Microplastic abundance and distribution in the open water and sediment of the Ottawa River, Canada, and its tributaries
Abstract
Microplastic pollution is prevalent in the Ottawa River, with all open water samples (n = 62) and sediment samples (n = 10) containing microplastics. The median microplastic concentration of nearshore 100 L water samples was 0.1 fragments per L (ranged between 0.05 and 0.24 fragments per L). The larger volume Manta trawls samples taken in the middle of the Ottawa River had an overall mean concentration of plastics of 1.35 fragments per m3. Plastic concentrations were significantly higher downstream of the wastewater treatment plant (1.99 fragments per m3) compared with upstream of the effluent output (0.71 fragments per m3), suggesting that the effluent plume is a pathway for plastic pollution to the Ottawa River. The mean concentration of microplastic fragments recovered in the sediment samples was 0.22 fragments per g dry weight. The abundance of microplastics in the sediment was not significantly related to the mean particle size or the organic content of the sediment. The most common form of plastic particles found was microfibers. These made up between 70% and 100% of all plastic particles observed, although plastic microbeads and secondary plastic fragments were also recovered.
Introduction
Plastics are ubiquitous in today’s society, and plastic pollution is one of the defining legacies of humans on the earth. In fact, over the last half century, plastic pollution has been synonymous with human society to the point that it has recently been proposed as a defining feature of the Anthropocene, a newly proposed geological epoch in which humans are a dominant force affecting the earth system (Waters et al. 2016).
In 2015, global plastic production was estimated at 322 million metric tons (Plastics—the Facts 2016), and the vast majority of plastic products are inexpensive and intended to be disposable. This has resulted in massive quantities of plastics being produced and thus disposed of on an annual basis. Plastic polymers do not biodegrade at timescales relevant for human society (i.e., multiple centuries to millennia or longer), but are broken down by UV radiation and mechanical forces into smaller and smaller strands of polymers (Barnes et al. 2009). This breakdown, but lack of biological degradation, results in a buildup of small plastic fragments, which has been most extensively studied in marine environments (Cole et al. 2011). These small plastic particles can have negative impacts on aquatic ecosystems including concentrating other contaminants in the system (Gregory 1996; Rios et al. 2007) and being ingested by animals, including apex predators, reducing their fitness and increasing mortality (Provencher et al. 2015; Sigler 2014; Lönnstedt and Eklöv 2016; Holland et al. 2016).
In aquatic pollution studies, plastic fragments are divided into two main groups based on size: microplastics (≤5 mm) and macroplastics (>5 mm; Masura et al. 2015). Microplastics found in natural systems can come from a variety of sources. Primary microplastics are manufactured by industry; for example, microbeads in cosmetics, toothpaste, and face wash, or microfibers from synthetic clothing and rope (Thompson et al. 2009). Secondary microplastics are the result of the breakdown of larger plastic fragments by erosion, solar radiation, water currents, and freezing and thawing (Barnes et al. 2009).
Microplastic pollution in marine environments is well established (Cole et al. 2011). It is only recently, however, that the presence of microplastics in freshwater systems has been reported (Eriksen et al. 2013; Castañeda et al. 2014; Baldwin et al. 2016), and there is still a limited understanding of where microplastics are occurring in freshwater systems and the controls on their abundance and distribution (Wagner et al. 2014; Anderson et al. 2016). In Canada, few studies have examined plastic pollution of freshwater outside of the Great Lakes/St. Lawrence system, and there is a lack of knowledge of the presence and concentration of plastics in Canadian freshwater systems (Anderson et al. 2016). The goals of this study were threefold. First we quantified the abundance of microplastics in the open water of the Ottawa River and its larger tributaries within the boundaries of the National Capital Region (including the cities of Ottawa, Ontario, and Gatineau, Quebec) to establish microplastic concentrations for this system. We then investigated microplastic pollution upstream and downstream of the city of Ottawa effluent plume in the Ottawa River to test if this effluent plume is a source of microplastics to the Ottawa River. Finally, we quantified the abundance of microplastics in river sediment samples along a depositional gradient to test if microplastic concentrations in the sediments were related to the depositional environment of the river.
Study region
The Ottawa River is a recently designated Canadian Heritage River and holds substantial cultural, ecological, and economic importance to Canada. The river flows for approximately 1271 km, west from northern Quebec into Lake Temiskaming, and then southeast along the border of Ontario and Quebec before emptying into Lake of Two Mountains and the St. Lawrence River in southeastern Quebec. The catchment area of the Ottawa River is 146 300 km2, larger than the size of England, and encompasses large areas of wetlands, boreal forest, and temperate forest in its southern reaches. Sampling took place in Canada’s National Capital Region, which includes the Canadian capital city of Ottawa as well as the neighboring city of Gatineau (Quebec) and smaller towns and rural communities with an approximate population of 1.23 million people.
Methods
Field sampling
To quantify the abundance of microplastics, surface water samples were collected in the summer of 2016 from sampling sites chosen to give a good representation of the Ottawa River watershed within the National Capital Region, both below and above the city of Ottawa’s wastewater treatment plant. These sites included locations along the Ottawa River, Rideau River, Rideau Canal and Brewery Creek, and effluent ponds at the city of Ottawa wastewater treatment plant. Water sampling for plastics was performed using two methods: (1) a nearshore bottle sampling method that filtered 100 L by hand, making it highly portable; and (2) a Manta trawl method that sampled a greater volume of water (∼100 000 L) and was ideal for comparisons with other microplastic research in large lake and marine environments, but challenging to deploy in a variety of shallower sites. The bottle sampling method was performed nearshore in flowing water at a depth of 0.5 m below the water’s surface. At each bottle sampling site, 4 L plastic jugs were used to filter 100 L of water through 100 μm nylon mesh. This was repeated three times at each location. Before use in the field, each filter was examined under a stereomicroscope to ensure that there was no microplastic contamination on the filter and then placed into a sterile Whirl-Pak bag until use. In the field, the filters were carefully removed from the Whirl-Pak bag and placed on the end of a metal cylinder and secured at the base by a hose clamp before water filtration. After each 100 L sample of water was filtered, the filters were carefully removed from the metal cylinder and placed directly back into a Whirl-Pak bag and sealed. Samples were kept refrigerated at 4 °C until processed in the laboratory.
Three 100 L effluent samples were also collected from the city of Ottawa wastewater treatment plant for microplastic analysis. Effluent samples were obtained from the final holding tank at the wastewater treatment plant before the effluent is released into the river. Effluent samples were collected from approximately 15 cm below the surface using an ISCO peristaltic pump and filtered using a 100 μm nylon mesh similar to the bottle sampling method used in the river water sampling.
To sample a larger volume of water specifically upstream and downstream of the effluent plume from the city of Ottawa’s wastewater treatment plant, Manta trawls, using a 100 μm mesh size, were taken from a boat above and below the Ottawa wastewater treatment effluent plume. The Manta net was deployed three times both above and below the effluent plume off the stern of the boat at surface depth for 20 min time intervals. To estimate the volume of water entering the Manta net, the flow of water entering the net was measured in meters per second using a Global Water flow meter. The total volume of water sampled by the Manta net varied between 84 and 181 m3 (mean = 128 m3, standard deviation = 37 m3) and was estimated based on the size of the Manta net opening (area = 0.116 m2), the average flow rate of the sampling interval, and the duration of the sampling interval. Following each Manta trawl, the material retained in the Manta net was washed through a clean 30 μm nylon mesh filter that was then sealed in a Whirl-Pak bag and kept refrigerated until processing. Between each sample, the Manta net was backwashed with river water, and the sock at the end of the Manta net was washed with deionized water in an effort to limit cross-contamination between sampling locations.
To quantify microplastic concentrations in the sediment along a depositional gradient, 10 sediment samples were collected in the fall of 2015 near Petrie Island in the Ottawa River using an Ekman bottom grab sampler with a sample volume of 3.5 L. The sampling site was selected because of its location downstream of the city of Ottawa’s wastewater treatment plant and because there is a large depositional gradient from sandy bottom sediment to fine silts and clays to test if plastic concentrations varied with depositional environment. Between each sample, the Ekman sampler was rinsed with river water and the river sediment was placed in sealed plastic bags and refrigerated until processing in the laboratory.
Laboratory and statistical analyses
In the laboratory, each surface water sample and effluent sample was washed from the mesh filter into a beaker using distilled water and underwent a wet hydrogen peroxide (H2O2) oxidation using a 30% H2O2 solution heated to 80 °C for 7 h to remove organic material from the sample. Following the wet oxidation, the resulting sample was filtered a second time through a clean 100 μm filter. The content in the filter was then backwashed with distilled water into a Petri dish and examined under a Leica stereomicroscope at 40× magnification for microplastics. The microplastics observed were enumerated, and larger fragments were isolated from the sample with forceps for permanent storage. It should be noted that this filtering and visual inspection methodology does not take into account microplastic particles less than 100 μm that would pass through the filter and would have been difficult to reliably identify under a stereomicroscope at 40× magnification.
