Criteria for a good catch: A conceptual framework to guide sourcing of sustainable salmon fisheries
Abstract
The identification of sustainably managed fisheries is problematic for marketers and consumers of Pacific salmon food products owing to lack of well-defined and robust criteria that take into account current ecosystem science of salmon. We present the rationale for an alternative conceptual framework for salmon management that supports the development of sustainable sourcing criteria. Our approach contrasts with current large-scale fisheries certification programs such as that of the Marine Stewardship Council (MSC) and general consumer recommendation services such as Monterey Bay Aquarium’s Seafood Watch (SFW) program. Our framework is based on the “place-based” character of salmon populations and recognition of fundamental aspects of salmon ecology, particularly the evolution of population life histories that are locally adapted to freshwater spawning and rearing habitats. We describe how this framework underpins development of science-based sourcing criteria and how it differs in important respects from the industrial approach that historically and currently is the basis for most salmon management. We conclude with a discussion of how the framework and its application may provide a model for redirecting salmon management, in general, towards a more science- and place-based approach and why that is likely to be sustainable in the long term in a way that most contemporary salmon management is not.
Introduction
The past two decades have seen increased desire by consumers in western countries to eat more natural, healthy foods and to support practices that provide such foods in a manner judged to be “sustainable”, i.e., products derived from ecosystem services deemed demonstrably viable over the long term (decades to centuries). This has led to the development of certification bodies that review and certify (or not) retail products and brands as produced from sustainable sources (for example, organically grown produce). Marine finfish and shellfish products, in particular, necessitate public resource management to address sustainable harvest. In the case of Pacific salmon, hatchery practices and the persistence of salmon for wildlife and ecosystem benefits are also considerations for fisheries sustainability.
The most prominent fisheries certification body is the Marine Stewardship Council (MSC) that uses independent third-party certification bodies to evaluate candidate fisheries. Less formally, the Seafood Watch (SFW) program of the Monterey Bay Aquarium reviews fishery practices and provides consumers with reports and general categories (red, yellow, green) of seafood products intended to help them identify products from sustainably managed fisheries. Concerns have been raised over the relatively large scale and rising cost of MSC certifications. In addition, controversies have arisen regarding the independence and accuracy of sustainability assessments (Jacquet and Pauly 2007; Christian et al. 2013; Kirby et al. 2014). Indeed, the MSC process has blanket-certified all five species of Pacific salmon caught in Alaska marine waters as sustainably harvested, even though hundreds of place-based stocks are involved, each with local environmental controls on production as well as highly variable stakeholder interests (e.g., many Alaskan-caught and -labelled Chinook, sockeye, and chum can have natal origins in watersheds of western Canada and the lower west coast of the US). The real and long-term impact of mixed-stock ocean fisheries on local (place-based) populations therefore is lost, or at least compromised, in this kind of wide-ranging certification process, and some of these populations are not being sustained (e.g., populations within BC pink and chum Certification Units and within BC’s Fraser sockeye Certification Unit, see Price et al. 2017).
A complementary, if not alternative, approach to large-scale certification is for retailers to develop their own sustainable sourcing criteria to guide their acquisition of raw or processed food products that they package or further process for direct sale to consumers. Development and application of self-determined criteria that are rooted in salmon ecology have the potential to result in a more rigorous evaluation of sustainability. This is because the retailer assumes responsibility for its claim that the fishery is sustainably managed.
The outdoor retail clothing company Patagonia has recently developed a consumer food products line known as Provisions. An important initial food item for the program is packaged smoked sockeye (Oncorhynchus nerka (Walbaum in Artedi, 1792)) and pink salmon (Oncorhynchus gorbuscha (Walbaum, 1792)). The program seeks to accomplish two related objectives with its salmon product sourcing. First, it aims to acquire premium salmon products from fisheries that harvest wild, naturally reproducing salmon in a sustainable manner. Second, it seeks to support salmon fisheries that provide models of local, place-based fisheries that contrast with the majority of large-scale, mixed-stock salmon fisheries on the west coast of the US and Canada. Patagonia wants its salmon sourcing criteria to reflect maintenance of local social values in addition to sound science regarding salmon ecology and fisheries management. To assist Patagonia, we developed over-arching criteria for sourcing wild salmon products with a view that these self-imposed criteria could serve as a well-defined and robust complement to existing certification programs. This approach could also increase return on investment for other retailers that choose to provide high-quality, sustainable salmon products derived from robust criteria.
