NSDA does not presently have the resources to raise all Nova Scotia dykes to heights that would be effective against future sea levels, and in many cases it may not even be technically feasible to keep raising the dykes. As a province, NS must begin to make difficult decisions about which dykes can and should be raised (van Proosdij and Page 2012).
The practice of re-introducing tidal flow to former dykelands, or managed realignment, has been actively performed in many places (French 2001; Bennett et al. 2020). Ducks Unlimited Canada began constructing freshwater wetlands on dykelands or impounding salt marshes with new dykes in the 1980s. In the mid-2000s, regional dyke decommissioning and tidal marsh restoration began, including on some Ducks Unlimited Canada impoundments (Bowron et al. 2012), but the first managed realignment project involving construction of an inner dyke in NS happened in 2018. Such restoration was begun in the Bay of Fundy to decrease the cost of maintaining failing dykes and (or) restore tidal wetland habitat (Singh et al. 2007). Wetland restoration remains primarily a response under provincial and federal wetland policies to compensate for wetland alteration and destruction (for instance by road construction) and increase wetland ES; the NS Wetland Conservation Policy has the primary goal of long-term net gain in salt marsh habitat (Government of Canada 1991; Rubec and Hanson 2009; Government of Nova Scotia 2011). Dykeland can be restored to tidal wetland either by actively dismantling dykes or through passive neglect, or by realigning (pulling back) the dyke where protection needs remain. Creating foreshore wetlands reduces coastal squeeze (Pontee 2013), allowing for more dynamic and naturally regulated shoreline movement and helping to protect infrastructure behind, including any realigned dyke. The restorations undertaken to date represent suitable sites for testing ES supply at various stages of maturity (Bowron et al. 2012; Boone et al. 2017).
2.2. Geographical setting
Local ecosystems and biodiversity are the engines of ES delivery (Close et al. 2009). The structure and functioning of the Bay of Fundy dykelands and tidal wetlands, including their vegetation, climate, hydrology, coastal zone dynamics and geology, determine the ES and disservices at play.
2.2.1. Vegetation
Tidal wetland vegetation in the Bay of Fundy shares a suite of repeating species common across the coast of northeastern North America (Bowron et al. 2012), dominated by native salt-tolerant perennial grasses (Porter et al. 2015). Three main zones are recognized: low marsh (lowest elevation) frequently inundates and is dominated by smooth cordgrass (Spartina alterniflora), high marsh inundates periodically and is dominated by salt hay (Spartina patens) or black grass (Juncus gerardii) and a suite of other native herbaceous perennials, and brackish marsh communities have a range of dominants depending on factors such as disturbance and freshwater influence (Porter et al. 2015).
Dykeland vegetation can be divided into the foreshore, the dyke itself, and the protected area inside the dyke. Foreshore vegetation often contains tidal wetland species, with specific communities largely determined by elevation within the tidal frame and freshwater influence. The dyke itself is covered with perennial upland grasses and is usually mown, with weeds such as wild chervil (Anthriscus sylvestris) and giant hogweed (Heracleum mantegazzianum) controlled with herbicide. Behind the dyke, row crops, hay fields, or pasture represent typical active uses of lands. If left to extended fallow, a variety of “old-field” vegetation types can occur, with soil moisture conditions a likely driver of the types found. This old-field vegetation tends to have a much higher proportion of non-native, diverse plant species than tidal wetlands but is dominated by perennial grasses and forbs. Unmanaged vegetation immediately adjacent to the dykes or between actively farmed areas commonly contains old-field vegetation. Predictable species and successional trajectories for dykelands left fallow are apparent, but published studies of them are lacking.
2.2.2. Climate and hydrology
Relative sea-level rise for the Fundy region is projected to be among the highest in Canada (Manson et al. 2019), and could range from 77 cm to 120 cm by 2100 compared to 1985 (James et al. 2015). Along with this rise comes the added vulnerability of the region to storm surges, which can already overtop some dykes (van Proosdij and Page 2012). It is estimated that 82% of extant Bay of Fundy dykes would be overtopped by a “1 in 50 year” storm by 2050, impacting 808 km of roads (van Proosdij et al. 2018a). In addition, dykes and associated aboiteaux that are meant to protect low-lying coastal lands from tidal flooding can actually exacerbate flooding by restricting freshwater drainage, as is the case with chronic flooding events in Truro, NS (Marvin and Wilson 2016). The Bay of Fundy coast is relatively wet, compared with other places in the region (see, for instance, Rivard et al. 2007), and rainfall events in the region are expected to become more severe and more frequent in future (Richards and Daigle 2011).
Whereas dykes are in place to prevent seawater inundation, periodic inundation of seawater by tides drives tidal wetland functions and the ES they support (e.g., Byers and Chmura 2014). Tidal wetlands are recharged by saline water through periodic flooding of the wetland surface during high tides as well as by freshwater through groundwater, precipitation, and overland runoff (Harvey et al. 1987; Nuttle and Hemand 1988; Byers and Chmura 2014). Tidal inundation, low permeability of the marsh sediment, and their intertidal elevation contribute to shallow water tables, influencing a tidal wetland’s capacity to sequester and store carbon from the atmosphere and maintain elevation in the face of sea-level rise. In contrast, the purpose of dykelands is to prevent tidal flooding, and as such, dykelands have extensive freshwater reserves, fed through precipitation and recharged from uplands.
2.2.3. Coastal zone dynamics
The dynamism of the Bay of Fundy is important to understand for its coastal management. The Bay’s geometry creates tidal resonance, resulting in mega-tidal conditions with the tidal ranges of up to 17 m (Garrett 1972; Godin 1988) and extensive intertidal zones grading from sand to mudflats and vegetated wetland surfaces (Desplanque and Mossman 2004), making them unique in the context of coastal restoration. The Bay of Fundy has a relatively high but spatially variable sediment supply, which is essential in helping its tidal wetlands keep pace with sea-level rise (storm buffering ES) and which influences restoration trajectories. Suspended sediment concentrations range from < 50 mg·L−1 in the lower Bay near Annapolis Royal to greater than 70,000 mg·L−1 in tidal rivers in the Minas Basin near the turbidity maximum (van Proosdij et al. 2018c). Much of this material is resuspended from mud and sand flats by waves, originating from erosion of sedimentary cliffs and foreshore marsh deposits (Wilson et al. 2017). Foreshore marshes, originally stimulated by dyking, typically exhibit patterns of erosion and accumulation at the Basin scale (Ollerhead et al. 2005; van Proosdij et al. 2018c) and are influenced by dykeland management practices and natural processes (e.g., storm activity, sea-level rise, river discharge, meandering tidal rivers) (Matheson 2020). Preservation and enhancement of foreshore marsh is now recognized and accepted as best management practices for decreasing dyke vulnerability (Vuik et al. 2016; van Proosdij et al. 2018c; Vuik et al. 2019).
2.2.4. Geology and hydrogeology
The bedrock geology in the Bay of Fundy region largely determines the availability of water in deeper groundwater systems (aquifers), whereas surficial geology affects shallow groundwater resources and salinized soil dynamics. The surficial geology of the region is characterized by glacial lake deposits (sand, silt, and clay), glacial river deposits (sand and gravel), glacial till, and marine deposits (Rivard et al. 2012). These deposits are generally not productive aquifers for pumping water; however, they play an important hydrogeological role in providing groundwater recharge to deeper bedrock aquifers and also influence saltwater intrusion dynamics. The majority of domestic water supplies in the region have historically been obtained from wells constructed in glacial till (Trescott 1969). However, the towns of Wolfville and Kentville also use surficial sediments as part of their water supply (Rivard et al. 2007). Because Atlantic Canada is at the periphery of the continent, the depression of the ground level due to glacial isostatic adjustment is resulting in the pronounced relative sea-level rise rates for the region (Savard et al. 2016), which in turn influences mixing between the Bay and coastal aquifers as well as the frequency and intensity of dyke overtopping and coastal erosion. In general, understanding the groundwater systems and saltwater flushing dynamics following dyke overtopping is key as fresh groundwater is used extensively in the region for drinking water and irrigation supply.
2.3. Governance, stakeholders, and key actors
Coastal areas in NS are a crowded jurisdictional and occupational space, but also a complex social, cultural, and organizational setting. Many groups play a role in the use and management of the coastal zone, and these can often come into conflict, as well as collaborate and innovate to undertake dyke realignment and (or) tidal wetland restoration (Rahman et al. 2019). Formal governance is complex, including key Provincial departments, such as Agriculture (farming, land protection), Environment (wetland regulation, climate adaptation, coastal protection), Transportation and Infrastructure Renewal (transportation infrastructure), and Lands and Forestry (beaches, hunting, wetland ecology, biodiversity), and at the Federal level the Department of Fisheries and Oceans (fish and fish habitat) and Environment Canada and Climate Change (wetlands, migratory birds). Other important players include departments related to Municipal Affairs (flooding), Aboriginal Affairs (First Nation rights), Acadian Affairs (culture and diaspora), and Communities, Culture and Heritage (tourism, archaeology), as well as nongovernment organizations and nonprofits (e.g., Ecology Action Centre, Ducks Unlimited Canada), and practitioners of coastal restoration (e.g., CBWES Inc., CBCL Limited) (for more fulsome descriptions, see Rahman et al. 2019; Sherren et al. 2019).