In addition to freshwater and effluent samples collected in the field, samples of tap water taken from Carleton University were processed in the same manner as the river water samples to serve as control samples for possible contamination with microplastics in the laboratory or during processing. For these control samples, 100 L of tap water were filtered through the same apparatus using the same 4 L Nalgene plastic bottles as the river water samples. These control samples then underwent wet H2O2 oxidation at the same time and location as the field samples using the same equipment. The control samples were then inspected under the stereomicroscope and any microplastic particles observed were counted.
The sediment samples required greater processing than the surface water samples to isolate microplastics because the sediment samples contained much more material retained by the initial sieving. Sediment sample preparation for the isolation of microplastics followed Masura et al. (2015) but omitted the first density separation. A 400 g sample of wet sediment was dried in an oven at 100 °C for 24 h to calculate the dry mass of the sediment. The dried sediment sample was then dispersed using a 5% sodium hexametaphosphate solution and mixed with a magnetic stir bar for 1 h. The dispersed sample was then passed through a 0.3 mm sieve and washed clean. The material retained in the 0.3 mm sieve was transferred to a beaker and underwent wet H2O2 oxidation using 300 mL of 30% H2O2 to remove organic material. The sample material was then sieved through a 100 μm sieve and washed, and the material remaining in the sieve was then transferred to a 500 mL beaker. The sample material then underwent a density separation using a sodium chloride (NaCl) solution of 6 g of salt per 20 mL of sample solution to separate plastic material from the denser sediment (Masura et al. 2015). The salt solution and material were then transferred to a separation funnel and allowed to settle for 30 min. The sediment that settled at the bottom of the separation funnel was then removed, examined for microplastics under a stereomicroscope, and discarded. The solution that remained in the separation funnel was then passed through a 100 μm sieve, thoroughly washed with deionized water to remove all salt, and then backwashed into a Petri dish and examined for microplastics under a Leica stereomicroscope at 40× magnification. Observed microplastic particles were counted, and larger fragments were removed from the Petri dish with forceps and permanently stored.
The organic content and grain size of the sediment were calculated to characterize the depositional environment of the sample site. To determine the organic content of the sediment, a loss-on-ignition analysis was performed using standard methodology (Heiri et al. 2001). Dry sediment (1 cc) was heated in a muffle furnace to 550 °C for 4 h, and the organic content of the sediment was estimated based on the weight loss of the sediment. The mean particle size of the sediment sample was determined using a Coulter laser diffraction analysis conducted on prepared sediment. For particle size analysis, 1 cc of sediment underwent wet H2O2 oxidation at 80 °C for 7 h to remove organic matter. Following oxidation, a 5% sodium hexametaphosphate solution was added to the sample as a dispersant for 24 h before the sample underwent particle size analysis. The arithmetic mean particle size of the sample was calculated using GRADISTAT version 8 (Blott and Pye 2001).
For the Manta net sample, to test if microplastic concentrations were greater downstream from the city of Ottawa effluent plume, a two-sided t test was carried out comparing samples above and below the effluent plume. For the sediment samples, to test if the depositional environment of the system is correlated to microplastic abundance, a nonparametric Spearman’s rank correlation test was performed to compare the abundance of microplastics (number of pieces of microplastic per kg of dry sediment) to the organic matter content and mean particle size of the sediment sample. All statistical analyses and graphing were carried out in R version 3.3.1 (R Development Core Team 2016), and the map was produced using ARCMap version 10.4.1 by Esri.
Results
Microplastics were found in every single surface water sample collected from the Ottawa River and its tributaries (n = 62). With the bottle sampling method, plastic concentrations ranged from a low of 0.05 plastic fragments per L at the confluence of the Madawaska River into the Ottawa River to a high of 0.24 fragments per L in the Rideau River (site a in Fig. 1). The greatest median concentration of plastics was observed in water samples from the Rideau Canal (0.19 fragments per L), followed closely by samples collected at Petrie Island in the Ottawa River (0.18 fragments per L), which is downstream from where the Ottawa wastewater treatment plant releases effluent into the Ottawa River (site h in Fig. 1). The overall median plastic concentration observed for surface water samples using the 100 L filtering technique was 0.1 fragments per L. Interestingly, samples collected directly from the effluent tank using a pump had some of the lowest plastic concentrations observed in all of our samples (median = 0.07, n = 3), suggesting that at the time, plastic concentrations were low at our sampling depth.
Fig. 1.
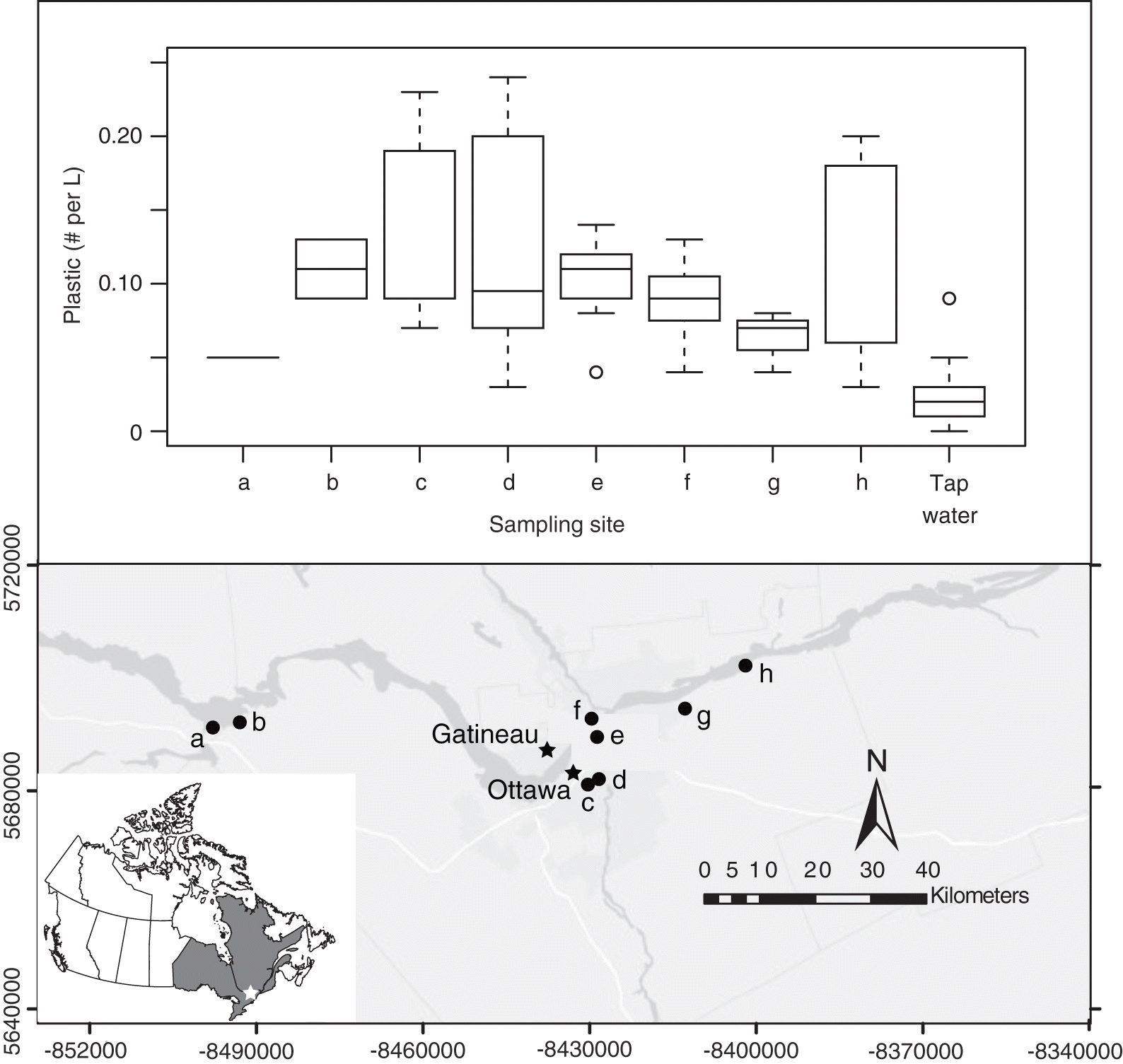
Using the bottle sampling method, not only were plastic fragments detected in all of our surface water samples, but they were also detected in approximately 80% of our tap water samples (9 out of 11 samples). Although the median concentration of plastics in the tap water samples (0.02 fragments per L) is an order of magnitude lower than the median plastic concentration of the field samples (0.1 fragments per L) and there was significantly more plastic in the field samples compared with the tap water controls based on a Kruskal–Wallis test (p < 0.001), these results highlight the potential for the contamination of microplastic samples and the need for laboratory controls to assess the level of contamination.