Salmon sourcing and framework criteria are based on the recognition that (1) wild salmon are locally adapted to the rivers and streams in which adults spawn and juveniles rear and (2) that abundant spawning and rearing salmon support numerous ecological functions (e.g., wildlife benefits, carcass fertilization to streams, etc.) that must be maintained to maintain the health of the salmon populations themselves. This makes salmon populations “place-based”, a response to and reflection of the local habitat and environmental conditions with which they have evolved and to which they are (locally) adapted. We argue that the place-based nature of salmon populations requires that the management of salmon harvest be appropriately place-based as well. Thus, we based our sourcing criteria on this fundamental attribute of wild salmon populations and its significance to Pacific salmon ecology and management. The place-based criteria are listed in Box 1 and discussed in the Criteria for Sustainable Sourcing section.
Box 1. Criteria for sustainable salmon sourcing.
Criteria that apply to the source population
•
Target source population(s) is (are) known or estimated with high probability.
•
A defensible escapement goal is in place and supported by high-quality data; the escapement goal meets, at minimum, a maximum sustainable yield (MSY) target and preferably the goal is greater than the MSY point estimate.
•
If the escapement goal is an MSY-based target, there must be evidence that the goal is met or exceeded in 80% of the past 10 years.
•
If an escapement goal is not in place and (or) sufficient high-quality escapement data are not available, additional fishery information must be provided for review by an independent science panel (ISP) familiar with the sourcing criteria or their derivation to determine if the fishery exemplifies a place-based and (or) risk-averse fishery (as described below).
Criteria concerning landed and non-landed by-catch of non-target salmon and non-salmon populations
•
Are non-target salmon or non-salmon species encountered and captured (landed) in the fishery?
◦
If “yes”, the population(s) of origin of the non-target fish must either be known or estimated with sufficient confidence (for example, from genetic stock identification) to determine that no conservation concerns exist for non-target populations. Ideally, determination of “no conservation concern” would require that equally risk-averse escapement goals exist for the non-target population(s) and are achieved with high confidence (accounting for all sources of fishery-induced mortality). In the interim, the minimum requirement is that “no conservation concern” exists, as determined by the management agency or supported by data reviewed by the ISP.
◦
Are non-target salmon or non-salmon species encountered by the fishery but not retained, either being released (harmed or unharmed) or encountered as drop-off from fishing gear?
◦
Preference is for fisheries that employ selective fishing gears and practices that can release non-target species with no or little harm and have supporting data that attest to high post-encounter/release survival from similar gear types or from the fishery itself.
◦
Preference is given to fisheries where by-catch populations are of known origin or credibly estimated by the management agency, and the status of those by-catch populations is known to meet risk-averse escapement goals.
◦
If by-catch is known or estimated to involve drop-off from encounter with the gear, there must be a credible estimate of the encounter rate and the drop-off mortality rate.
Criteria concerning the evaluation of the fishing methodology
•
Regardless of the fishery location, the river(s) of origin of the source population(s) must be known or credibly estimated (for example, by genetic stock identification). Knowing the source population(s) is important if the fishery targets several populations that are not distinguishable by external characteristics from any other individuals retained by the fishery.
•
Preference is given to fishing gears that are population selective and capable of identifying and releasing non-target populations/species with minimal harm.
•
Preference is given to passive fishing gears such as reef nets, fish traps, and fish wheels that do not require lengthy handling of non-target individuals, if any, and do not require removing the fish from the water for any significant time, if at all.
•
Preference is given to fishing gears and associated practices that result in little or no crushing or bruising of landed fish and that the quality of flesh is preserved by promptly bleeding and icing the catch. Passive selective gears are most capable of meeting this criterion.
•
Purse seine and drift or set gillnet fisheries must employ short soak times and landing practices that avoid or minimize bruising or crushing of retained fish and that employ careful sorting and release of by-catch. Evidence must be provided regarding the post-release survival of by-catch.
Criteria concerning model fisheries and gears
Preference is given to salmon sourced from fisheries that produce a high value-added product that can be marketed to consumers wishing to support ecologically sustainable and socially responsible products that return a living wage to fishers in local fishing communities. Such fisheries should have the following characteristics:
•
small, local fisheries that meet the biological criteria that exemplify place-based fishing,
•
fisheries that are, or are capable of, sustaining local place-based fishing communities,
•
fisheries using passive fishing gears capable of releasing non-target individuals with little or no handling,
•
fisheries whose gear and practices have a low carbon footprint relative to boat-based fisheries that employ drift gillnets or purse seines and for which fuel may make up a significant component of daily operating costs, and
•
fisheries that land target fish with little or no handling, bruising, or crushing and that promptly bleed and ice the landed catch.