While dyke decisions pose a clear challenge to those who live and (or) work on dykelands (e.g., farmers and other Marsh Body members), the question of dykeland futures is perhaps most complicated for members of the Mi’kmaw (First Nations) community because it represents a trade-off between the integrity of the archaeological resources often located in dykelands and the potential restoration of ES that nourish today’s Mi’kmaw culture and society. Mi’kmaw governing bodies are heavily engaged in advocacy for coastal restoration projects and monitoring, including in erstwhile dykelands, with a strong focus on fish passage between ocean and river. At the same time, the Mi’kmaw Rights Initiative (KMKNO, Kwilmu’kw Maw-klusuaqn) has the mandate to protect the archaeological resources often present in dykelands that represent heritage as well as evidence of occupation, and that could potentially be damaged by inundation or earth-moving activities typical of dyke realignment. Debates between stakeholders and rights holders on these issues can be difficult.
Finally, all residents of the Bay of Fundy coast are affected by the decisions to be made, whether they come from Mi’kmaw heritage, Acadian, Planter, or other early or more recent settler groups. Many coastal occupants and land users rely on dykes for their protection, as well as sense of place, recreation, and livelihoods. Media coverage of conversations about dyke realignment and tidal wetland restoration have received mixed responses among affected residents since the first intentional salt marsh restoration project began in the province in 2005 in Cheverie, NS (Bowron et al. 2012). Residents in towns like Hantsport and Advocate Harbour have been recently upset by discussions of possible realignment of dykes or the removal of large tidal barriers near their towns, sometimes so effectively as to weaken political will to carry out such decisions. In other settings, such as Truro, such changes have not yet been controversial (Sherren et al. 2019). Path dependency (when what has been done in the past constrains future options) is not just a technical phenomenon, but a psychological one, as residents anchor in their current landscape norms (Sherren et al. 2017). Robust engagement is critical in such situations, but there has been little social science conducted in the dykelands to inform it.
3. Ecosystem service provision and knowledge gaps
Many ES frameworks are aimed at economic assessments of the environment (de Groot et al. 2012; Davidson et al. 2019), in which coastal wetlands are highly valued in part because of their rarity. There have been local attempts to calculate economic value for our two ecosystems, particularly the wetlands, but this too has suffered from poor local data. One early NS assessment took one of the lower coastal wetland ES values calculated in the United States to calculate annual losses to the province as a result of the estimated 62% of tidal wetlands dyked or otherwise destroyed (19,958 ha or $706 million) and “earnings” from those extant (Wilson 2000). In another study, De Maio Sukic (2001) found that the mean compensation that private landowners on the NB side of the Bay of Fundy would be willing to accept to contribute a hectare of tidal wetland to a conservation program was $1004.22. A decade or so earlier, Stokoe et al. (1989) explored the public willingness to pay for a hypothetical conversion of the Minudie dykeland near Amherst to wetland and found values ranging from $2.50 per ha for nonwetland-using naturalists to $25 by members of the general public who use wetlands. According to the Canadian Environmental Valuation Reference Inventory there has been no work on this financial question in the last two decades in this region, perhaps in part because such valuations have been shown to be so variable and to have little impact on coastal decision-making (Laurans and Mermet 2014; Marre et al. 2016), though it has been applied in similar contexts for managed coastal realignment in the United Kingdom (Luisetti et al. 2011).
We take a more conceptual approach here, inspired by the ES assessment of deltas by Adger et al. (2018). This helps us to follow ES delivery from the elements comprising the two main landscape alternatives—dykelands and wetlands—through a combination of ecosystem processes (otherwise known as supporting ES) and anthropogenic inputs (human and manufactured capital) to specific services (Fig. 3). Some of those linkages are well-understood, and others less so (see dotted lines in Fig. 3). The landforms in question, moving from inland to ocean, include various amounts of dykeland and wetland elements (inside boxes in Fig. 3). A “unit” of dykeland generally comprises: inactive/fallow, cultivated, or grazed dykeland (other dykeland land uses are outside this model); ditches that drain freshwater from it thanks to careful forming of the dykeland; aboiteaux that allow freshwater to escape at low tide and prevent saltwater coming in at high tide; the dyke structure itself; and the borrow pit on the ocean side from which material was excavated to construct it. Tidal wetland is considered to comprise a range of vegetation types from brackish to low marsh (see Section 2.2.1), some of it forming a protective foreshore for the dyke. Conversion from tidal wetland to dykeland is no longer permitted in NS, beyond the wider footings that may accompany dyke raising. Conversion from dykeland to wetland can in rare cases be done by breaking a gap in the dyke and re-engineering natural drainage to the whole area, but it is more often partial, where a new dyke or dyke section is constructed upland from the water to continue to protect land behind while leaving space in front for restoration of tidal flow. A preliminary listing of ES delivery by land-use alternative (Table 1) suggests dyke management decisions will require careful weighing of costs and benefits, over time and space, and for a range of beneficiaries. The wider context of climate change and the Canadian context of reconciliation with Indigenous people over injustices both provide important dimensions to the question of dykeland–wetland futures.
Fig. 3.

Table 1.
Ecosystem service category | Dykeland maintanance | Shared ecosystem services or synergies | Wetland restoration |
---|---|---|---|
Provisioning/material | Food crops and fodder Turf/sod | Fresh groundwater supply (where significant foreshore marsh also present) | Materials for cultural practices Wild food Fish stocks/commercial fishing |
Regulating | — | Overland flood management Erosion control Carbon sequestration Coastal storm protection | Aboveground water quality |
Cultural/immaterial | Access for recreation, learning, and provisioning Settler identity and heritage tourism | Sense of place and aesthetics Relationships and social bonds | Birdwatching and other education/science Mi’kmaw identity and belief system |
3.1. Material ecosystem services
Material services are often treated as a stock of raw materials (i.e., goods) when they are really the reproductive capacity of ecosystems (Farley 2012). In the context of dykelands and tidal wetlands, the key material services are food, freshwater, and biomass. Some of these services overlap with nonmaterial and regulating services discussed later.
Food is clearly provided from dykeland systems in the form of crops and livestock; that is what they are designed for, and today dykelands provide a good share of NS’s highest quality (Class 2) agricultural land (Millward 1999). Actively farmed dykelands (70.7%) are roughly three-quarters field crops and one-quarter pasture (NSDA 2019). Significant development pressures exist on dykelands, particularly those near larger population centres, including farmland abandonment associated with various socio-economic dynamics, such as inheritance and succession issues and the high cost of farmland for new farmers (Clowes 2016). Dykelands may also be used for turf/sod production. As there is more flooding at the low-lying Chignecto Isthmus which serves as a critical transportation route for fresh food, dykelands may be important for future food supply (Rapaport et al. 2017).
Dykeland freshwater resources and dependent crops are threatened by surface and subsurface saltwater intrusion, though little research on these dynamics has been done in the Fundy area (Kennedy 2012). As noted above, large storm events that overtop dykes can cause extensive flooding of dykelands, threatening agricultural productivity and damaging infrastructure (Yang et al. 2013; Elsayed and Oumeraci 2018). Because dykes act as a barrier between land and sea, they can also prevent the seaward drainage of flood water, threatening crop yields and the water quality of irrigation wells (Yang et al. 2013). Dykeland groundwater quality is also threatened by sea-level rise. Even if the dykes are able to protect against the ocean, as sea levels rise, the groundwater table responds by increasing in elevation proportional to the magnitude of sea-level rise (Rotzoll and Fletcher 2013). In low-lying dykelands with shallow water tables, a rising groundwater table may intersect the ground surface, causing complete soil saturation that can be harmful to crops and infrastructure. Furthermore, such saturated soil is prone to flooding. Dykelands are particularly vulnerable to groundwater saturation, as the process of draining wetlands and lowering water tables for agriculture causes organic matter to decompose and land to subside. They are also subsiding because their sediments are no longer recharged from the muddy Bay of Fundy as was occasionally done (intentionally and unintentionally) during early settlement.
Apart from a few wetland species collected for traditional food purposes (e.g., Salicornia spp. also known as pickleweed or samphire), tidal wetland food provision is related to fishing and hunting. Wild food represents provisioning services, but the harvesting activities can be recreational services, depending on circumstances. Little is known about the importance of tidal wetlands to Bay of Fundy commercial or recreational fisheries (e.g., compared to New England (Taylor 2008)), such as striped bass (Morone saxatilis) which are critical to the local gross domestic product, though the nursery function of low marsh is well known (Baker et al. 2016). There are important commercial fisheries for herring (Clupea harengus) and American eel (Anguilla rostrata) in NS as well as an important Indigenous fishery for gaspereau/alewife (Alosa pseudoharengus) and shad (Alosa sapidissima) (Fisheries and Oceans Canada 2018). All such species have been found in various life stages in tidal wetlands, including juvenile stages indicating nursery use (e.g., Eberhardt et al. 2015). Although the habitat used by juvenile lobsters is still somewhat of a mystery, there is evidence that “peat reefs” (chunks of peat colonized by Spartina alterniflora that have broken off from the main salt marsh surface and settled in adjacent subtidal marsh channels) may be important nursery habitats for juvenile lobsters (Able et al. 1988). Given the importance of the lobster fishery in NS, the province’s most valuable seafood export at over $700 million annually (Fisheries and Oceans Canada 2018), the condition of the habitats that support lobsters has important implications for the valuation of ES.
Hunting remains a key activity in North America (Heffelfinger et al. 2013; Arnett and Southwick 2015), contributing approximately 40 billion dollars to the North American economy and helping to sustain waterfowl numbers through collaborations with groups like Ducks Unlimited (Cooper et al. 2015), although hunting’s relative importance is declining compared with various forms of wildlife watching (Hill et al. 2010). In Maritime Canada, dykelands and tidal wetlands alike have a long and storied tradition as key habitats and hunting locations for waterfowl (Rusch et al. 1989; Ringelman et al. 2015), but demographic shifts and urbanization have reduced the number of hunters and the hunting economy in a decades-long pattern of decline (Canadian Wildlife Service Waterfowl Committee 2019).