The vast majority of plastic fragments recovered were microfibers (Fig. 2). Using the bottle sampling method, >95% of plastic fragments recovered were microfibers with the remainder of the sample consisting of microbeads and unidentified plastic fragments. The Manta net sampling was also dominated by plastic microfibers (mean 73% of plastic particles), but there was a greater proportion of microbeads (mean 7% of plastic particles) and other plastic fragments (mean 20% of plastic fragments).
Fig. 2.
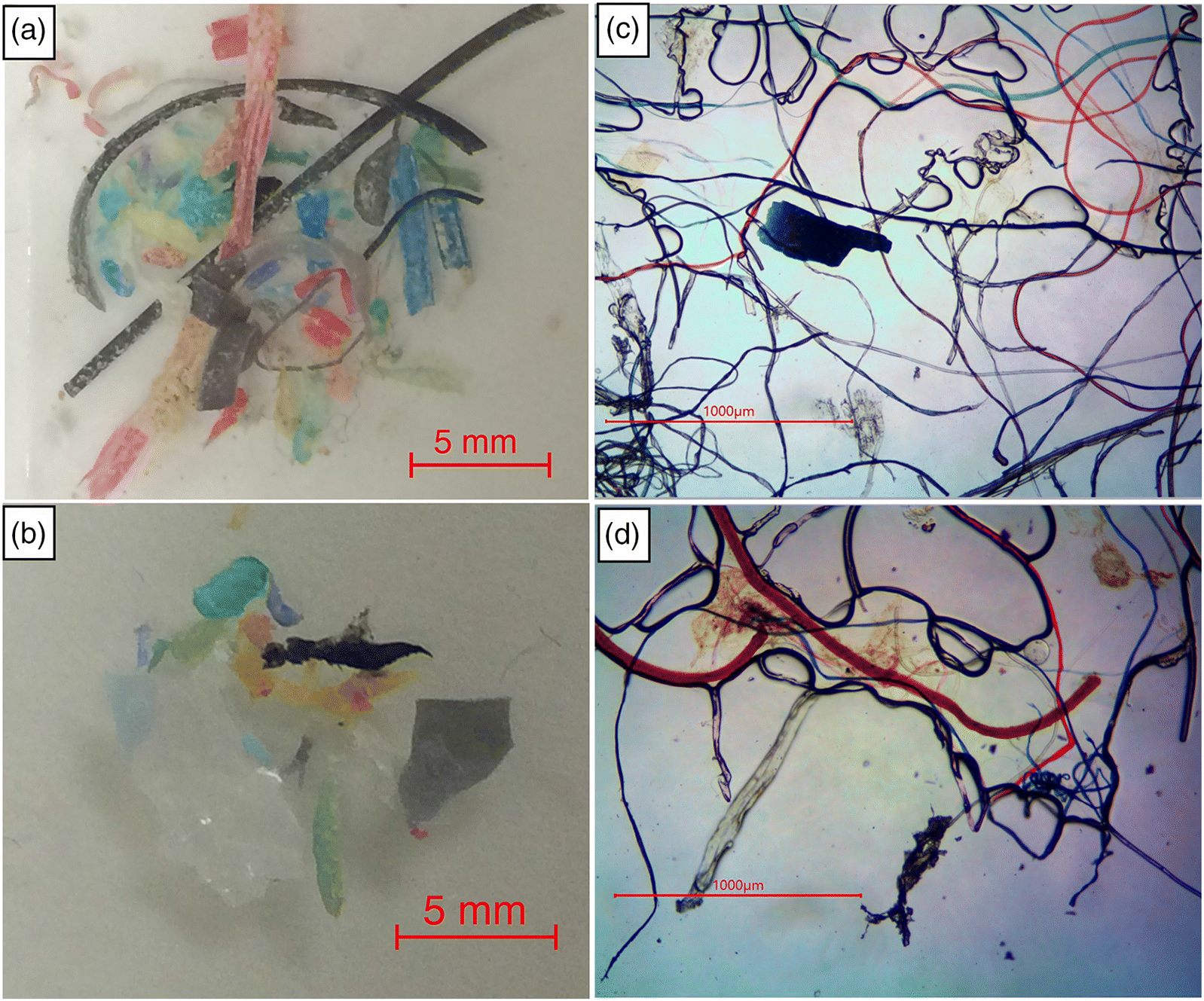
The Manta trawl samples allowed for a much larger volume of water to be filtered than the bottle sampling method, giving a better approximation of plastic concentrations in the open water environment but at a reduced spatial coverage because it requires that the site is accessible by boat. Each 20 min Manta trawl filtered between approximately 84 000 and 180 000 L of water depending on the velocity of the river’s flow and the speed of the boat. Based on the Manta trawls, plastic concentrations in the Ottawa River below the effluent output (1.99 fragments per m3) were 2.8 times greater than plastic concentrations above the effluent output (0.71 fragments per m3) and statistically significant based on a two-sided t test (t = −4.97, df = 3.99, p = 0.008). Based on the Manta trawl sampling, the overall mean concentration of plastics in the open water of the Ottawa River was 1.35 fragments per m3 (Fig. 3). Estimates of plastic concentrations based on the Manta trawl are systematically lower than those estimates based on the bottle sampling method, which may reflect differences in sampling location (e.g., middle of river vs. edge of river) or in sampling methodology (small vs. large volume samples).
Fig. 3.

Microplastics were recovered from every sediment sampling location (Fig. 4). Similar to the open water samples, the microplastics observed in the sediment samples were dominated by microfibers (>95% of fragments), but some microbeads and other plastic fragments were also observed (Fig. 2). The mean concentration of microplastic fragments recovered in all 10 sediment samples was 33 fragments per 400 g wet weight of sediment (or 0.22 fragments per g dry weight). The maximum microplastic abundance observed was 56 fragments per 400 g wet weight (or 0.45 fragments per g dry weight). Although the samples with the greatest microplastic concentrations were those with lower mean particle size and higher organic content, there was no significant correlation between the number of pieces of microplastic per g dry weight or mean grain size (p = 0.51, ρ = −0.24; Fig. 4). The percent organic matter content was also not significantly related to the number of microplastic fragments per g dry weight (p = 0.84, ρ = −0.08; Fig. 4).
Fig. 4.