Criteria regarding chain of custody
All salmon products sold under the retailer’s label must provide the following information:
•
the fishery from which the salmon was obtained,
•
the type of gear employed, and
•
the identity of the population(s) to the finest spatial scale possible, sufficient so that the consumer can determine the status of the population(s) to which the salmon belong.
Materials and methods
Ecosystem ecology of wild salmon
The recent diversity and distribution of wild Pacific salmon derives from complex, dynamic freshwater and marine environments that have shaped populations through multiple generations, following the end of the Pleistocene glaciation, approximately 10 000 years ago (Waples et al. 2008). New freshwater spawning and rearing environments, estuaries, and ocean habitats offered numerous ecological niches that salmon recolonized, adapted to, and exploited. As described by Stanford et al. (2005), the dynamism of river/floodplain/estuary systems results in a temporally and spatially shifting mosaic of rearing and spawning habitats that drives the local adaptation and life-history diversity of wild salmon. This process has maintained (1) a balance between egg fecundity (several thousand per female) and large egg size that ensures survival to emergence in freshwater, subsequent juvenile survival to ocean entry, and then adequate returns as adults to replace the parental generation (Fleming and Gross 1990; Einum et al. 2002; Heath et al. 2003) and (2) high homing fidelity of returning adult spawners to natal habitats in freshwater (Hendry et al. 2004; Quinn 2005). Local or place-based adaptation has been defined as a “process that increases the frequency of traits within a population that enhance survival or reproductive success of individuals expressing such traits” (Taylor 1991, p. 185). Thus, these adaptations involve suites of biological and behavioral characteristics that are under varying degrees of genetic and epigenetic control (Hutchings 2011; Salvolainen et al. 2013). Biological characteristics that drive and reflect local adaptation include:
•
age, size, and timing of smoltification and ocean entry (Taylor 1991; Groot and Margolis 1991; Quinn 2005);
•
influence of temperature at specific life stages (e.g., rate of incubation, timing of emergence, rate and timing of juvenile growth, upstream spawning migration (Groot and Margolis 1991; Quinn 2005; Hutchings 2011));
•
direction of migration to lake (sockeye inlet- or outlet-spawning populations) (Taylor 1991; Groot and Margolis 1991; Quinn 2005);
•
age/size(s) at maturation (Taylor 1991; Groot and Margolis 1991; Quinn et al. 2001a, 2001b; Quinn 2005); and
•
sex ratio of adult spawning populations (Groot and Margolis 1991; Quinn 2005).
This suite of life-history characteristics has evolved to provide a solution to the challenges that salmon face in finding favorable and productive habitat during each life cycle stage in sequence, temporally and spatially, from spawning grounds to freshwater rearing to ocean migration and adult return to natal watersheds. This process results in salmon populations possessing a portfolio of successful life histories organized at a hierarchy of spatial scales that serve to buffer local and metapopulation complexes from environmental variations that can affect survival at different life-history stages (Schindler et al. 2003, 2010; Moore et al. 2010, 2014; Satterthwaite and Carlson 2015). Moreover, salmon populations fulfill key ecological roles (niches) within the salmon ecosystem throughout their life cycles, as detailed below.
•
Salmon deliver significant quantities of key nutrients that subsidize and link marine, freshwater, and terrestrial food webs (Gende et al. 2002; Kohler et al. 2013; Harding and Reynolds 2014).
•
Salmon are an important, often primary, prey item for aquatic, avian, and terrestrial predators and scavengers before, during, and after spawning, and as juveniles rearing in freshwater (e.g., Wood 1987; Cederholm et al. 1999; Darimont et al. 2010; Elliott et al. 2011; Levi et al. 2012).
•
In marine environments, salmon provide equally important prey for marine mammals, salmon sharks, other fish, and avian predators at key times and stages in their life cycles (Fryer 1998; Ford et al. 2010; Williams et al. 2011; Chasco et al. 2017).
Salmon also experience high levels of intraspecific competition for finite amounts of rearing space and food. As such, they have evolved a suite of juvenile growth strategies that enable populations to thrive in spite of intense competition for food and cover, predators, and widely varying environmental conditions such as temperature and salinity (Groot and Margolis 1991; Quinn 2005; Moore et al. 2014).