Beyond the above provisioning services, Mi’kmaq also use tidal wetlands for the collection of many plants for various traditional purposes, but these are not documented in ways that facilitate integration with botanical and ES knowledge (e.g., using different names, oral traditions). Sweetgrass (Hierochloe odorata or Kjimskiku) is used for smudging ceremonies during significant events, and reportedly sea lavender (Limonium) is used for ornamental purposes. In brackish marshes the cattail (Typha sp.) is also a traditional food source (i.e., roots, young shoots, inflorescences), the leaves of which can also be woven to make mats and baskets. Numerous medicinal uses would exist for such tidal wetland plants as well, but such knowledge is carefully managed by Elders, to protect the ecosystems and the culture alike.
3.2. Regulating ecosystem services
Regulating services are those that moderate key environmental processes, maintaining the structure and function of ecosystems around long-term mean conditions (Brander et al. 2013). Indicators of regulating services can be straightforward to select but are challenging to operationalize due to inadequate knowledge on how ecosystem functions generate corresponding services (Reed 1995; Hattam et al. 2015). The regulating services we explore here are pollination, storm protection, flood reduction, erosion control, water filtration, and carbon sequestration. Some of these have relationships with agricultural provisioning (pollination and erosion control), and there are needs for further research in this region.
Wild pollinators of crop plants in NS, such as blueberries, beans, peas, squash, and pumpkins, mainly consist of bees, butterflies, and hoverflies (Larson et al. 2001). The persistence of wild pollinators in agricultural landscapes requires food and nesting resources. Food resources include pollen and nectar produced by crop plants, but most pollinator species require these resources outside of the narrow flowering window of crop plants so adjacent habitats that can provide floral resources at other times of year are crucial (Mallinger et al. 2016; McCallum and McLean 2017). Nesting resources differ by pollinator species, and can include specific larval host plants (e.g., for butterflies), bare soil (e.g., for ground-nesting bees), or woody debris (e.g., for cavity-nesting bees) (Moissett and Buchanan 2010). The earliest ecological accounts suggest that tidal wetlands have low value for pollinators since the most abundant plants are wind-pollinated and do not produce floral resources (Ganong 1903), but pollinator assemblages in tidal wetlands have not been sampled in the region. We expect that high and brackish marsh zones contain the most resources for pollinators as lower inundation rates may allow ground-nesting bees to nest and there is a greater diversity of plant species that are insect-pollinated compared with low marsh zones. New tidal wetland habitat resulting from managed realignment or other restoration activities may also contain dead trees or shrubs that could represent importing nesting resources for wild bees. On dykelands, foreshore vegetation is likely to provide few resources for pollinators, but dyke tops and sides and spontaneous vegetation on the inland side of dykes tend to have a wide variety of plant species that are insect pollinated (Sheffield et al. 2013), so we suspect that dykelands may support larger and more diverse populations of bees and other beneficial insects.
Other than pollination, tidal wetlands are renowned for providing a wide range of regulating services, although wetland restoration may not be valued for these services among some stakeholders. Along with climate change itself, restoration reintroduces saltwater further inland through both surface and subsurface pathways, affecting freshwater resources but on shorter timescales (Willemsen et al. 2020). Wetland vegetation and a range of other biogeochemical processes also remove nutrients, such as anthropogenic nitrogen and phosphorous from land uses, as well as other upland contaminants moving to the Bay (Molnar et al. 2012). With respect to storms and resilience, however, tidal wetland processes help them survive and even thrive under extreme or changing conditions. The dense vegetation of tidal wetlands decreases wave energy, minimizing overland flooding due to storm events (Möller and Spencer 2002; Vuik et al. 2016). Tidal wetlands are resilient to sea-level rise through vertical accretion (build-up) and horizontal migration, which occurs as tidal channels deliver water and suspended sediment to the marsh platform where dense vegetation diminishes water velocity and enables sediment settlement and mineral accumulation (Temmerman et al. 2013; Narayan et al. 2017). While measurements of surface elevation change have been conducted as part of wetland habitat compensation projects (van Proosdij et al. 2010; Bowron et al. 2012), systematic analysis and comparison to natural marshes is limited. This is particularly relevant for sites within the lower Bay of Fundy with lower sediment supply or sites with poor drainage affecting below-ground (root) processes.
Tidal wetlands can sequester large amounts of carbon (C) relative to most terrestrial ecosystems (Mcleod et al. 2011), including freshwater wetlands, a phenomenon also called blue carbon. The role of tidal wetlands as carbon sinks is due to two key features of these ecosystems: (i) C produced elsewhere can be sequestered (captured and stored) by tidal wetlands due to deposition and accretion of the marsh surface over time, and (ii) in situ productivity can be high and decomposition rates tend to be low due to flooding and high salinity. Conversion of tidal wetlands into agricultural land often results in a loss of stored C and a net increase in greenhouse gas emissions (through fertilizer-induced N2 release and enhanced CH4 production in soils; Tan et al. 2020). We expect that tidal wetlands will generally provide more value related to mitigation of climate change than dykelands (Connor et al. 2001; Burden et al. 2013), but local data on C stocks and offsets from non-CO2 greenhouse gases in the diversity of dykeland habitats present in the region are lacking (Roughan et al. 2018).
Research in other regions suggests the value of C sequestration services increases over time in restoring tidal wetlands (Abbott et al. 2019; Burden et al. 2019; O’Connor et al. 2020), so a key knowledge gap in our landscape is the relationship between C storage and time since restoration of tidal flow. The restoration process can cause short-term release of N2O and CH4, both greenhouse gases that counteract the climate benefits, but studies suggest that C storage will outweigh these emissions over the long term (Wollenberg et al. 2018a). The relative contribution of C present in suspended sediment compared with C produced by C fixation by local vegetation is also unknown. While initial reports suggest that plant production on new marshes is a minor contributor to soil organic C stocks (Wollenberg et al. 2018b), we require information from multiple sites, sediment depths, and vegetation types to create a complete account of C stocks in regional tidal wetlands. As plant density and coverage are key determinants of sediment accumulation and local biological production, understanding the dynamics of plant establishment and subsequent decomposition in marsh restoration is also important. We need more research on the abiotic and biotic factors that determine establishment rates of plants during restoration, including the importance of mycorrhizal colonization in allowing tidal wetland grasses to colonize and flourish (Cooke and Lefor 1990; d’Entremont et al. 2019).
Feedbacks between plants and other environmental factors may play a major role in determining carbon sequestration rates as well. Tidal wetland organic matter decomposition is increased by input of nutrients, such as nitrate from agricultural fertilizer use, and this might mean less rather than more burial of organic C rich sediment (Bulseco et al. 2019). It could also reduce the belowground root biomass that stabilizes sediments, increasing wetland susceptibility to coastal erosion (Deegan et al. 2012). In other words, with pollution, tidal wetlands could switch from being C sinks to sources.
3.3. Nonmaterial ecosystem services
Nonmaterial services are by definition intangible and experienced differently by different individuals, a subjectivity that makes it challenging to understand delivery and identify beneficiaries (Daniel et al. 2012; Small et al. 2017). Such services tend to be difficult to enumerate or disentangle, but are key to the formation of sense of place and certainly not immaterial to decision-making in dykeland–wetland settings or elsewhere (Scholte et al. 2016; Margaryan et al. 2018). Changes in them can be deeply felt and fuel resistance to landscape change. Most nonmaterial services are experienced in “bundles”, which means they can foster multi-use, multi-benefit plans that appeal to divergent preferences within the community (Milcu et al. 2013). Neither NS tidal wetlands nor dykelands have been well studied for this purpose.
The easiest nonmaterial services to assess are those that come with a market element that makes them trackable, such as tourism and recreation. For instance, the UNESCO World Heritage Site, Landscape of Grand Pré, brings significant numbers of tourists to the province, including members of the wider Acadian diaspora (George 2013). The agricultural products grown on the dykelands are part of a strong local food and drink movement in the province that attracts numerous visitors to the area for an experience that transcends the material, including benefits like social interaction and the consumption of heritage in the form of “terroir” (Winkler et al. 2017). Wildlife observation, particularly bird-watching, is a multi-billion dollar and growing industry in North America (O’Donnell 2016). For birders, locations that offer a mix of habitats (e.g., coastal marsh, forest, freshwater wetlands, fields) tend to allow people to see more types of species. While birding has potential to be a source of economic development in fine-grained geographies like that of coastal NS (Glowinski 2008), there is a challenge in that birds are often attracted to wetlands but most easily viewed from dykes, which can be eroded through heavy use.
The access benefits that dykes represent along the coast are used by other recreational users (and in some areas, even commuters), including runners, walkers, and cyclists (Chen et al. 2020) as well as motorized users on all-terrain vehicles and dirt bikes and consumptive wildlife users, such as hunters and fishers. Recent work using Instagram to assess nonmaterial benefits in dykelands and tidal wetlands in NS showed that dykes are often used for coastal access (including by school groups) and dykelands are commonly mentioned in photo captions. Consistent with work in North Carolina (Van Berkel et al. 2018), however, the tidal wetland visible in many of the photos is rarely identified as such and thus less clearly understood or valued (Chen et al. 2020). The ES provided by access to natural places, which dyke structures provide in this region, is increasingly recognized as being a key mental, physical, and economic benefit to society (Thompson Coon et al. 2011; Ward Thompson and Aspinall 2011; White et al. 2016). As such, there may be well-being benefits to account for among some individuals and risks in losses of these connections (Cunsolo and Ellis 2018). For others, particularly Mi’kmaw people, the loss of wetlands that help to sustain their livelihoods, cultural practices, and belief systems means the impacts of disconnection are ongoing. It is expected that the boundaries between nonmaterial and other services may be less clear for First Nations people than others, but this is yet to be explored. Such distinctions raise the idea of relational values, which are not entirely instrumental to people (e.g., ES) or intrinsic to an ecosystem, but arise in the interstices of people and nature or between people being together in nature, such as fulfillment from stewardship or shared identity (Chan et al. 2016).