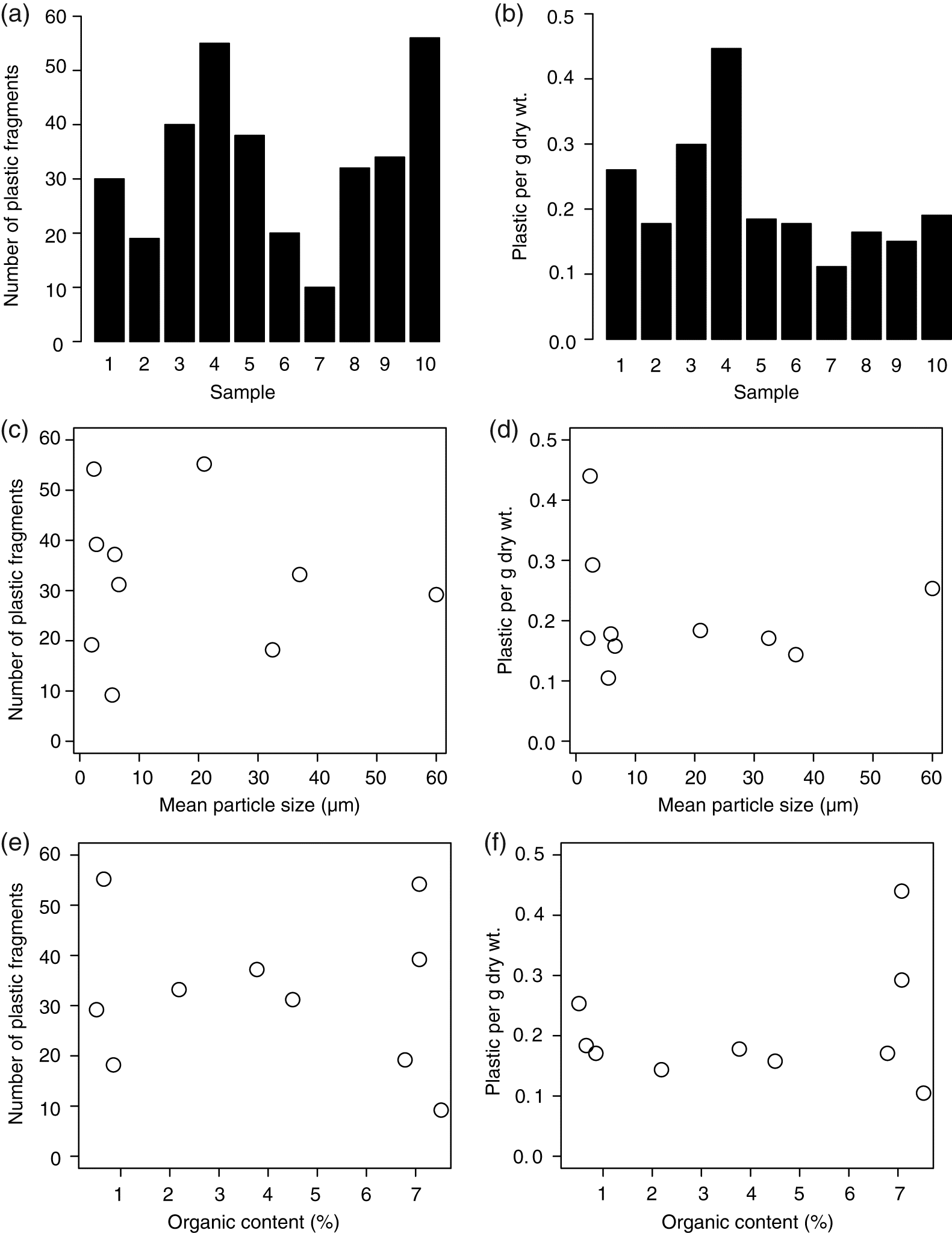
Discussion
Our research shows that plastic pollution is prevalent in the Ottawa River and its tributaries, with microplastics recovered in every water and sediment sample analyzed. Based on Manta net sampling, plastic concentration in the Ottawa River was greater than that reported in the Great Lakes (Eriksen et al. 2013), but less than the tributaries to the Great Lakes or more heavily populated rivers in the United States (Moore et al. 2002; Baldwin et al. 2016) (Fig. 3). Although microplastics were present in every sample, sampling methodology influenced the estimated microplastic concentration of the river, with the smaller volume bottle samples (100 L) taken nearshore being an order of magnitude greater than the open water samples taken with a Manta trawl. Therefore, to compare microplastic pollution among studies it is important that similar methodologies are used. For microplastic concentrations in the sediment, the depositional environment (mean particle size) and organic content of the sediment had little influence on the concentration of plastic in the sediment, suggesting that other factors are important for predicting microplastic deposition in the sediment.
Concentrations of microplastics reported in this study (mean of 1.35 fragments per m3 based on the larger volume Manta net sampling) are greater than have been reported in the Great Lakes (Eriksen et al. 2013), the San Gabriel River in the United States (0.002 fragments per m3; Moore et al. 2002), and the Danube River (0.8 fragments per m3; Lechner et al. 2014), but generally lower than reported in the North Shore Channel in Chicago, Illinois, USA (1.94–17.93 fragments per m3; McCormick et al. 2014), and much lower than has been reported from heavily urbanized California rivers (30–12 000 fragments per m3; Moore et al. 2002). Similar to marine environments, as predicted by Jambeck et al. (2015), given the increasing production and use of plastic, it is likely that plastic pollution of freshwater systems including the Ottawa River will increase in the future.
The types of plastic fragments, dominated by red and blue microfibers, found in the sediment samples resembled what was recovered in the open water sampling, suggesting that the microplastics in the sediment settled out of the water column despite the plastics being less dense than freshwater. It may be that microplastics in the open water environment aggregate together with particulate organic and settle out of the water column. Comparing the abundance of microplastics recovered in the Ottawa River sediment with other freshwater studies is somewhat complicated by the unit of measure. We found a mean of 0.22 plastic fragments per g dry weight in the Ottawa River, which is comparable with what has been reported in German rivers (0.34–0.64 fragments per g dry weight; Wagner et al. 2014). Other freshwater studies of microplastics in sediment have tended to express their values on an areal basis (Anderson et al. 2016), but as an approximate comparison, our values appear greater than those from Great Lake sediments 0–34 fragments per m2 (Zbyszewski et al. 2014) but lower than sediments from the St. Lawrence River (mean of 13 822 fragments per m2; Castañeda et al. 2014).
The concentration of microplastics recovered in the sediment samples was not significantly related to either the sediment particle size or the organic content of the sediment. It therefore appears that the depositional environment has little influence on the concentration of microplastics settling out of the water column into the sediment. It may be that the majority of these plastic particles are settling out of the water in larger aggregates of microplastics mixed with particulate organic and inorganic material, giving the aggregate a greater density and allowing the plastic to settle out under greater water velocities, although this would need to be examined in another study. An additional possibility is that our sample size was too small to give us the statistical power to detect a significant trend given the variability in the data. A post hoc power analysis indicated that a sample size of 60 would be required to adequately test the effect of depositional environment on microplastic concentrations given the variability observed in this study.
Although the impact of microplastics on freshwater ecosystems is poorly understood (Wagner et al. 2014; Anderson et al. 2016), it is clear that some freshwater organisms are consuming this plastic pollution (Holland et al. 2016), and given what has been observed in marine environments (Provencher et al. 2015; Lönnstedt and Eklöv 2016) it is plausible that they are having some detrimental impacts on freshwater organisms. Few toxicological studies have investigated the impact of microplastics on freshwater organisms and this remains a major knowledge gap in our assessment of water quality for the protection of aquatic life (Anderson et al. 2016). Au et al. (2015) investigated the impact of microplastics on Hyalella azteca, an amphipod crustacean, and found that microfibers were significantly more toxic than microbeads to the organism due to the longer residence time of the fibers in the gut of the organism, although all forms of microplastic displayed a toxic effect. This is worrying given that the vast majority of plastic particles recovered in our study were microfibers, but at concentrations that were orders of magnitude lower than those used by Au et al. (2015) in their acute and chronic exposure experiments. The greater prevalence and toxicity of plastic microfibers is also a concern because this form of plastic pollution is even more difficult to control than secondary plastics and microbeads, whose concentrations may be partially reduced through improved waste management and public policy phasing out the use of microbeads in cosmetics. Microfibers on the other hand come from multiple sources (Browne et al. 2011) and are easily transported into aquatic system both by runoff and by atmospheric deposition (Browne et al. 2011; Gasperi et al. 2015).
It is also of note that many of our tap water control samples also had a small amount of microplastic in the sample (one or two fibers per 100 L filtered). It is plausible that these fibers were in the tap water and are not a source of contamination, as microplastic pollution has even been reported in beer purchased in a German supermarket (Liebezeit and Liebezeit 2014). The city of Ottawa gets its drinking water from the Ottawa River; however, the city filters their drinking water through a 1.0 μm filter, which should remove all but the smallest plastic fragments from the river water. Although post-filter contamination of the drinking water with microplastics is entirely plausible, another possibility is that these samples became contaminated with small amounts of microplastics during the sampling or in the lab. Although efforts were taken to prevent contamination during the sampling and in the lab (using pre-inspected filters, sterile bags, and always keeping samples covered), microplastic fibers are common in the air and atmosphere, with indoor air samples reporting microplastic fiber concentrations in the range of 3–15 particles per m3 (Gasperi et al. 2015). It is an important point that the microplastics reported in air quality testing were always microfibers (Gasperi et al. 2015), which were the only types of plastic particles observed in our tap water samples. Due to the high prevalence of microplastic fibers in the air, it is important when sampling aquatic microplastics to limit open contact with the air as much as possible to limit contamination. It is also important to consider that smaller sample sizes are more prone to having large relative errors due to lab contamination by a single microplastic fiber. Therefore, whenever possible, large volume samples should be employed to get the best estimate of microplastic concentrations in the system. To account for possible contamination, we recommend running control samples such as the tap water samples used in this study. This should provide a representative background contamination level based on plastic concentrations at the sampling site and in the laboratory’s air.
Conclusions
Our study has shown that plastic pollution of the Ottawa River and its major tributaries in Canada’s National Capital Region is prevalent and at concentrations greater than have been reported for many other freshwater systems including the Great Lakes. Our study contributes to a growing body of research (reviewed by Wagner et al. 2014 and Anderson et al. 2016) that suggests that microplastic pollution of freshwater ecosystems may be ubiquitous. It is therefore important that we develop reliable and repeatable methods for sampling and the reporting of microplastic pollution, and that we increase our understanding of the spatial and temporal variability of plastic pollution in freshwater systems. In addition, the effects of microplastic pollution, if any, on freshwater ecosystems and human health remain an important knowledge gap in our management of water quality for the protection of aquatic life.