Salmon reproduction is characterized by moderate levels of fecundity (egg numbers of 2–12 000) and large egg sizes relative to most marine fishes (4–8 mm diameter, Groot and Margolis 1991; Quinn 2005). Egg size is adapted to provide for relatively high embryo survival and subsequent fry dispersal to rearing habitats. A population’s mean egg size and number is the likely result of adaptation to the range of average environmental conditions that affect juvenile growth and survival, marine survival, and ultimately productivity (Fleming and Gross 1990; Einum et al. 2002; Braun et al. 2013).
Complementing these characteristics of salmon fecundity is the presence of multiple ages of maturity, with the notable exception of pink salmon that mature at a single age (two). Multiple ages of maturity result in overlapping generations (Waples et al. 2013) that further buffer environmental variation and contribute to the portfolio of different life histories (Moore et al. 2014; Copeland et al. 2017). This helps to explain the persistence of wild salmon presence across much of the northern hemisphere and their rapid dispersion after introduction to non-native habitats of Chile, Argentina, and New Zealand (Quinn et al. 2001a, 2001b; Quinn 2005).
Conceptual foundation for criteria development
Lichatowich and Williams (2009, p. 1011) characterized a conceptual foundation for fisheries management as “the set of principles, assumptions, and beliefs about how ecosystem processes influence or control fish productivity. A robust conceptual foundation therefore determines what problems (e.g., limitations on production) are identified, what information is collected, and how it is interpreted, and as a result, establishes the range of appropriate solutions”. It also determines broadly where the “burden of proof” lies when developing fishery management plans (Dayton 1998; Gerrodette et al. 2002; Charles 2002). Burden of proof in this context would identify where harm from a contemplated action is presumed to result and which party—the party proposing the action or the party opposing it—must demonstrate that the potential harm is, or is not, likely to occur. Under the place-based conceptual foundation, the burden lies on those proposing a specific fishery management action to demonstrate (with sufficiently high probability) that the action assures spawner escapement goals will be met and associated ecosystem services provided by the exploited population(s) will not be impaired.
Thus, we grounded our sourcing criteria within a scientific and philosophical framework that emphasizes place-based life-history diversity within and between the five species of wild Pacific salmon native to the north eastern Pacific Ocean (sensu Lichatowich 2013; see also, Lichatowich et al. 2017) and in a burden of proof context. As described in the Ecosystem section, diversity of Pacific salmon manifests at the population level from unique adaptations to specific conditions within freshwater habitats and the high degree of homing fidelity to these natal sites. This fundamental character of wild salmon—and the conservation and management considerations that emerge from it—are referred to as place-based. Most contemporary management schemes mix or amalgamate populations, even within river systems and thus to varying degrees depart from this basic tenet and obfuscate assessment of sustainability. We use the term “population” instead of the more common term “stock” to emphasize our focus on the place-based character of wild salmon (see also, Waples and Gaggiotti 2006).
Implications of the place-based approach for harvest management
The aspects of salmon ecology that we argue are associated with high intraspecific competitive abilities in juvenile rearing habitats (including a modest number of relatively large eggs, multiple ages at maturity, and the resulting overlapping of generations) have significant implications for salmon harvest management. Salmon populations are composed of portfolios of life histories that are organized at hierarchical spatial scales, primarily within and between river basins. Each population within a portfolio is place-based, a product of the selective forces operating within the range of habitats to which the population and its component life histories have adapted.
Because the individual population is the fundamental unit, population portfolios within river basins are likely to be particularly important for the resilience of the larger river basin portfolio (Moore et al. 2010, 2014; Carlson and Satterwaite 2011). These populations at the “within-river basin” scale are most vulnerable to exploitation because they are numerically smaller than populations at the aggregate whole-basin or regional scale (e.g., the entire Fraser or Skeena River systems versus the Chilcotin or Babine Rivers that are a tributary to the main Fraser and Skeena Rivers, respectively). Salmon harvest (and salmon population management more generally) must, therefore, emphasize the preservation of the adaptive integrity of individual populations within river basins. And, importantly, this requires managing salmon harvest to assure that spawning escapements at a level equal to or greater than maximum sustainable yield (MSY) are consistently attained in the majority of return years for all target populations.