There is much that we do not yet know about the nonmarket, nonmaterial services that dykelands and tidal wetlands might provide and to whom. As difficult as it is to monitor such a diffuse, rural area for its physical uses, it is even harder to understand other values and meanings it might represent. The higher diversity of plant communities found in dykelands may be more aesthetically appreciated by some communities based on visual criteria, including commodities that can generate pleasing linear patterns, such as vineyards and corn. Yet tidal wetlands foster more native plants that may be important to others for food, medicine, or spiritual resources like sweetgrass. The challenge of tracking services becomes even more difficult with the importance of the dykeland system to the identity and culture of the Acadian diaspora (Le Ménestrel 2005; Hodson 2012). A NS survey about dykeland futures was undertaken in 2015, and suggested low attachment to tidal wetlands or their services, but strong attachment to dykelands on the basis of flood protection, food security, recreation, and also for cultural reasons (Sherren et al. 2016). Knowledge gaps abound in all these nonmaterial services and their various sets and scales of beneficiary.
4. Synthesis and conclusions
This baseline exploration of ES delivery by NS dykelands and tidal wetlands reveals broad sets of services (and disservices) and their trade-offs that have been documented in similar landscapes in New England (Taylor 2008) and Europe (Zorrilla-Miras et al. 2014; Karrasch et al. 2019). The overarching theme is one of loss in natural regulating services due to shifts from coastal wetlands to dykelands (Table 1). Trade-offs exists between and within ES categories when considering the specific service (e.g., agriculture versus fisheries) and the beneficiary (e.g., settler versus Mi’kmaq). In a systematic review of over a thousand ES studies from 2000-2013, Howe et al. (2014) established three indications of when trade-offs are likely: (i) private interests in natural resources exist, (ii) provisioning ES are involved, and (iii) local stakeholders are included. The dykeland–wetland challenge includes all three, but transactions associated with dykelands are more likely to be marketized and settler-focused than those associated with wetlands. While the same review indicates that ES trade-offs are three times more likely to be reported than synergies, the latter win-wins are more likely to be reported when three issues are overcome: (i) failure to account for all benefits/stakeholders, (ii) failed management, and (iii) assumptions that provisioning services should dominate.
The most significant failure thus far has been in understanding how Mi’kmaw people benefit from the dykeland and wetland systems, as well as how they conceptualize and approach the issue of adaptation. This is more complex than it may seem on the surface. Though it has become a cornerstone framework for decision-making, researchers have argued that ES might not capture more intangible or relational values of nature (Chan et al. 2016), such as those held by Indigenous communities (Cooper et al. 2016). Among the studies that consider ES in Indigenous contexts comparable to the dykelands, only a minority have used a classic ES approach by monetizing their findings (Konar et al. 2019; Sangha et al. 2019) and the authors acknowledge that monetization cannot fully capture Indigenous-specific nuance. Most studies use ES as a starting point and adapt it to better align with the Indigenous context. Researchers have approached adaptation by incorporating relational values (e.g., Russell et al. 2020), by emphasizing specific aspects of ES that best reflect the Indigenous community’s values (e.g., Robinson et al. 2016), by using theories in addition to ES that foreground the language and experiences of the research participants, or by combining multiple of these approaches (e.g., Satterfield et al. 2013; Levine et al. 2017; Bélisle et al. 2021). Adapting ES to suit the Indigenous research context may better represent and include Indigenous ways of relating to, knowing, and experiencing nature; the importance of such is underscored in the context of reconciliation with Indigenous Peoples over injustices. Such engagement is not only necessary to be a “good” researcher in this context, but also critical to land use change decisions constrained or stimulated by protection of cultural and archeological resources through the NS Special Places Act and Federal Bills C-68 and C-69 that are designed to improve Indigenous engagement and partnership in project reviews and regulatory decisions.
What is suggested here, and described more fully elsewhere (Gómez-Baggethun et al. 2019), is the one-way friction in these landscape changes and the delivery of ES. The conversion from wetland to agriculturally productive dykeland may take a decade, but the trip from dykeland back to fully functioning wetland will be longer. This temporal process is true although Spartina alterniflora can come back surprisingly quickly after flow is restored, meaning it looks like a tidal wetland somewhat before it functions like one. Such dynamics are likely characteristic of shifts between regulating and provisioning services. Other evidence suggests, consistent with work elsewhere (Pedersen et al. 2019), that proximity is important for regulating and nonmaterial service delivery, in particular, but poorly understood. This calls for spatial understanding not only of ecosystems but of beneficiaries to understand chaotic and sometimes idiosyncratic flows of ES (Bagstad et al. 2014).
Dykeland decision-making will have to balance the needs of this range and granularity of beneficiaries from individual to group (Scholte et al. 2016), and recognize the possibility of disbenefits as well as benefits (Rendón et al. 2019), ensuring public support (and thus political support) within the context of reconciliation. At the same time, there remain uncertainties in the restoration process itself given global change; past experiences are not always representative of how an ecosystem will react to a management decision today. It is also important to recognize that dykeland services call for more human input than tidal wetlands systems over the long term, so comparisons should be made with caution. In NSERC ResNet, we will help to fill the knowledge gaps identified above about flows of all three categories of final ES from dykelands and both extant and regenerating tidal wetlands to inform decision-making in the complex Bay of Fundy context (Table 2). Integrating such knowledge is the best chance we have at balancing ecosystem delivery across the whole system, and ensuring a healthy, liveable, and sustainable coast.
Table 2.
Identified knowledge gap | Research stream |
---|---|
Wetland vegetation trajectories after restoration | Planting technique experimentation and implications for ecosystem services lag time |
Fisheries implications of wetlands | Literature review and experimentation to understand fish use |
Storm protection processes and pace in wetlands | Tracking progress of past wetland restorations Quantification of protective services (including resilience to sea-level rise) |
Mi’kmaq ecosystem services across categories | Qualitative engagement with Mi’kmaw communities |
Net greenhouse gas implications, including non-C02 offsets | Sediment and gas-based greenhouse gas investigation, including mycorrhizal fungi |
Non-material services by beneficiaries | Social survey to capture nonmaterial benefits spatially and by beneficiary |
Pollination processes in dykelands and wetlands | Pollinator audits across landscape transects |
Salt water intrusion with sea level rise | Groundwater monitoring and modelling |
Dykeland vegetation trajectories and biodiversity | — |
Acknowledgements
We acknowledge the support of the Natural Sciences and Engineering Research Council of Canada (NSERC), funding reference number NSERC NETGP 523374-18. Cette recherche a été financée par le Conseil de recherches en sciences naturelles et en génie du Canada (CRSNG), [numero de référence CRSNG NETGP 523374-18]. We also acknowledge Graeme Matheson for permission to use Fig. 1. Additional support comes from a grant to DVP from the Canadian Department of Fisheries and Oceans Coastal Restoration Fund. Additional support for NL is from Agriculture and Agri-food Canada and a Nova Scotia Graduate Scholarship. JAG is supported by a National Science Foundation Earth Science Postdoctoral Fellowship (Award 1952627). Thanks to Yan Chen for assistance with the production of Fig. 2.
References
Abbott KM, Elsey-Quirk T, and DeLaune RD. 2019. Factors influencing blue carbon accumulation across a 32-year chronosequence of created coastal marshes. Ecosphere, 10(8): e02828.
Able KW, Heck KL, Fahay MP, and Roman CT. 1988. Use of salt-marsh peat reefs by small juvenile lobsters on Cape Cod, Massachusetts. Estuaries, 11(2): 83.
Adger WN, Adams H, Kay S, Nicholls RJ, Hutton CW, Hanson SE, et al. 2018. Ecosystem services, well-being and deltas: Current knowledge and understanding. In Ecosystem Services for Well-Being in Deltas. Palgrave Macmillan, Cham. pp. 3–27.
Arnett EB, and Southwick R. 2015. Economic and social benefits of hunting in North America. International Journal of Environmental Studies, 72(5): 734–745.
Bagstad KJ, Villa F, Batker D, Harrison-Cox J, Voigt B, and Johnson GW. 2014. From theoretical to actual ecosystem services: mapping beneficiaries and spatial flows in ecosystem service assessments. Ecology and Society, 19(2): 64 [online]: Available from ecologyandsociety.org/vol19/iss2/art64/.
Baker HK, Nelson JA, and Leslie HM. 2016. Quantifying striped bass (Morone saxatilis) dependence on saltmarsh-derived productivity using stable isotope analysis. Estuaries and Coasts, 39(5): 1537–1542.
Bélisle AC, Wapachee A, and Asselin H. 2021. From landscape practices to ecosystem services: Landscape valuation in Indigenous contexts. Ecological Economics, 179: 106858 [online]: Available from sciencedirect.com/science/article/pii/S0921800919318579.
Bennett WG, van Veelen TJ, Fairchild TP, Griffin JN, and Karunarathna H. 2020. Computational Modelling of the impacts of saltmarsh management interventions on hydrodynamics of a small macro-tidal estuary. Journal of Marine Science and Engineering, 8(5): 373.
Berkes F, and Davidson-Hunt IJ. 2006. Biodiversity, traditional management systems, and cultural landscapes: examples from the boreal forest of Canada. International Social Science Journal, 58(187): 35–47.