Acknowledgements
We would like to thank Brian Bezaire for making the wastewater effluent sampling possible. This research was supported by an NSERC Discovery Grant to JCV, an NSERC USRA to CP, and a Carleton University Deans Summer Research Internship to SMH. The Ottawa Wavemakers (Hub Ottawa and WWF), Evergreen Freshwater Grant Program and Muskoka Brewery, TD Friends of Environment Foundation, and The 5 Gyres Institute provided support to MM.
References
Anderson JC, Park BJ, and Palace VP. 2016. Microplastics in aquatic environments: implications for Canadian ecosystems. Environmental Pollution, 218: 269–280.
Au SY, Bruce TF, Bridges WC, and Klaine SJ. 2015. Responses of Hyalella azteca to acute and chronic microplastic exposures. Environmental Toxicology and Chemistry, 34(11): 2564–2572.
Baldwin AK, Corsi SR, and Mason SA. 2016. Plastic debris in 29 Great Lakes tributaries: relations to watershed attributes and hydrology. Environmental Science & Technology, 50(19): 10377–10385.
Barnes DKA, Galgani F, Thompson RC, and Barlaz M. 2009. Accumulation and fragmentation of plastic debris in global environments. Philosophical Transactions of the Royal Society B: Biological Sciences, 364(1526): 1985–1998.
Blott SJ, and Pye K. 2001. GRADISTAT: a grain size distribution and statistics package for the analysis of unconsolidated sediments. Earth Surface Process and Landforms, 26(11): 1237–1248.
Browne MA, Crump P, Niven SJ, Teuten E, Tonkin A, Galloway T, et al. 2011. Accumulation of microplastic on shorelines worldwide: sources and sinks. Environmental Science & Technology, 45(21): 9175–9179.
Castañeda RA, Avlijas S, Simard MA, and Ricciardi A. 2014. Microplastic pollution in St. Lawrence River sediments. Canadian Journal of Fisheries and Aquatic Sciences, 71(12): 1767–1771.
Cole M, Lindeque P, Halsband C, and Galloway TS. 2011. Microplastics as contaminants in the marine environment: a review. Marine Pollution Bulletin, 62(12): 2588–2597.
Collignon A, Hecq J-H, Galgani F, Collard F, and Goffart A. 2014. Annual variation in neustonic micro- and meso-plastic particles and zooplankton in the Bay of Calvi (Mediterranean-Corsica). Marine Pollution Bulletin, 79(1–2): 293–298.
Dris R, Imhof H, Sanchez W, Gasperi J, Galgani F, Tassin B, et al. 2015. Beyond the ocean: contamination of freshwater ecosystems with (micro-)plastic particles. Environmental Chemistry, 12(5): 539–550.
Eriksen M, Mason S, Wilson S, Box C, Zellers A, Edwards W, et al. 2013. Microplastic pollution in the surface waters of the Laurentian Great Lakes. Marine Pollution Bulletin, 77(1–2): 177–182.
Faure F, Corbaz M, Baecher H, and de Alencastro LF. 2012. Pollution due to plastics and microplastics in Lake Geneva and in the Mediterranean Sea. Archives des Sciences, 65(1): 157–164.
Gasperi J, Dris R, Mirande-Bret C, Mandin C, Langlois V, and Tassin B. 2015. First overview of microplastics in indoor and outdoor air [online]. Available from https://hal-enpc.archives-ouvertes.fr/hal-01195546.
Gilffilan LR, Ohman MD, Doyle MJ, and Watson W. 2009. Occurrence of plastic micro-debris in the Southern California current system. California Cooperative Oceanic Fisheries Investigations Reports, 50: 123–133.
Goldstein MC, and Goodwin DS. 2013. Gooseneck barnacles (Lepas spp.) ingest microplastic debris in the North Pacific Subtropical Gyre. PeerJ, 1: e184.
Gregory MR. 1996. Plastic ‘scrubbers’ in hand cleansers: a further (and minor) source for marine pollution identified. Marine Pollution Bulletin, 32(12): 867–871.
Heiri O, Lotter AF, and Lemcke G. 2001. Loss on ignition as a method for estimating organic and carbonate content in sediments: reproducibility and comparability of results. Journal of Paleolimnology, 25(25): 101–110.
Holland ER, Mallory ML, and Shutler D. 2016. Plastics and other anthropogenic debris in freshwater birds from Canada. Science of the Total Environment, 571: 251–258.
Jambeck JR, Geyer R, Wilcox C, Siegler TR, Perryman M, Andrady A, et al. 2015. Marine pollution. Plastic waste inputs from land into the ocean. Science, 347(6223): 768–771.
Law KL, Morét-Ferguson S, Maximenko NA, Proskurowski G, Peacock EE, Hafner J, et al. 2010. Plastic accumulation in the North Atlantic Subtropical Gyre. Science, 329(5996): 1185–1188.
Lechner A, Keckeis H, Lumesberger-Loisl F, Zens B, Krusch R, Tritthart M, et al. 2014. The Danube so colourful: a potpourri of plastic litter outnumbers fish larvae in Europe’s second largest river. Environmental Pollution, 188: 177–181.
Liebezeit G, and Liebezeit E. 2014. Synthetic particles as contaminants in German beers. Food Additives and Contaminants. Part A, Chemistry, Analysis, Control, Exposure & Risk Assessment, 31(9): 1574–1578.
Lönnstedt OM, and Eklöv P. 2016. Environmentally relevant concentrations of microplastic particles influence larval fish ecology. Science, 352(6290): 1213–1216.
Magnusson K, and Norén F. 2014. Screening of microplastic particles in and down-stream a wastewater treatment plant. Report number C55 for IVL Swedish Environmental Research Institute Ltd.
Mani T, Hauk A, Walter U, and Burkhardt-Holm P. 2015. Microplastics profile along the Rhine River. Scientific Reports, 5: 17988.
Masura J, Baker J, Foster G, and Arthur C. 2015. Laboratory methods for the analysis of microplastics in the marine environment: recommendations for quantifying synthetic particles in waters and sediments. NOAA Technical Memorandum NOS-OR&R-48.
McCormick A, Hoellein TJ, Mason SA, Schluep J, and Kelly JJ. 2014. Microplastic is an abundant and distinct microbial habitat in an urban river. Environmental Science & Technology, 48(20): 11863–11871.
Moore CJ, Moore SL, Weisberg SB, Lattin GL, and Zellers AF. 2002. A comparison of neustonic plastic and zooplankton abundance in southern California’s coastal waters. Marine Pollution Bulletin, 44(10): 1035–1038.
Moore CJ, Lattin GL, and Zellers AF. 2011. Quantity and type of plastic debris owing from two urban rivers to coastal waters and beaches of Southern California. Journal of Integrated Coastal Zone Management, 11(1): 65–73.
Plastics—the Facts. 2016. An analysis of European plastics production, demand and waste data [online]. PlasticsEurope, 35 p. Available from http://www.plasticseurope.org/documents/document/20161014113313-plastics_the_facts_2016_final_version.pdf.
Provencher JF, Bond AL, and Mallory ML. 2015. Marine birds and plastic debris in Canada: a national synthesis and a way forward. Environmental Reviews, 23(1): 1–13.
R Development Core Team. 2016. R: a language and environment for statistical computing. R Foundation for Statistical Computing, Vienna, Austria [online]: Available from http://www.R-project.org.
Rios LM, Moore C, and Jones PR. 2007. Persistent organic pollutants carried by synthetic polymers in the ocean environment. Marine Pollution Bulletin, 54(8): 1230–1237.
Sadri SS, and Thompson RC. 2014. On the quantity and composition of floating plastic debris entering and leaving the Tamar Estuary, Southwest England. Marine Pollution Bulletin, 81(1): 55–60.
Sigler M. 2014. The effects of plastic pollution on aquatic wildlife: current situations and future solutions. Water, Air, & Soil Pollution, 225(11): 2184.
Thompson RC, Swan SH, Moore CJ, and vom Saal FS. 2009. Our plastic age. Philosophical Transactions of the Royal Society B: Biological Sciences, 364(1526): 1973–1976.
Wagner M, Scherer C, Alvarez-Muñoz D, Brennholt N, Bourrain X, Buchinger S, et al. 2014. Microplastics in freshwater ecosystems: what we know and what we need to know. Environmental Sciences Europe, 26(1): 12.
Waters CN, Zalasiewicz J, Summerhayes C, Barnosky AD, Poirier C, Gałuszka A, et al. 2016. The Anthropocene is functionally and stratigraphically distinct from the Holocene. Science, 351(6269): aad2622.
Zbyszewski M, Corcoran PL, and Hockin A. 2014. Comparison of the distribution and degradation of plastic debris along shorelines of the Great Lakes, North America. Journal of Great Lakes Research, 40(2): 288–299.