The current framework for US and Canadian harvest management of Pacific salmon is firmly based in the concept of MSY. MSY is defined as the level of average annual spawning escapement (or total egg deposition) that will produce the greatest total recruitment in excess of the average parent spawning escapement under prevailing average environmental conditions affecting recruitment. MSY is estimated from stock-specific data used to estimate adult-to-adult recruitment parameters of a presumed appropriate stock-recruit model. Although there are concerns whether MSY spawning levels are sufficient to preserve locally adapted populations or, more concerning, whether MSY is at all compatible with an ecosystem approach to fisheries management (Larkin 1977; Sainsbury et al. 2000; Mangel et al. 2002; Pikitch et al. 2004; Cadrin and Pastoors 2008), we believe that, if the MSY approach is used, minimum escapement levels for individual populations must be estimated from high-quality time-series data and estimation procedures account for all major sources of uncertainty. Consistent attainment of minimal spawner escapements is necessary to maintain a population’s life history, genetic diversity, and intraspecific competition at levels adequate to drive adaptation to changing environments.
One of the principal sources of uncertainty for assuring that MSY (or greater) spawner escapement goals are consistently attained is the current mixed-stock nature of most marine salmon fisheries. Most wild salmon harvest is managed at broad spatial scales at which multiple populations of target species are encountered. Management of salmon populations as large aggregate stocks is implicitly in conflict with the place-based character of salmon that is fundamental to their long-term viability and resiliency. This further implies that such management is in conflict with the preservation of salmon diversity and life history complexity. To varying degrees, depending on the stock aggregates, such management is placeless and largely exonerates management from the need to maintain specific wild salmon populations.
Criteria for sustainable sourcing
In Box 1, we list the basic criteria for retail sourcing of salmon from sustainably managed fisheries. The criteria were based on fundamental principles of salmon ecology and developed as a result of our work with the Patagonia Provisions program; these criteria can be applied by any retailer sourcing wild salmon that wants to assure consumers that its salmon products meet a high standard for sustainability. The criteria attempt to capture the primary features of place-based salmon ecology as they apply to the acquisition of salmon products from fisheries that meet or closely approach the place-based ideal. We discuss the strengths and weaknesses of the criteria after listing them.
These criteria represent a foundation for the support of place-based salmon management by conscientious retailers who desire salmon products from sustainable fisheries. The criteria embody two key elements of place-based fisheries management. First, they allow selection of populations currently managed for MSY if accompanied by clear evidence of regularly attaining or exceeding those MSY-determined escapements. Second, other criteria emphasize fishing practices that demonstrably sustain target populations without adverse effects to their respective ecosystems. This requires chain of custody documentation on the product label delivered to consumers. This requirement differs from consumer advisory services such as SFW that do not address chain of custody and from assessment bodies employing third-party assessors, such as the MSC. MSC only requires traceability of a certification unit to a supplier and doesn’t provide fishery details on the label. This addresses the difficulty most establishments selling sustainable seafood currently experience—they cannot answer consumer questions about which population or fishery the salmon they sell comes from or what gear type was used to catch it. Adherence to the criteria in Box 1 ensures a high-quality, sustainable salmon product that supports value-added processing and a high return to fishers and their communities.
A related feature of our criteria is the absence of conditional approval for a product caught in a fishery that does not fully meet the criteria. Salmon from an MSC fishery that have been certified “sustainable” but with “conditions” are indistinguishable at the point of sale from salmon from a fishery certified unconditionally. Consumers have no knowledge that the fishery has failed one or more sustainability criteria or received conditional certification and repeatedly failed to meet the conditions (see Christian et al. 2013). Acceptance of a product under our approach is all or nothing: a product is accepted if and only if it meets the criteria.
Discussion
Requirements for the implementation of place-based salmon management
As a policy, Patagonia Provisions has chosen not source hatchery-produced salmon. Sustainable, place-based salmon management requires an approach that is not reliant on, or attracted to, technological subsidies to harvest from large-scale hatchery production that imposes high ecological costs on wild salmon and undetermined economic costs on society (NRC 1996; Knapp et al. 2007; Radke and Carter 2009; Lichatowich et al. 2017). Place-based management also requires the employment of selective fishing practices and gears that enable non-target salmon populations to be released with a high probability of post-release survival to spawn. Place-based management also supports a focus on the preservation and restoration of natural physical processes that form and maintain salmon spawning and rearing habitats (sensu Stanford et al. 2005). The criteria we have developed for sourcing salmon support these objectives.
We believe that place-based salmon management is most likely to succeed when most, perhaps all, salmon harvest occurs at spatial scales closer to the rivers of origin than today’s mixed-stock marine fisheries, much as it did in the early 20th century (Lichatowich 2013; Price et al. 2013). Data requirements for place-based management are likely to be relatively high in the short term because of neglect on the part of management agencies to prioritize or fund regular monitoring of smaller spawning populations often caught in mixed-stock fisheries (Price et al. 2008, 2017; Lichatowich et al. 2017). There is a need to identify the diversity of component stocks in large terminal river systems, monitor the abundance and productivity of component populations, determine sustainable spawning escapement reference points (levels > = MSY), and determine effective harvest control rules through precautionary management strategy evaluations (e.g., Pestal et al. 2011).