Boone LK, Ollerhead J, Barbeau MA, Beck AD, Sanderson BG, and McLellan NR. 2017. Returning the tide to dikelands in a macrotidal and ice-influenced environment: challenges and lessons learned. In Coastal Wetlands: Alteration and Remediation. Edited by CW Finkl and C Makowski. Springer International Publishing, Cham. pp. 705–749.
Bowron TM, Neatt N, van Proosdij D, and Lundholm J. 2012. Salt marsh tidal restoration in Canada’s Maritime Provinces. In Tidal Marsh Restoration: A Synthesis of Science and Management. Edited by CT Roman and DM Burdick. Island Press/Center for Resource Economics, Washington, DC. pp. 191–209.
Brander L, Brouwer R, and Wagtendonk A. 2013. Economic valuation of regulating services provided by wetlands in agricultural landscapes: A meta-analysis. Ecological Engineering, 56: 89–96.
Bulseco AN, Giblin AE, Tucker J, Murphy AE, Sanderman J, Hiller‐Bittrolff K, et al. 2019. Nitrate addition stimulates microbial decomposition of organic matter in salt marsh sediments. Global Change Biology, 25(10): 3224–3241.
Burden A, Garbutt A, and Evans C. 2019. Effect of restoration on saltmarsh carbon accumulation in Eastern England. Biology letters, 15(1): 20180773.
Burden A, Garbutt R, Evans C, Jones D, and Cooper D. 2013. Carbon sequestration and biogeochemical cycling in a saltmarsh subject to coastal managed realignment. Estuarine, Coastal and Shelf Science, 120: 12–20.
Butzer KW. 2002. French wetland agriculture in Atlantic Canada and its European roots: different avenues to historical diffusion. Annals of the Association of American Geographers, 92(3): 451–470.
Byers SE, and Chmura GL. 2014. Observations on shallow subsurface hydrology at Bay of Fundy macrotidal salt marshes. Journal of Coastal Research, 30(5): 1006–1016.
Canadian Wildlife Service Waterfowl Committee. 2019. Population Status of Migratory Game Birds in Canada, November 2019. CWS Migratory Birds Regulatory Report Number 52. Canadian Wildlife Service, Ottawa, ON. [online]: Available from canada.ca/en/environment-climate-change/services/migratory-game-bird-hunting/consultation-process-regulations/report-series/population-status-2019.html.
Chan KM, Balvanera P, Benessaiah K, Chapman M, Díaz S, Gómez-Baggethun E, et al. 2016. Opinion: Why protect nature? Rethinking values and the environment. Proceedings of the National Academy of Sciences, 113(6): 1462–1465.
Chen Y, Caesemaecker C, Rahman HMT, and Sherren K. 2020. Comparing cultural ecosystem service delivery in dykelands and marshes using Instagram: A case of the Cornwallis (Jijuktu’kwejk) River, Nova Scotia, Canada. Ocean & Coastal Management, 193: 105254 [online]: Available from sciencedirect.com/science/article/pii/S0964569120301642.
Chmura GL, Burdick DM, and Moore GE. 2012. Recovering salt marsh ecosystem services through tidal restoration. In Tidal Marsh Restoration: A Synthesis of Science and Management. Edited by CT Roman and DM Burdick. Island Press/Center for Resource Economics, Washington, DC. pp. 233–251.
Chmura GL, Kellman L, van Ardenne L, and Guntenspergen GR. 2016. Greenhouse gas fluxes from salt marshes exposed to chronic nutrient enrichment. PLoS ONE, 11(2): e0149937.
Choi YD, Temperton VM, Allen EB, Grootjans AP, Halassy M, Hobbs RJ, et al. 2008. Ecological restoration for future sustainability in a changing environment. Écoscience, 15(1): 53–64 [online]: Available from tandfonline.com/doi/abs/10.2980/1195-6860%282008%2915%5B53%3AERFFSI%5D2.0.CO%3B2.
Close A, Zammit C, Boshier J, Gainer K, and Mednis A. 2009. Ecosystem services: key concepts and applications. Department of the Environment, Water, Heritage and the Arts, Canberra, Australia. [online]: Available from environment.gov.au/biodiversity/publications/ecosystem-services.html.
Clowes L. 2016. Agricultural Land Use Planning in Nova Scotia: A Case Study in Kings County. MES, Dalhousie University.
Connor RF, Chmura GL, and Beecher CB. 2001. Carbon accumulation in Bay of Fundy salt marshes: implications for restoration of reclaimed marshes. Global Biogeochemical Cycles, 15(4): 943–954.
Cooke JC, and Lefor MW. 1990. Comparison of vesicular-arbuscular mycorrhizae in plants from disturbed and adjacent undisturbed regions of a coastal salt marsh in Clinton, Connecticut, USA. Environmental Management, 14(1): 131–137.
Cooper C, Larson L, Dayer A, Stedman R, and Decker D. 2015. Are wildlife recreationists conservationists? Linking hunting, birdwatching, and pro-environmental behavior. The Journal of Wildlife Management, 79(3): 446–457.
Cooper N, Brady E, Steen H, and Bryce R. 2016. Aesthetic and spiritual values of ecosystems: Recognising the ontological and axiological plurality of cultural ecosystem ‘services’. Ecosystem Services, 21: 218–229 [online]: Available from sciencedirect.com/science/article/pii/S2212041616301942.
Cunsolo A, and Ellis NR. 2018. Ecological grief as a mental health response to climate change-related loss. Nature Climate Change, 8(4): 275–281.
d’Entremont TW, López-Gutiérrez JC, and Walker AK. 2019. Examining arbuscular mycorrhizal fungi in saltmarsh hay (Spartina patens) and smooth cordgrass (Spartina alterniflora) in the Minas Basin, Nova Scotia. Northeastern Naturalist, 25(1): 72–86.
Daniel TC, Muhar A, Arnberger A, Aznar O, Boyd JW, Chan KM, et al. 2012. Contributions of cultural services to the ecosystem services agenda. Proceedings of the National Academy of Sciences, 109(23): 8812–8819.
Davidson NC, van Dam AA, Finlayson CM, and McInnes RJ. 2019. Worth of wetlands: revised global monetary values of coastal and inland wetland ecosystem services. Marine and Freshwater Research, 70(8): 1189–1194.
de Groot R, Brander L, van der Ploeg S, Costanza R, Bernard F, Braat L, et al. 2012. Global estimates of the value of ecosystems and their services in monetary units. Ecosystem Services, 1(1): 50–61. [online]: Available from sciencedirect.com/science/article/pii/S2212041612000101.
De Maio Sukic A. 2001. Landowners’ willingness to accept compensation for selling saltwater marshes to a conservation program: a multiple bounded discrete choice approach. M.Sc. thesis, McGill University.
Deegan LA, Johnson DS, Warren RS, Peterson BJ, Fleeger JW, Fagherazzi S, et al. 2012. Coastal eutrophication as a driver of salt marsh loss. Nature, 490(7420): 388–392.
Desplanque C. 1982. Dykelands (polders) along the Bay of Fundy, Canada. In Polders of the World. International Insitutte for Land Reclamation and Improvement/ILR, Wageningen University, Lelystad, The Netherlands. pp. 5–14.
Desplanque C, and Mossman DJ. 2004. Tides and their seminal impact on the geology, geography, history, and socio-economics of the Bay of Fundy, eastern Canada. Atlantic Geology, 40(1): 1–130.
Díaz S, Pascual U, Stenseke M, Martín-López B, Watson RT, Molnár Z, et al. 2018. Assessing nature’s contributions to people. Science, 359(6373): 270–272.
Eberhardt AL, Burdick DM, Dionne M, and Vincent RE. 2015. Rethinking the freshwater eel: salt marsh trophic support of the American eel, Anguilla rostrata. Estuaries and Coasts, 38(4): 1251–1261.
Elsayed SM, and Oumeraci H. 2018. Modelling and mitigation of storm-induced saltwater intrusion: Improvement of the resilience of coastal aquifers against marine floods by subsurface drainage. Environmental Modelling & Software, 100: 252–277 [online]: Available from sciencedirect.com/science/article/pii/S136481521730806X.
Farley J. 2012. Ecosystem services: The economics debate. Ecosystem Services, 1(1): 40–49 [online]: Available from sciencedirect.com/science/article/pii/S2212041612000071.
Fisheries and Oceans Canada. 2018. Seafisheries landings – Atlantic. Government of Canada. [online]: Available from dfo-mpo.gc.ca/stats/commercial/sea-maritimes-eng.htm.
French PW. 2001. Coastal defences: processes, problems and solutions. Routledge Press, London and New York.
Friess DA, Yando ES, Alemu I JB, Lynn-W W, Soto SD, and Bhatia N. 2020. Ecosystem services and disservices of mangrove forests and salt marshes. In Oceanography and marine biology: An annual review. Edited by SJ Hawkins, AL Allcock, AE Bates, AJ Evans, LB Firth, CD McQuaid, et al. Taylor and Francis. pp. 107–142.
Ganong WF. 1903. The vegetation of the Bay of Fundy salt and diked marshes: An ecological study. Botanical Gazette, 36: 161–186 [online]: Available from jstor.org/stable/2465663.
Garrett C. 1972. Tidal resonance in the bay of fundy and gulf of maine. Nature, 238(5365): 441–443.
Gedan KB, and Silliman BR. 2009. Patterns of salt marsh loss within coastal regions of North America. In Human impacts on salt marshes: a global perspective. Edited by BR Sillman, ED Grosholz, and MD Bertness. University of California Press, Berkeley and Los Angeles, California. pp. 253–265.