Information & Authors
Information
Published In
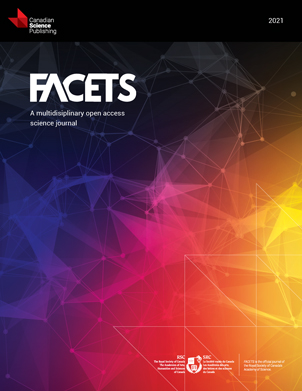
FACETS
Volume 2 • Number 1 • January 2017
Pages: 301 - 314
Editor: Daniel E. Schindler
History
Received: 28 November 2016
Accepted: 26 January 2017
Version of record online: 21 March 2017
Copyright
© 2017 Vermaire et al. This work is licensed under a Creative Commons Attribution 4.0 International License (CC BY 4.0), which permits unrestricted use, distribution, and reproduction in any medium, provided the original author(s) and source are credited.
Data Availability Statement
All relevant data are within the paper.
Key Words
Sections
Subjects
Plain Language Summary
Microplastic pollution in Canada’s capital region
Authors
Author Contributions
All conceived and designed the study.
All performed the experiments/collected the data.
JCV and MM analyzed and interpreted the data.
JCV and MM contributed resources.
JCV, CP, and MM drafted or revised the manuscript.
Competing Interests
The authors have declared that no competing interests exist.
Metrics & Citations
Metrics
Other Metrics
Citations
Cite As
Jesse C. Vermaire, Carrington Pomeroy, Sofia M. Herczegh, Owen Haggart, and Meaghan Murphy. 2017. Microplastic abundance and distribution in the open water and sediment of the Ottawa River, Canada, and its tributaries. FACETS.
2: 301-314.
https://doi.org/10.1139/facets-2016-0070
Export Citations
If you have the appropriate software installed, you can download article citation data to the citation manager of your choice. Simply select your manager software from the list below and click Download.
Cited by
1. A global review of river sediment contamination and remobilization through climate change-induced flooding
2. The entrainment of polyester microfibers modifies the structure and function of periphytic biofilms
3. Microplastic distribution and composition in mudflat sediments and varnish clams (Nuttallia obscurata) at two estuaries of British Columbia, Canada: An assessment of potential anthropogenic sources
4. Presence of microplastics during high rainfall events in the Cauvery River (South India): Ecological risk and cultural practices
5. Quantifying Effects and Ingestion of Several Pristine Microplastics in Two Early Life Stages of Freshwater Mussels
6. Seasonal and Distributional Changes in the Composition and Flux of Anthropogenic Microparticles in the Surface Waters of the Charles River, Massachusetts, United States
7. Microplastics in Farmed Animals—A Review
8. Inter riverine variability in microplastic distribution among two tropical rivers – Chalakudy and Periyar, Southwest India
9. Microplastic pollution in the surface waters of the zuari river, goa, india
10. Fibrous microplastics in the environment: Sources, occurrence, impacts, and mitigation strategies
11. Microplastic pollution in the North-east Atlantic Ocean surface water: How the sampling approach influences the extent of the issue
12. Modeling the settling and resuspension of microplastics in rivers: Effect of particle properties and flow conditions
13. Microplastic evidence assessment from water and sediment sampling in a shallow tropical lake
14. Distribution, flux, and risk assessment of microplastics at the Anzali Wetland, Iran, and its tributaries
15. Distribution and effects of microplastics as carriers of heavy metals in river surface sediments
16. Microplastics monitoring in freshwater systems: A review of global efforts, knowledge gaps, and research priorities
17. An Analysis of Suspected Microplastics in the Muscle and Gastrointestinal Tissues of Fish from Sarasota Bay, FL: Exposure and Implications for Apex Predators and Seafood Consumers
18. Sediment profiles and recording the effects of anthropogenic activities
19. Unveiling Small-Sized Plastic Particles Hidden behind Large-Sized Ones in Human Excretion and Their Potential Sources
20. Emerging pollutant in surface water bodies: a review on monitoring, analysis, mitigation measures and removal technologies of micro-plastics
21. Dinámica espaciotemporal de la contaminación por microplásticos en sedimentos marinos de la bahía de Tumaco, pacífico colombiano
22. Understanding microplastic pollution of marine ecosystem: a review
23. Microplastics in freshwater systems: Dynamic behaviour and transport processes
24. Assessment of microplastic content in natural waters and sediments: sampling and sample preparation
25. Microplastic in Dredged Sediments: From Databases to Strategic Responses
26. Microplastic Pollution in High Population Density Zones of Selected Rivers from Southeast Asia
27. Assessment of Microplastics and Potentially Toxic Elements in Surface Sediments of the River Kelvin, Central Scotland, United Kingdom
28. Assessment of the Microplastics Content in Natural Waters and Sediments: Sampling and Sample Preparation
29. Changes and transport of microplastics in the riverbed of the mainstream below the Three Gorges Dam of the Yangtze River
30. Microplastics in the Volta Lake: Occurrence, distribution, and human health implications
31. Microplastics in packaged water, community stored water, groundwater, and surface water in rivers of Tamil Nadu after the COVID-19 pandemic outbreak
32. Approaches for Sampling and Sample Preparation for Microplastic Analysis in Laundry Effluents
33. Microplastics in complex soil matrix: Recovery, identification and removal using micro nano techniques
34. A meta-analysis highlighting the increasing relevance of the hair matrix in exposure assessment to organic pollutants
35. Why Nigeria should ban single-use plastics: Excessive microplastic pollution of the water, sediments and fish species in Osun River, Nigeria
36. Spatial distribution and vertical characteristics of microplastics in the urban river: The case of Qinhuai River in Nanjing, China
37. Katı Atık Depolama Sahası Sızıntı Sularında Mikroplastik Kirliliği
38. Recent Investigation of Characterizing, quantifying, and Contamination of Microplastic in the surface water of Adyar River Estuary, Tamil Nadu, India
39. Microplastics as Water Pollutants and Sustainable Management Strategies
40. The flow of microplastics from wastewater to the urban aquatic environment: Occurrence, fate, and an outlook on management strategies in Asia
41. Effects of microplastics on amphibian performance and survival: Current knowledge and research gaps
42. The good, the bad and the ugly: Critical insights on the applications of microbes in microplastic degradation
43. Microplastics contamination in bivalves off the island in the strait of malacca and its potential health risks
44. Identification and characteristic of microplastics in sediment and macrozoobenthos of upper Citarum River
45. The choice of a method for selecting and determining the quantitative and qualitative content of microplastics in wastewater
46. Microplastics in river sediments in two streams in an Andean region of Peru
47. Impact of flooding on microplastic abundance and distribution in freshwater environment: a review
48. What is hiding below the surface – MPs including TWP in an urban lake
49. The fate of microplastics from municipal wastewater in a surface flow treatment wetland
50. Microplastics and heavy metals in a tropical river: Understanding spatial and seasonal trends and developing response strategies using DPSIR framework
51. Abundance, spatial distribution, and physical characteristics of microplastics in stormwater detention ponds
52. Microplastics in water from the confluence of tropical rivers: Overall review and a case study in Paraiba do Sul River basin
53. Microplastic distribution in the surface water and sediment of the Ergene River
54. Microplastics in the environment: An urgent need for coordinated waste management policies and strategies
55. Microplastics everywhere: A review on existing methods of extraction
56. Riverine microplastics and their interaction with freshwater fish
57. The Presence of Microplastics and Plasticizers in Different Tissues of Mullet (Mugil cephalus) Along the East Java Coast in Indonesia
58. Levels and composition of microplastics and microfibers in the South Saskatchewan River and stormwater retention ponds in the City of Saskatoon, Canada
59. Distribution of microplastics in upstream and downstream surface waters of the Iranian rivers discharging to the southern Caspian Sea
60. Preservation, storage, and sample preparation methods for freshwater microplastics – a comprehensive review
61. Risk assessments of microplastics accumulated in estuarine sediments at Cuddalore, Tamil Nadu, southeast coast of India
62. Microplastic Pollution in Freshwater Sediments: Abundance and Distribution in Selangor River Basin, Malaysia
63. Microplastic pollution in riverine ecosystems: threats posed on macroinvertebrates
64. Levels and composition of microplastics and microfibers in the South Saskatchewan River and stormwater retention ponds in the City of Saskatoon, Canada
65. Spatial distribution of microplastics in a large watershed: a case study of the Ottawa River watershed
66. Microplastics in freshwater environment: occurrence, analysis, impact, control measures and challenges
67. Occurrence and spatial distribution of microplastic contaminated with heavy metals in a tropical river: Effect of land use and population density
68. Microplastic as an Emerging Environmental Threat: A Critical Review on Sampling and Identification Techniques Focusing on Aquactic Ecoystem
69. Exposure of the amphipod Hyalella azteca to microplastics. A study on subtoxic responses and particle biofragmentation