In the long term, after stock-specific baseline data have been collected, data collection and analysis are likely no more costly than appropriately risk-averse management of aggregate populations in mixed-stock fisheries, and are certainly more compatible with the place-based character of salmon populations. For example, at regional levels (e.g., the Skeena River, the West Coast of Vancouver Island, northern Puget Sound, Washington (Oregon) tributaries to the Columbia River downstream of the Bonneville Dam) rotating panels of sites in selected river systems can be identified to systematically monitor component populations representative of the sizes and life histories of regional populations. Sampling can be conducted at appropriate frequencies (e.g., annually, once every three to five years) to acquire the requisite data for estimating escapement and harvest rates for individual populations. Once these sustainable escapement levels have been identified, the relationship among physical habitat characteristics (such as basin or floodplain area, e.g., Parken et al. 2006; Liermann et al. 2010), equilibrium abundance, and sustainable escapement levels can be robustly estimated and the results applied to unsurveyed populations with greater confidence than at present. Although robust cost estimates are needed, we expect that the costs of the data acquisition and monitoring necessary to support place-based MSY-plus management at the regional level will be no greater than that required to manage current mixed-stock marine salmon fisheries. Regardless, the costs of monitoring necessary to assure that wild populations are being sustainably managed should be borne by the fisheries as they are a component of the necessary management costs of the fisheries. Failure to undertake and fund such monitoring, thereby internalizing these management costs, treats the costs imposed on wild populations in the form of overfishing, under-escapement, population declines and (or) future lost fishing opportunities as externalities subsidized by the public, both present and future generations. Sustainability requires that such subsidies be eliminated (Lichatowich et al. 2017).
We do, however, acknowledge that it is possible, in principle, to manage aggregate stocks in a manner that accounts for the presence of component populations and to be consistent with place-based management. Such harvest requires a costly research-based adaptive management infrastructure that includes extensive sampling to acquire high-quality genetic stock identification data and a robust array of frequent spawner escapement estimates. These data would provide the information required to determine the impacts on all salmon populations encountered by a mixed-stock fishery and to follow those effects to the spawning grounds with sufficient detail and sampling frequency to determine with high confidence that population-specific sustainable escapement targets are being attained. Considerable progress has been made on the first component (development of genetic stock identification (GSI ) databases for most mixed-stock fishery aggregates on the US/Canada west coast, reviewed by Seeb et al. 2007 and Moran et al. 2013, Hess et al. 2014), but the resolution remains at the scale of large basin aggregates not individual component populations (though see Steele et al. 2013 for recent progress on stock-specific GSI and parentage-based tagging in the Columbia Basin). Until these elements are in place, mixed-stock marine salmon fisheries will fall short of the required sustainability standards (assuring and verifying regular attainment of MSY-based or greater spawner escapement and accounting for all by-catch and incidental mortality to assure that non-target salmon populations are not over-fished). A lower cost interim remedy for these fisheries would be the adoption of a robust MSY-plus management regime under which current MSY estimates are treated as limit thresholds.
Transitioning all, or a majority of, salmon management to the place-based approach will be challenging and will not occur overnight. Management of salmon harvest with selective gears and at more local scales than the mixed stock fisheries currently operate will, in the short term, not benefit everyone in commercial fishing communities. Some individuals will not be able to continue fishing with their current gears and may not be able to transition to selective practices and more terminal fisheries. In addition, overall harvest levels will likely have to be reduced. As well, many of the large-scale hatchery programs that currently subsidize unsustainable harvest levels with high ecological effects on depressed and at-risk wild populations (including adverse genetic effects from straying of hatchery fish to wild spawning grounds (HSRG 2009, 2015)) will have to be reduced in scale if not eliminated altogether. But these transition costs can be addressed, especially if planned for as part of a comprehensive reconfiguration of regional salmon fisheries. The criteria we have developed recognize the complexities of this transition and take into account the current state of salmon fisheries that complicate the ability to identify sustainable fishing practices. For example, the criteria require that candidate salmon sources frequently attain MSY-based escapement goals supported by well-documented evidence. Within fisheries that attain this escapement threshold, the criteria prioritize selective fishing gears that are passive (traps, reef nets) or close to passive (set gillnets) and then prioritize fisheries that land fish promptly while still alive and bleed and ice them soon after landing. There are few salmon fisheries that meet these criteria and have products available to conscientious retailers. The desire for such products should move salmon managers toward place-based management. Indeed, we endeavored to build a modicum of flexibility into the criteria to support salmon fisheries and products that strive to be consistent with the place-based philosophy at the beginning of this transition.