George EW. 2013. World heritage, tourism destination and agricultural heritage landscape: The case of Grand Pré, Nova Scotia, Canada. Journal of Resources and Ecology, 4(3): 275–284.
Glowinski SL. 2008. Bird-watching, ecotourism, and economic development: a review of the evidence. Applied Research in Economic Development, 5(3): 65–77.
Godin G. 1988. The resonant period of the Bay of Fundy. Continental Shelf Research, 8(8): 1005–1010 [online]: Available from sciencedirect.com/science/article/pii/0278434388900593.
Gómez-Baggethun E, Tudor M, Doroftei M, Covaliov S, Năstase A, Onără D-F, et al. 2019. Changes in ecosystem services from wetland loss and restoration: An ecosystem assessment of the Danube Delta (1960–2010). Ecosystem Services, 39: 100965 [online]: Available from sciencedirect.com/science/article/pii/S2212041617304539.
Government of Canada. 1991. The Federal policy on wetland conservation. Minister of Environment, Ottawa, ON. [online]: Available from nawcc.wetlandnetwork.ca/Federal%20Policy%20on%20Wetland%20Conservation.pdf.
Government of Nova Scotia. 2000. Agricultural Marshland Conservation Act. Acts of the Nova Scotia Legislature. Halifax, NS. [online]: Available from nslegislature.ca/legc/statutes/agricmar.htm.
Government of Nova Scotia. 2011. Nova Scotia Wetland Conservation Policy. Govenment of Nova Scotia, Department of Environment, Halifax, NS. [online]: Available from novascotia.ca/nse/wetland/docs/Nova.Scotia.Wetland.Conservation.Policy.pdf.
Hanson A, and Calkins L. 1996. Wetlands of the Maritime Provinces: revised documentation for the wetlands inventory. Environment Canada, Canadian Wildlife Service, Atlantic Region.
Harvey JW, Germann PF, and Odum WE. 1987. Geomorphological control of subsurface hydrology in the creekbank zone of tidal marshes. Estuarine, Coastal and Shelf Science, 25(6): 677–691 [online]: Available from sciencedirect.com/science/article/pii/0272771487900151.
Hattam C, Atkins JP, Beaumont N, Börger T, Böhnke-Henrichs A, Burdon D, et al. 2015. Marine ecosystem services: linking indicators to their classification. Ecological Indicators, 49: 61–75.
Heffelfinger JR, Geist V, and Wishart W. 2013. The role of hunting in North American wildlife conservation. International Journal of Environmental Studies, 70(3): 399–413.
Hill SG, Cable TT, and Scott D. 2010. Wildlife-based recreation as economic windfall: a rhetorical analysis of public discourse on birding. Applied Environmental Education & Communication, 9(4): 224–232.
Hodson C. 2012. The acadian diaspora: an eighteenth-century history. OUP, USA.
Hornborg A-C. 2008. Mi’kmaq landscapes : from animism to sacred ecology. Ashgate, Aldershot, England
Howe C, Suich H, Vira B, and Mace GM. 2014. Creating win-wins from trade-offs? Ecosystem services for human well-being: A meta-analysis of ecosystem service trade-offs and synergies in the real world. Global Environmental Change, 28: 263–275 [online]: Available from sciencedirect.com/science/article/pii/S0959378014001320.
James TS, Henton JA, Leonard LJ, Darlington A, Forbes DL, and Craymer M. 2015. Tabulated values of relative sea-level projections in Canada and the adjacent mainland United States, Open File 7942. Geological Survey of Canada, Ottawa, ON. [online]: Available from ftp.geogratis.gc.ca/pub/nrcan_rncan/publications/ess_sst/297/297048/of_7942.pdf.
Karrasch L, Klenke T, and Kleyer M. 2019. Land-use elements and attributed ecosystem services: an archetype approach to land-use evaluation at the German North Sea coast. Ecology and Society, 24(2): 13 [online]: Available from ecologyandsociety.org/vol24/iss2/art13/.
Kennedy GW. 2012. Development of a GIS-based approach for the assessment of relative seawater intrusion vulnerability in Nova Scotia, Canada. In International Association of Hydrogeologists Congress, Niagara Falls, ON.
Konar M, Qiu S, Tougher B, Vause J, Tlusty M, Fitzsimmons K, et al. 2019. Illustrating the hidden economic, social and ecological values of global forage fish resources. Resources, Conservation and Recycling, 151: 104456 [online]: Available from sciencedirect.com/science/article/pii/S0921344919303623.
Larson B, Kevan P, and Inouye DW. 2001. Flies and flowers: taxonomic diversity of anthophiles and pollinators. The Canadian Entomologist, 133(4): 439–465.
Laurans Y, and Mermet L. 2014. Ecosystem services economic valuation, decision-support system or advocacy? Ecosystem Services, 7: 98–105 [online]: Available from sciencedirect.com/science/article/pii/S2212041613000843.
Le Ménestrel S. 2005. Connecting past to present: Louisiana cajuns and their sense of belonging to an Acadian diaspora. Nuevo Mundo Mundos Nuevos. [online]: Available from journals.openedition.org/nuevomundo/646.
Levine J, Muthukrishna M, Chan KMA, and Satterfield T. 2017. Sea otters, social justice, and ecosystem-service perceptions in Clayoquot Sound, Canada. Conservation Biology, 31(2): 343–352.
Lieske DJ. 2015. Coping with climate change: the role of spatial decision support tools in facilitating community adaptation. Environmental Modelling & Software, 68(Supplement C): 98–109. [online]: Available from sciencedirect.com/science/article/pii/S1364815215000547.
Luisetti T, Turner RK, Bateman IJ, Morse-Jones S, Adams C, and Fonseca L. 2011. Coastal and marine ecosystem services valuation for policy and management: managed realignment case studies in England. Ocean & Coastal Management, 54(3): 212–224. [online]: Available from sciencedirect.com/science/article/pii/S0964569110001961.
Mallinger RE, Gibbs J, and Gratton C. 2016. Diverse landscapes have a higher abundance and species richness of spring wild bees by providing complementary floral resources over bees’ foraging periods. Landscape Ecology, 31(7): 1523–1535.
Manson GK, Couture NJ, and James TS. 2019. CanCoast 2.0: data and indices to describe teh sensitivity of Canada’s marine coasts to changing climate, Open File 8551. Geological Survey of Canada, Ottawa.
Margaryan L, Prince S, Ioannides D, and Röslmaier M. 2018. Dancing with cranes: a humanist perspective on cultural ecosystem services of wetlands. Tourism Geographies, 1–22.
Marre J-B, Thébaud O, Pascoe S, Jennings S, Boncoeur J, and Coglan L. 2016. Is economic valuation of ecosystem services useful to decision-makers? Lessons learned from Australian coastal and marine management. Journal of Environmental Management, 178: 52–62. [online]: Available from sciencedirect.com/science/article/pii/S0301479716301773.
Marvin J, and Wilson AT. 2016. One dimensional, two dimensional and three dimensional hydrodynamic modeling of a dyked coastal river in the Bay of Fundy. Journal of Water Management Modeling, 25: 404.
Matheson G. 2020. Enhancing dykeland resiliency in a macrotidal estuary. M.Sc. in Applied Science, Saint Mary’s University.
McCallum RS, and McLean NL. 2017. Floral resources and bumble bee abundance in lowbush blueberry field margins. Journal of the Acadian Entomological Society, 13: 37–45.
Mcleod E, Chmura GL, Bouillon S, Salm R, Björk M, Duarte CM, et al. 2011. A blueprint for blue carbon: toward an improved understanding of the role of vegetated coastal habitats in sequestering CO2. Frontiers in Ecology and the Environment, 9(10): 552–560.
Milcu AI, Hanspach J, Abson D, and Fischer J. 2013. Cultural ecosystem services: a literature review and prospects for future research. Ecology and Society, 18(3): 44. [online]: Available from ecologyandsociety.org/vol18/iss3/art44/.
Millward H. 1999. The location of farmland in the maritime provinces: historical, regional, and local perspectives. Saltzburger Geographische Arbeiten, 34: 175–188.
Mitsch W, Bernal B, Nahlik A, Mander Ü, Zhang L, Anderson C, et al. 2013. Wetlands, carbon, and climate change. Landscape Ecology, 28(4): 583–597.
Mitsch WJ, and Gosselink JG. 2000. The value of wetlands: importance of scale and landscape setting. Ecological Economics, 35(1): 25–33. [online]: Available from sciencedirect.com/science/article/pii/S0921800900001658.
Moissett B, and Buchanan S. 2010. Bee basics: an introduction to our native bees. USDA, Forest Service.
Möller I, and Spencer T. 2002. Wave dissipation over macro-tidal saltmarshes: effects of marsh edge typology and vegetation change. Journal of Coastal Research, 36(sp1): 506–521.
Molnar M, Kocian M, and Batker D. 2012. Valuing the aquatic benefits of British Columbia’s lower mainland: Nearshore natural capital valuation. David Suzuki Foundation and Earth Economics, Vancouver and Tacoma. [online]: Available from davidsuzuki.org/wp-content/uploads/2012/11/nearshore-natural-capital-valuation-aquatic-benefits-british-columbia-lower-mainland.pdf.
Narayan S, Beck MW, Wilson P, Thomas CJ, Guerrero A, Shepard CC, et al. 2017. The value of coastal wetlands for flood damage reduction in the northeastern USA. Scientific reports, 7(1): 1–12.
NSDA. 2019. Nova Scotia Provincial Dykelands Summaries, November 11, 2019, based on data from the Agricultural Land Identification Program (ALIP) beta dataset compiled in 2018 by Environment and Agriculture Technology (EAT) group of the Nova Scotia Community College. Nova Scotia Department of Agriculture, Truro, NS.