70. Microplastic pollution in the Himalayas: Occurrence, distribution, accumulation and environmental impacts
71. Biological responses of Chironomus sancticaroli to exposure to naturally aged PP microplastics under realistic concentrations
72. Microplastics in carnivorous fish species, water and sediments of a coastal urban lagoon in Nigeria
73. Trapped microplastics within vertical redeposited sediment: Experimental study simulating lake and channeled river systems during resuspension events
74. Characterization of microplastic pollution in the Pasur river of the Sundarbans ecosystem (Bangladesh) with emphasis on water, sediments, and fish
75. Characterization of suspended microplastics in surface waters of Chalakudy River, Kerala, India
76. Effects of microfiber exposure on medaka (Oryzias latipes): Oxidative stress, cell damage, and mortality
77. Recent developments in microplastic contaminated water treatment: Progress and prospects of carbon-based two-dimensional materials for membranes separation
78. Temporal and spatial distribution of microplastic in the sediment of the Han River, South Korea
79. Microplastics and leaf litter decomposition dynamics: New insights from a lotic ecosystem (Northeastern Italy)
80. Microfluidics as a Ray of Hope for Microplastic Pollution
81. Unaccounted Microplastics in the Outlet of Wastewater Treatment Plants—Challenges and Opportunities
82. Impact of coastal wastewater treatment plants on microplastic pollution in surface seawater and ecological risk assessment
83. Baseline concentration of microplastics in surface water and sediment of the northern branches of the Mekong River Delta, Vietnam
84. Environmental and land use controls of microplastic pollution along the gravel-bed Ain River (France) and its “Plastic Valley”
85. Estimated discharge of microplastics via urban stormwater during individual rain events
86. Evaluating community science sampling for microplastics in shore sediments of large river watersheds
87. Occurrence and Characteristics of Microplastics in the Surface Water and Sediment of Lagos Lagoon, Nigeria
88. Characterization and Toxicology of Microplastics in Soils, Water and Air
89. Removal of Environmental Microplastics by Advanced Oxidation Processes
90. Status of Microplastic Pollution in the Freshwater Ecosystems
91. Microplastic Pollution in the Yellow River Basin: Current Status and Control Strategy
92. Microplastics in water, sediments, and fish at Alpine River, originating from the Hindu Kush Mountain, Pakistan: implications for conservation
93. Occurrence of MPs and NPs in freshwater environment
94. Sources and occurrence of microplastics and nanoplastics in the environment
95. Spatial Distribution and Vertical Characteristics of Microplastics in the Urban River: The Case of Qinhuai River in Nanjing, China
96. Microplastics Contamination in Receiving Water Systems
97. Interaction of micro(nano)plastics with extracellular and intracellular biomolecules in the freshwater environment
98. Comparison between the traditional Manta net and an innovative device for microplastic sampling in surface marine waters
99. Potential Adsorption Affinity of Estrogens on LDPE and PET Microplastics Exposed to Wastewater Treatment Plant Effluents
100. Comparison of different salt solutions for density separation of conventional and biodegradable microplastic from solid sample matrices
101. Pervasiveness and characteristics of microplastics in surface water and sediment of the Buriganga River, Bangladesh
102. Pilot study on microplastics in the Suquía River basin: Impact of city run-off and wastewater treatment plant discharges in the mid-2010s
103. An innovative approach for microplastic sampling in all surface water bodies using an aquatic drone
104. Fate, transport and degradation pathway of microplastics in aquatic environment — A critical review
105. A case study on small-size microplastics in water and snails in an urban river
106. Spatial Variations in Microfiber Transport in a Transnational River Basin
107. Microplastics in freshwater environment: the first evaluation in sediment of the Vaal River, South Africa
108. Wastewater plastisphere enhances antibiotic resistant elements, bacterial pathogens, and toxicological impacts in the environment
109. Change in microplastic concentration during various temporal events downstream of a combined sewage overflow and in an urban stormwater creek
110. Characteristics of microplastic pollution and analysis of colonized-microbiota in a freshwater aquaculture system.
111. First biomonitoring of microplastic pollution in the Vaal river using Carp fish (Cyprinus carpio) “as a bio-indicator”
112. Microplastics in soil and freshwater: Understanding sources, distribution, potential impacts, and regulations for management
113. Microplastic occurrence in the northern South China Sea, A case for Pre and Post cyclone analysis
114. First observation of microplastics in surface sediment of some aquaculture ponds in Hanoi city, Vietnam
115. Biofilm formation and its implications on the properties and fate of microplastics in aquatic environments: A review
116. Vertical distribution and river-sea transport of microplastics with tidal fluctuation in a subtropical estuary, China
117. Interactions of Microplastics Toward an Ecological Risk in Soil Diversity
118. A PLETHORA OF MICROPLASTIC POLLUTION STUDIES: THE NEED FOR A FORENSIC APPROACH
119. Microplastics pollution in selected rivers from Southeast Asia
120. Source-sink process of microplastics in watershed-estuary-offshore system
121. Quantification and Characterisation of Pre-Production Pellet Pollution in the Avon-Heathcote Estuary/Ihutai, Aotearoa-New Zealand
122. A Peristaltic Pump and Filter-Based Method for Aqueous Microplastic Sampling and Analysis
123. Sample preparation methods for the analysis of microplastics in freshwater ecosystems: a review
124. Microplastic concentrations, characteristics, and fluxes in water bodies of the Tollense catchment, Germany, with regard to different sampling systems
125. Controlling Factors of Microplastic Riverine Flux and Implications for Reliable Monitoring Strategy
126. Microplastics in Freshwater Ecosystems
127. Microplastics in Terrestrial and Freshwater Environments
128. Microplastics Occurrence in Different Regions Around the World
129. Microplastics Pollution in Rivers
130. The Role of Rivers in Microplastics Spread and Pollution
131. What have we known so far for fluorescence staining and quantification of microplastics: A tutorial review
132. Microplastics in the Freshwater Environment
133. Polyethylene terephthalate and di-(2-ethylhexyl) phthalate in surface and core sediments of Bohai Bay, China: Occurrence and ecological risk
134. Seasonal distribution of microplastics in the surface water and sediments of the Vellar estuary, Parangipettai, southeast coast of India
135. Microplastic sampling techniques in freshwaters and sediments: a review
136. Microplastics in the Koshi River, a remote alpine river crossing the Himalayas from China to Nepal
137. How fast, how far: Diversification and adoption of novel methods in aquatic microplastic monitoring
138. Distribution and characteristics of microplastics and phthalate esters from a freshwater lake system in Lesser Himalayas
139. A proposed nomenclature for microplastic contaminants
140. Behavior of Microplastics in Inland Waters: Aggregation, Settlement, and Transport
141. Microplastic pollution in freshwater systems in Southeast Asia: contamination levels, sources, and ecological impacts
142. Identification and removal of micro- and nano-plastics: Efficient and cost-effective methods
143. Spatio-temporal distribution of microplastics in a Mediterranean river catchment: The importance of wastewater as an environmental pathway
144. Review of Microplastic Distribution, Toxicity, Analysis Methods, and Removal Technologies
145. Evaluating Marine Debris Trends and the Potential of Incineration in the Context of the COVID-19 Pandemic in Southern Bali, Indonesia
146. What You Net Depends on if You Grab: A Meta-analysis of Sampling Method’s Impact on Measured Aquatic Microplastic Concentration
147. Big eyes can't see microplastics: Feeding selectivity and eco-morphological adaptations in oral cavity affect microplastic uptake in mud-dwelling amphibious mudskipper fish
148. Acute riverine microplastic contamination due to avoidable releases of untreated wastewater
149. Microplastic pollution in Southern Atlantic marine waters: Review of current trends, sources, and perspectives
150. Microplastics in aquatic environments: A review on occurrence, distribution, toxic effects, and implications for human health
151. Abundance, interaction, ingestion, ecological concerns, and mitigation policies of microplastic pollution in riverine ecosystem: A review
152. Anthropogenic particles (including microfibers and microplastics) in marine sediments of the Canadian Arctic
153. The missing ocean plastic sink: Gone with the rivers
154. Salt marsh sediments act as sinks for microplastics and reveal effects of current and historical land use changes
155. Treatment processes for microplastics and nanoplastics in waters: State-of-the-art review
156. A comprehensive review on assessment of plastic debris in aquatic environment and its prevalence in fishes and other aquatic animals in India
157. A New Collection Tool-Kit to Sample Microplastics From the Marine Environment (Sediment, Seawater, and Biota) Using Citizen Science