The short- and long-term benefit of such a transition is great. Place-based management with selective practices and gears will result in higher quality products due to more careful handling of individual fish. This will generate a premium sustainable product that provides a higher price to fishers per fish landed. Of equal importance, place-based, selective fishing practices, and gears such as salmon pound nets (traps), fish wheels, and reef nets, have a significantly lower carbon footprint than purse seine and gill net vessels operating in large rivers, estuaries, and the coastal nearshore. Consequently, fuel and related travel costs are much lower. Combined with management for larger and more consistently attained spawning escapement goals, place-based fisheries will have a higher probability of fishing opportunities on stronger wild salmon stocks than current mixed-stock fisheries. These features of place-based management and fisheries will reinvigorate local fishing economies and communities with resulting social benefits that are likely to be non-trivial.
Conclusion
Adoption of the criteria that we have developed will help retailers like Patagonia ensure that their products are derived from sustainable wild salmon fisheries. We believe that sustainable sourcing criteria for food product retailers can be simpler, more rigorous, and less ambiguous than large-scale certification processes. Our criteria (Box 1) require that the identities and abundance of populations encountered by candidate source fisheries are well-known and managed to consistently achieve escapements at or above MSY. By-caught salmon species are required to be equally well known and managed. Preference is given to selective harvest gears that are capable of releasing non-target species and populations with documented low or zero post-release mortality and target populations are handled in a manner that assures high flesh quality. The criteria do not allow for conditional approval of salmon fisheries that fall short of the criteria. This is in contrast to MSC “conditional” certifications for fisheries that do not meet MSC standards but still bear the MSC label. In our approach, fisheries must meet the criteria before their product can be eligible for sourcing to the retailer. Where doubt exists as to whether or not a candidate fishery meets the sourcing criteria, a scientific panel that is familiar with the criteria is required to provide a more detailed assessment of the fishery.
The current management paradigm that emphasizes marine mixed-stock fisheries is failing to protect and sustain wild salmon because it fails to recognize salmon as a plethora of place-based or locally adapted populations. Broad-scale certification processes that confer sustainable status to mixed-stock fisheries may perpetuate unsustainable harvest and mislead consumers who believe certifications are consistently held to high standards. In contrast, population portfolios conservatively harvested, managed, and monitored at the river-basin scale will have a greater probability of ensuring resiliency over the long term and of meeting ecosystem considerations. Rigorous sourcing criteria from retailers based on recognition of the place-based character of wild salmon provide an alternative that is fully capable of being more sustainable and transparent. Acceptance and application of this approach to providing sustainably sourced salmon products will provide an important contribution to the long-overdue transformation of salmon fisheries from unconstrained mixed-stock fisheries to place-based management of sustainable salmon population portfolios.
Acknowledgements
The authors wish to thank Dr. Carol Ann Woody, Center for Science in Public Participation; Matthew Stoecker, Stoecker Ecological; and Jamie Glasgow, Director of Science and Research, Wild Fish Conservancy, for comments on earlier versions of the sourcing criteria for Patagonia that greatly improved the substance and form of that document and helped us to clarify many of the issues developed in this paper. We are also grateful to Jim Lichatowich for numerous informative discussions regarding salmon fisheries management, sustainability, and the importance of conceptual foundations for providing clear priorities and guidance of management actions.
References
Braun DC, Patterson DA, and Reynolds JD. 2013. Maternal and environmental influences on egg size and juvenile life-history traits in Pacific salmon. Ecology and Evolution, 3(6): 1727–1740.
Cadrin SX, and Pastoors MA. 2008. Precautionary harvest policies and the uncertainty paradox. Fisheries Research, 94: 367–372.
Carlson SM, and Satterwaite WH. 2011. Weakened portfolio effect in a collapsed salmon population complex. Canadian Journal of Fisheries and Aquatic Sciences, 68: 1579–1589.
Cederholm CJ, Kunze MD, Murota T, and Sibatani A. 1999. Pacific salmon carcasses: essential contributions of nutrients and energy for aquatic and terrestrial ecosystems. Fisheries, 24(10): 6–15.
Charles AT. 2002. The precautionary approach and ‘burden of proof’ challenges in fishery management. Bulletin of Marine Sciences, 70(2): 683–694.