Nuttle WK, and Hemand HF. 1988. Salt marsh hydrology: Implications for biogeochemical fluxes to the atmosphere and estuaries. Global Biogeochemical Cycles, 2(2): 91–114.
O’Connor JJ, Fest BJ, Sievers M, and Swearer SE. 2020. Impacts of land management practices on blue carbon stocks and greenhouse gas fluxes in coastal ecosystems—A meta‐analysis. Global Change Biology, 26(3): 1354–1366.
O’Donnell M. 2016. Is willingness to pay for non-consumptive wildlife watching falling? Evidence from three rounds of the national survey of fishing, hunting, and wildlife-associated recreation. Human dimensions of wildlife, 21(6): 475–490.
Ollerhead J, Davidson-Arnott RGD, and Scott A. 2005. Cycles of saltmarsh extension and contraction, Cumberland Basin, Bay of Fundy, Canada. In Geomorphologia Littoral I Quaternari: Homenatge al Professor V.M Rossello, I. Verger. Edited by E Sanjaume and JF Mateu. Publicacions Universitat de Valencia. pp. 293–305.
Paul DN. 2006. We were not the savages: collision between European and native American civilizations. 3rd ed. Fernwood Publishing, Halifax, NS.
Pedersen E, Weisner SEB, and Johansson M. 2019. Wetland areas’ direct contributions to residents’ well-being entitle them to high cultural ecosystem values. Science of The Total Environment, 646: 1315–1326. [online]: Available from sciencedirect.com/science/article/pii/S0048969718327335.
Pontee N. 2013. Defining coastal squeeze: A discussion. Ocean & Coastal Management, 84: 204–207 [online]: Available from sciencedirect.com/science/article/pii/S0964569113001786.
Porter C, Lundholm J, Bowron T, Lemieux B, van Proosdij D, Neatt N, et al. 2015. Classification and environmental correlates of tidal wetland vegetation in Nova Scotia, Canada. Botany, 93(12): 825–841.
Rahman H, Sherren K, and van Proosdij D. 2019. Institutional innovation for nature-based coastal adaptation: lessons from salt marsh restoration in Nova Scotia, Canada. Sustainability, 11(23): 6735.
Rapaport E, Starkman S, and Towns W. 2017. Atlantic Canada. In Climate risks and adaptation practices for the Canadian transportation sector 2016 Edited by K Palko and DS Lemmen. Government of Canada, Ottawa, ON. [online]: Available from nrcan.gc.ca/sites/www.nrcan.gc.ca/files/earthsciences/pdf/assess/2016/Chapter-8e.pdf. pp. 218–262.
Reed DJ. 1995. The response of coastal marshes to sea-level rise: Survival or submergence? Earth Surface Processes and Landforms, 20(1): 39–48.
Rendón OR, Garbutt A, Skov M, Möller I, Alexander M, Ballinger R, et al. 2019. A framework linking ecosystem services and human well-being: Saltmarsh as a case study. People and nature, 1(4): 486–496.
Richards W, and Daigle R. 2011. Scenarios and guidance for adaptation to climatechange and sea-level rise—NS and PEI municipalities. Atlantic Climate Adaptation Solutions Association, Halifax, NS. [online]: Available from gov.pe.ca/photos/original/ccscenarios.pdf. 87 p.
Ringelman KM, Williams CK, Devers PK, Coluccy JM, Castelli PM, Anderson KA, et al. 2015. A meta-analysis of American black duck winter habitat use along the Atlantic Coast. The Journal of Wildlife Management, 79(8): 1298–1307.
Rist L, Felton A, Nyström M, Troell M, Sponseller RA, Bengtsson J, et al. 2014. Applying resilience thinking to production ecosystems. Ecosphere, 5(6): 1–11.
Rivard C, Deblonde C, Boivin R, Bolduc A, Paradis SJ, Paradis D, et al. 2007. Canadian groundwater inventory: hydrogeological atlas of the Annapolis Valley, Nova Scotia. Geological Survey of Canada, Open File 5541. Natural Resources Canada, Ottawa, ON. [online]: Available from geoscan.nrcan.gc.ca/starweb/geoscan/servlet.starweb?path=geoscan/fulle.web&search1=R=223781.
Rivard C, Paradis D, Paradis SJ, Bolduc A, Morin RH, Liao S, et al. 2012. Canadian groundwater inventory: regional hydrogeological characterization of the Annapolis Valley aquifers. Geological Survey of Canada, Bulletin 598. Natural Resources Canada, Ottawa, ON. 161 p.
Robinson CJ, James G, and Whitehead PJ. 2016. Negotiating Indigenous benefits from payment for ecosystem service (PES) schemes. Global Environmental Change, 38: 21–29 [online]: Available from sciencedirect.com/science/article/pii/S0959378016300152.
Rosenberg KV, Dokter AM, Blancher PJ, Sauer JR, Smith AC, Smith PA, et al. 2019. Decline of the North American avifauna. Science, 366(6461): 120 [online]: Available from science.sciencemag.org/content/366/6461/120.abstract.
Rotzoll K, and Fletcher CH. 2013. Assessment of groundwater inundation as a consequence of sea-level rise. Nature Climate Change, 3(5): 477–481
Roughan BL, Kellman L, Smith E, and Chmura GL. 2018. Nitrous oxide emissions could reduce the blue carbon value of marshes on eutrophic estuaries. Environmental Research Letters, 13(4): 044034.
Rubec CDA, and Hanson AR. 2009. Wetland mitigation and compensation: Canadian experience. Wetlands Ecology and Management, 17(1): 3–14.
Rusch DH, Ankney CD, Boyd H, Longcore JR, Montalbano F, Ringelman JK, et al. 1989. Population ecology and harvest of the American black duck: a review. Wildlife Society Bulletin (1973-2006), 17(4): 379–406. jstor.org/stable/3782702.
Russell S, Ens E, and Ngukurr Yangbala Rangers. 2020. Connection as country: relational values of billabongs in Indigenous northern Australia. Ecosystem Services, 45: 101169. [online]: Available from sciencedirect.com/science/article/pii/S221204162030111X.
Sangha KK, Stoeckl N, Crossman N, and Costanza R. 2019. A state-wide economic assessment of coastal and marine ecosystem services to inform sustainable development policies in the Northern Territory, Australia. Marine Policy, 107: 103595. [online]: Available from sciencedirect.com/science/article/pii/S0308597X18309837.
Satterfield T, Gregory R, Klain S, Roberts M, and Chan KM. 2013. Culture, intangibles and metrics in environmental management. Journal of Environmental Management, 117: 103–114. [online]: Available from sciencedirect.com/science/article/pii/S0301479712006184.
Savard J-P, van Proosdij D, and O’Carroll S. 2016. Perspectives on Canada’s East Coast region. In Canada’s Marine Coasts in a Changing Climate. Edited by DS Lemmen, FJ Warren, TS James, and CSL Mercer Clarke. Government of Canada, Ottawa, ON. pp. 99–152. [online]: Available from nrcan.gc.ca/sites/www.nrcan.gc.ca/files/earthsciences/pdf/assess/2016/Coastal_Assessment_Chapter4_EastCoastRegion.pdf.
Scholte SSK, Todorova M, van Teeffelen AJA, and Verburg PH. 2016. Public support for wetland restoration: what is the link with ecosystem service values? Wetlands, 36(3): 467–481.
Sheffield CS, Kevan PG, Pindar A, and Packer L. 2013. Bee (Hymenoptera: Apoidea) diversity within apple orchards and old fields in the Annapolis Valley, Nova Scotia, Canada. The Canadian Entomologist, 145(1): 94–114.
Sherren K, Beckley T, Greenland-Smith S, and Comeau L. 2017. How provincial and local discourses aligned against the prospect of dam removal in New Brunswick, Canada. Water Alternatives, 10(3): 697.
Sherren K, Bowron T, Graham JM, Rahman HMT, and van Proosdij D. 2019. Coastal infrastructure realignment and salt marsh restoration in Nova Scotia, Canada. In Responding to Rising Seas: OECD Country Approaches to Tackling Coastal Risks. OECD. pp. 111–135.
Sherren K, Loik L, and Debner JA. 2016. Climate adaptation in ‘new world’cultural landscapes: The case of Bay of Fundy agricultural dykelands (Nova Scotia, Canada). Land Use Policy, 51: 267–280.
Singh K, Walters BB, and Ollerhead J. 2007. Climate change, sea-level rise and the case for salt marsh restoration in the Bay of Fundy, Canada. Environments, 35(2): 71.
Small N, Munday M, and Durance I. 2017. The challenge of valuing ecosystem services that have no material benefits. Global Environmental Change, 44: 57–67. [online]: Available from sciencedirect.com/science/article/pii/S0959378017303540.
Smith JAM, Hafner SF, and Niles LJ. 2017. The impact of past management practices on tidal marsh resilience to sea level rise in the Delaware Estuary. Ocean & Coastal Management, 149: 33–41. [online]: Available from sciencedirect.com/science/article/pii/S0964569117307676.
Stokoe P, Roots J, and Walters B. 1989. Application of wetland evaluation methodologies to the Minudie dykelands, Nova Scotia. Report 5, Wetlands Are Not Wastelands Project. Wildlife Habitat Canada and Environment Canada, Ottawa, ON.
Tan L, Ge Z, Zhou X, Li S, Li X, and Tang J. 2020. Conversion of coastal wetlands, riparian wetlands, and peatlands increases greenhouse gas emissions: A global meta‐analysis. Global Change Biology, 26(3): 1638–1653.