158. Microplastics in lakeshore and lakebed sediments – External influences and temporal and spatial variabilities of concentrations
159. Characterization of plastics and their ecotoxicological effects in the Lambro River (N. Italy)
160. Assessing plastic size distribution and quantity on a remote island in the South Pacific
161. Considerations on salts used for density separation in the extraction of microplastics from sediments
162. Presence and fate of microplastics in the water sources: focus on the role of wastewater and drinking water treatment plants
163. Comparative study of three sampling methods for microplastics analysis in seawater
164. Abundance and distribution of microplastics in Baturusa watershed of Bangka Belitung Islands Province
165. Spatial distribution of microplastic in sediment of the Citanduy River, West Java, Indonesia
166. Impact of dyes and finishes on the microfibers released on the laundering of cotton knitted fabrics
167. Sampling and processing methods of microplastics in river sediments - A review
168. Effects of hydrodynamics on the cross‐sectional distribution and transport of plastic in an urban coastal river
169. Microplastic and other anthropogenic microparticles in water and sediments of Lake Simcoe
170. A systematic review of the literature on plastic pollution in the Laurentian Great Lakes and its effects on freshwater biota
171. Characterization of microplastics in mangrove sediment of Muara Angke Wildlife Reserve, Indonesia
172. Microplastics in freshwater sediment: A review on methods, occurrence, and sources
173. Horizontal and vertical distribution of microplastics in the Wuliangsuhai Lake sediment, northern China
174. Transfer and effects of PET microfibers in Chironomus riparius
175. Stable Isotope Insights into Microplastic Contamination within Freshwater Food Webs
176. Spatial Distribution of Microplastics in Surficial Benthic Sediment of Lake Michigan and Lake Erie
177. Characterization of microplastics and anthropogenic fibers in surface waters of the North Saskatchewan River, Alberta, Canada
178. Occurrence, Fate, and Removal of Microplastics in Sewage Treatment Plants (STPs)
179. Microplastics in freshwater ecosystems: a recent review of occurrence, analysis, potential impacts, and research needs
180. Filling in the knowledge gap: Observing MacroPlastic litter in South Africa's rivers
181. Microplastics as an emerging hazard to terrestrial and marine ecosystems: Sources, Occurrence and Analytical Methods
182. Kelimpahan dan komposisi sampah plastik di DAS Baturusa Provinsi Kepulauan Bangka Belitung
183. A Review on Analytical Methods and Occurrences for Microplastics in Freshwater
184. Microplastics in a dam lake in Turkey: type, mesh size effect, and bacterial biofilm communities
185. Occurrence, removal and potential threats associated with microplastics in drinking water sources
186. Rapid fragmentation of microplastics by the freshwater amphipod Gammarus duebeni (Lillj.)
187. Microplastic Presence in Sediment and Water of a Lagoon Bordering the Urban Agglomeration of Lagos, Southwest Nigeria
188. Microplastics in the environment: Interactions with microbes and chemical contaminants
189. Sample Preparation Techniques for the Analysis of Microplastics in Soil—A Review
190. Microplastic Concentrations in Raw and Drinking Water in the Sinos River, Southern Brazil
191. Warum eine Risikoabschätzung und Grenzwertsetzung für Mikrokunststoffe in der aquatischen Umwelt problematisch ist
192. Microplastic quantification affected by structure and pore size of filters
193. Microplastics in water, sediment and fish from the Fengshan River system: Relationship to aquatic factors and accumulation of polycyclic aromatic hydrocarbons by fish
194. Microplastic abundance and accumulation behavior in Lake Onego sediments: a journey from the river mouth to pelagic waters of the large boreal lake
195. Approaching the environmental problem of microplastics: Importance of WWTP treatments
196. The first report on the source-to-sink characterization of microplastic pollution from a riverine environment in tropical India
197. Quantification of microplastic in Red Hills Lake of Chennai city, Tamil Nadu, India
198. How to detect small microplastics (20–100 μm) in freshwater, municipal wastewaters and landfill leachates? A trial from sampling to identification
199. Sampling and Quality Assurance and Quality Control: A Guide for Scientists Investigating the Occurrence of Microplastics Across Matrices
200. Soil Pollution from Micro- and Nanoplastic Debris: A Hidden and Unknown Biohazard
201. Effects of MP Polyethylene Microparticles on Microbiome and Inflammatory Response of Larval Zebrafish
202. Infiltration Behavior of Microplastic Particles with Different Densities, Sizes, and Shapes—From Glass Spheres to Natural Sediments
203. Microplastics in sediments from an interconnected river-estuary region
204. The Role of Legislation, Regulatory Initiatives and Guidelines on the Control of Plastic Pollution
205. Global distribution of microplastics and its impact on marine environment—a review
206. Microplastics as contaminants in freshwater environments: A multidisciplinary review
207. Microplastics in offshore fish from the Agulhas Bank, South Africa
208. Bioremediation as a promising strategy for microplastics removal in wastewater treatment plants
209. Microplastics in fishes and their living environments surrounding a plastic production area
210. An unintended challenge of microplastic pollution in the urban surface water system of Lahore, Pakistan
211. Distribution, abundance, and diversity of microplastics in the upper St. Lawrence River
212. The way of microplastic through the environment – Application of the source-pathway-receptor model (review)
213. What Is the Minimum Volume of Sample to Find Small Microplastics: Laboratory Experiments and Sampling of Aveiro Lagoon and Vouga River, Portugal
214. Distribution of microplastics in surface water of the lower Yellow River near estuary
215. The first occurrence, spatial distribution and characteristics of microplastic particles in sediments from Banten Bay, Indonesia
216. Factors Controlling the Distribution of Microplastic Particles in Benthic Sediment of the Thames River, Canada
217. Potential health impact of environmental micro‐ and nanoplastics pollution
218. Occurrence, Fate and Fluxes of Plastics and Microplastics in Terrestrial and Freshwater Ecosystems
219. Occurrence, Sources, Transport, and Fate of Microplastics in the Great Lakes–St. Lawrence River Basin
220. Microplastics in Freshwater Ecosystems
221. “Down by the River”: (Micro-) Plastic Pollution of Running Freshwaters with Special Emphasis on the Austrian Danube
222. Plastic and Microplastic Pollution: From Ocean Smog to Planetary Boundary Threats
223. Initial Survey of Microplastics in Bottom Sediments from United States Waterways
224. Microplastics in Freshwater Environments
225. Superimposed microplastic pollution in a coastal metropolis
226. A Review of Microplastics in Freshwater Environments: Locations, Methods, and Pollution Loads
227. The impact of improper solid waste management to plastic pollution in Indonesian coast and marine environment
228. Microplastics in the environment: A critical review of current understanding and identification of future research needs
229. Impacts of plastic products used in daily life on the environment and human health: What is known?
230. Microplastics in oysters (Crassostrea gigas) and water at the Bahía Blanca Estuary (Southwestern Atlantic): An emerging issue of global concern
231. Evaluation of continuous flow centrifugation as an alternative technique to sample microplastic from water bodies
232. Spatiotemporal distribution and annual load of microplastics in the Nakdong River, South Korea
233. Recommended best practices for plastic and litter ingestion studies in marine birds: Collection, processing, and reporting
234. Emerging threats and persistent conservation challenges for freshwater biodiversity
235. The effect of dams on river transport of microplastic pollution
236. Microplastics in freshwaters and drinking water: Critical review and assessment of data quality
237. Citizen science sampling programs as a technique for monitoring microplastic pollution: results, lessons learned and recommendations for working with volunteers for monitoring plastic pollution in freshwater ecosystems
238. Microplastics in freshwater sediments of Atoyac River basin, Puebla City, Mexico
239. Methods for sampling and detection of microplastics in water and sediment: A critical review
240. OCCURENCE OF MICROPLASTICS AND ESTIMATION OF SOURCES TO RIVER WATER IN KATHMANDU CITY, NEPAL
241. Synthetische Kunststoffe (Plastik)
242. Garbage in guano? Microplastic debris found in faecal precursors of seabirds known to ingest plastics
243. A watershed-scale, citizen science approach to quantifying microplastic concentration in a mixed land-use river
244. Toward an ecotoxicological risk assessment of microplastics: Comparison of available hazard and exposure data in freshwaters
245. Abundance, Distribution, and Drivers of Microplastic Contamination in Urban River Environments
246. Microplastic hotspots in the Snake and Lower Columbia rivers: A journey from the Greater Yellowstone Ecosystem to the Pacific Ocean
247. Microplastics in sediment from Skudai and Tebrau river, Malaysia: a preliminary study
248. Occurrence of microplastics in municipal sewage treatment plants: a review
249. Mountains to the sea: River study of plastic and non-plastic microfiber pollution in the northeast USA