Taylor PH. 2008. Salt marshes in the Gulf of Maine: Human impacts, habitat restoration, and long-term change analysis. Gulf of Maine Council on the Marine Environment.
Temmerman S, Meire P, Bouma TJ, Herman PM, Ysebaert T, and De Vriend HJ. 2013. Ecosystem-based coastal defence in the face of global change. Nature, 504(7478): 79–83.
Thompson Coon J, Boddy K, Stein K, Whear R, Barton J, and Depledge MH. 2011. Does participating in physical activity in outdoor natural environments have a greater effect on physical and mental wellbeing than physical activity indoors? A systematic review. Environmental science & technology, 45(5): 1761–1772.
Trescott PC. 1969. Groundwater resources and hydrogeology of the western Annapolis Valley, Nova Scotia. Report 69-1. Province of Nova Scotia, Department of Mines, Groundwater Section, Halifax, NS. [online]: Available from novascotia.ca/nse/groundwater/docs/GroundwaterResourceReport_WesternAnnapolisValley.pdf.
Van Berkel DB, Tabrizian P, Dorning MA, Smart L, Newcomb D, Mehaffey M, et al. 2018. Quantifying the visual-sensory landscape qualities that contribute to cultural ecosystem services using social media and LiDAR. Ecosystem Services, 31: 326–335. [online]: Available from sciencedirect.com/science/article/pii/S2212041617307714.
Van Coppenolle R, and Temmerman S. 2019. A global exploration of tidal wetland creation for nature-based flood risk mitigation in coastal cities. Estuarine, Coastal and Shelf Science, 226: 106262. [online]: Available from sciencedirect.com/science/article/pii/S0272771418308758.
van Proosdij D, Jahnke R, and Ross C. 2018a. Fundy Agriculture Marshland Flood Maps. NS Department of Agriculture, Land Protection, Nova Scotia, Canada.
van Proosdij D, Lundholm J, Neatt N, Bowron T, and Graham J. 2010. Ecological re-engineering of a freshwater impoundment for salt marsh restoration in a hypertidal system. Ecological Engineering, 36(10): 1314–1332. [online]: Available from sciencedirect.com/science/article/pii/S092585741000162X.
van Proosdij D, and Page S. 2012. Best Management Practices for Climate Change Adaptation in Dykelands: Recommendations for Fundy ACAS sites. Atlantic Climate Adaptations Solutions Association, Halifax, NS.
van Proosdij D, Ross C, and Matheson G. 2018b. Risk proofing Nova Scotia agriculture: Nova Scotia Dyke vulnerability assessment. Nova Scotia federation of agriculture, Bible Hill, NS. 202 p.
van Proosdij D, Ross C, and Matheson G. 2018c. Risk poofing Nova Scotia Agriculture: Nova Scotia Dyke vulnerability assessment. NS Federation of Agriculture, Halifax, NS.
Vuik V, Borsje BW, Willemsen PW, and Jonkman SN. 2019. Salt marshes for flood risk reduction: Quantifying long-term effectiveness and life-cycle costs. Ocean & Coastal Management, 171: 96–110.
Vuik V, Jonkman SN, Borsje BW, and Suzuki T. 2016. Nature-based flood protection: The efficiency of vegetated foreshores for reducing wave loads on coastal dikes. Coastal engineering, 116: 42–56.
Ward Thompson C, and Aspinall PA. 2011. Natural environments and their impact on activity, health, and quality of life. Applied Psychology: Health and Well-Being, 3(3): 230–260.
White M, Elliott L, Taylor T, Wheeler B, Spencer A, Bone A, et al. 2016. Recreational physical activity in natural environments and implications for health: A population based cross-sectional study in England. Preventive Medicine, 91: 383–388.
Willemsen PW, Borsje BW, Vuik V, Bouma TJ, and Hulscher SJ. 2020. Field-based decadal wave attenuating capacity of combined tidal flats and salt marshes. Coastal engineering, 156: 103628.
Wilson EK, Hill PS, van Proosdij D, and Ruhl M. 2017. Coastal retreat rates and sediment input to the Minas Basin, Nova Scotia. Canadian Journal of Earth Sciences, 54(4): 370–378.
Wilson S. 2000. The GPI Water Quality Accounts: Nova Scotia’s Water Resource Values and the Damage Costs of Declining Water Resources and Water Quality. GPI Atlantic, Halifax, NS. [online]: Available from gpiatlantic.org/publications/abstracts/waterquality-ab.htm.
Winkler KJ, Viers JH, and Nicholas KA. 2017. Assessing ecosystem services and multifunctionality for vineyard systems. Frontiers in Environmental Science, 5: 15.
Wollenberg JT, Biswas A, and Chmura GL. 2018a. Greenhouse gas flux with reflooding of a drained salt marsh soil. PeerJ, 6: e5659–e5659. [online]: Available from pubmed.ncbi.nlm.nih.gov/30479881.
Wollenberg JT, Ollerhead J, and Chmura GL. 2018b. Rapid carbon accumulation following managed realignment on the Bay of Fundy. PLOS ONE, 13(3): e0193930.
Yang J, Graf T, Herold M, and Ptak T. 2013. Modelling the effects of tides and storm surges on coastal aquifers using a coupled surface–subsurface approach. Journal of Contaminant Hydrology, 149: 61–75. [online]: Available from sciencedirect.com/science/article/pii/S0169772213000417.
Zedler JB. 2000. Progress in wetland restoration ecology. Trends in Ecology & Evolution, 15(10): 402–407 [online]: Available from sciencedirect.com/science/article/pii/S0169534700019595.
Zorrilla-Miras P, Palomo I, Gómez-Baggethun E, Martín-López B, Lomas PL, and Montes C. 2014. Effects of land-use change on wetland ecosystem services: A case study in the Doñana marshes (SW Spain). Landscape and Urban Planning, 122: 160–174. [online]: Available from sciencedirect.com/science/article/pii/S0169204613001928.
Supplementary material
Supplementary Material 1 (DOCX / 421 KB)
- Download
- 421.63 KB
Information & Authors
Information
Published In
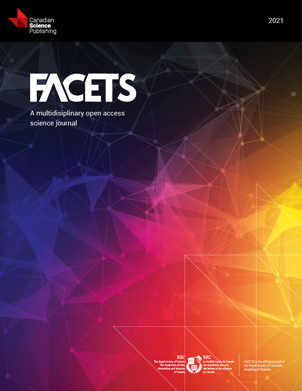
FACETS
Volume 6 • Number 1 • January 2021
Pages: 1446 - 1473
Editor: Evan Fraser
History
Received: 31 August 2020
Accepted: 19 April 2021
Version of record online: 26 August 2021
Notes
This paper is part of a Collection titled “Food, Fiber, Fuel, and Function: Pathways to manage ecosystem services in Canada’s working landscapes”.
Copyright
© 2021 Sherren et al. This work is licensed under a Creative Commons Attribution 4.0 International License (CC BY 4.0), which permits unrestricted use, distribution, and reproduction in any medium, provided the original author(s) and source are credited.
Data Availability Statement
All relevant data are within the paper and in the Supplementary Material.
Key Words
Sections
Subjects
Authors
Author Contributions
KS, KE, BK, JL, MLM, DvP, and AKW conceived and designed the study.
All drafted or revised the manuscript.
Competing Interests
Mark L. Mallory is on the Editorial Board of this journal.
Metrics & Citations
Metrics
Other Metrics
Citations
Cite As
Kate Sherren, Kirsten Ellis, Julia A. Guimond, Barret Kurylyk, Nicole LeRoux, Jeremy Lundholm, Mark L. Mallory, Danika van Proosdij, Allison K. Walker, Tony M. Bowron, John Brazner, Lisa Kellman, B. L. Turner II, and Emily Wells. 2021. Understanding multifunctional Bay of Fundy dykelands and tidal wetlands using ecosystem services—a baseline. FACETS.
6(): 1446-1473. https://doi.org/10.1139/facets-2020-0073
Export Citations
If you have the appropriate software installed, you can download article citation data to the citation manager of your choice. Simply select your manager software from the list below and click Download.
Cited by
1. Enhancing surface drainage mapping in eastern Canada with deep learning applied to LiDAR-derived elevation data
2. Reimagining nature‐based coastal adaptation: A nested framework
3. Landscapes on the edge
4. Mapping wetland habitat health in moribund deltaic India using machine learning and deep learning algorithms
5. Comparing thematic and search term-based coding in understanding sense of place in survey research
6. PCR-Based Amplification of a Cox1 Mini-DNA Barcode Gene from Feces: A Non-Invasive Molecular Technique to Identify Environmental DNA Samples of Maritime Shrew (Sorex maritimensis)
7. The impact of coastal realignment on the availability of ecosystem services: gains, losses and trade-offs from a local community perspective
8. Mega‐Tidal and Surface Flooding Controls on Coastal Groundwater and Saltwater Intrusion Within Agricultural Dikelands
9. Impacts of repeated coastal flooding on soil and groundwater following managed dike realignment
10. Diversity of fungi from marine inundated wood from the Bay of Fundy, Nova Scotia, Canada
11. Disturbance of primary producer communities disrupts the thermal limits of the associated aquatic fauna
12. Modelling cultural ecosystem services in agricultural dykelands and tidal wetlands to inform coastal infrastructure decisions: A social media data approach
13. Vertical Saltwater Intrusion in Coastal Aquifers Driven by Episodic Flooding: A Review
14. Facing the challenges of using place-based social-ecological research to support ecosystem service governance at multiple scales
15. Managing Canada’s land- and seascapes for multiple ecosystem services in the Anthropocene: introduction to the Food, Fiber, Fuel, and Function collection