Chasco BE, Kaplan IC, Thomas AC, Acevedo-Gutiérrez A, Noren DP, Ford MJ, et al. 2017. Competing tradeoffs between increasing marine mammal predation and fisheries harvest of Chinook salmon. Scientific Reports, 7: 15439.
Christian C, Ainley D, Bailey M, Dayton P, Hocevar J, LeVine M, et al. 2013. A review of formal objections to Marine Stewardship Council fisheries certifications. Biological Conservation, 161: 10–17.
Copeland T, Ackerman MW, Wright KK, and Byrne A. 2017. Life history diversity of snake river steelhead populations between and within management categories. North American Journal of Fisheries Management, 37(2): 395–404.
Darimont CT, Bryan HM, Carlson SM, Hocking MD, MacDuffee M, Paquet PC, et al. 2010. Salmon for terrestrial protected areas. Conservation Letters, 3: 379–389.
Dayton PK. 1998. Reversal of the burden of proof in fisheries management. Science, 279: 821–822.
Einum S, Hendry AP, and Fleming IA. 2002. Egg-size evolution in aquatic environments: does oxygen availability constrain size? Proceedings of the Royal Society B, Biological Sciences, 269: 2325–2330.
Elliott KH, Elliott JE, Wilson LK, Jones I, and Stenerson K. 2011. Density-dependence in the survival and reproduction of bald eagles: linkages to chum salmon. The Journal of Wildlife Management, 75: 1688–1699.
Fleming IA, and Gross MR. 1990. Latitudinal clines: a trade-off between egg number and size in Pacific salmon. Ecology, 71: 1–11.
Ford JKB, Ellis GM, Olesiuk PF, and Balcomb KC. 2010. Linking killer whale survival and prey abundance: food limitation in the oceans’ apex predator? Biology Letters, 6: 139–142.
Fryer JK. 1998. Frequency of pinniped-caused scars and wounds on adult spring—summer Chinook and sockeye salmon returning to the Columbia River. North American Journal of Fisheries Management, 18(1): 46–51.
Gende SM, Edwards RT, Willson MF, and Wipfli MS. 2002. Pacific salmon in aquatic and terrestrial ecosystems. BioScience, 52(10): 917–928.
Gerrodette T, Dayton PK, Mecinko S, and Fogarty MJ. 2002. Precautionary management of marine fisheries: moving beyond burden of proof. Bulletin of Marine Science, 70(2): 657–668.
Groot C, and Margolis L. 1991. Pacific salmon life histories. University of British Columbia Press, Vancouver.
Harding JN, and Reynolds JD. 2014. Opposing forces: evaluating multiple ecological roles for Pacific salmon in coastal stream ecosystems. Ecosphere, 5(12): art157–22.
Hatchery Science Review Group. 2009. Report to Congress on Columbia River basin hatchery reform [online]: Available from hatcheryreform.us/wp-content/uploads/2016/05/HSRG-2009-Report-to-Congress.pdf.
Hatchery Science Review Group. 2015. Annual report to Congress on the science of hatcheries. [online]: Available from hatcheryreform.us/wp-content/uploads/2016/05/HSRG_Report-to-Congress_2015.pdf.
Heath DD, Heath JW, Bryden CA, Johnson RM, and Fox CW. 2003. Rapid evolution of egg size in captive salmon. Science, 299: 1738–1740.
Hendry AP, Castric V, Kinnison MT, and Quinn TP. 2004. The evolution of philopatry and dispersal: homing vs. straying in salmonids. In Evolution illuminated: salmon and their relatives. Edited by AP Hendry, and SC Stearns. Oxford University Press, Oxford, UK. pp. 52–91.
Hess JE, Whiteaker JM, Fryer JK, and Narum SR. 2014. Monitoring stock-specific abundance, run timing, and straying of Chinook salmon in the Columbia River using genetic stock identification (GSI). North American Journal of Fisheries Management, 34: 184–201.
Hutchings JA. 2011. Old wine in new bottles: reaction norms in salmonid fishes. Heredity, 106: 421–437.
Jacquet JL, and Pauly D. 2007. The rise of seafood awareness campaigns in an era of collapsing fisheries. Marine Policy, 31: 308–313.
Kirby DS, Visser C, and Hanich Q. 2014. Assessment of eco-labelling schemes for Pacific tuna fisheries. Marine Policy, 43: 132–142.
Knapp G, Roheim CA, and Anderson JL. 2007. Chapter 4. The role of hatcheries in North American salmon production. In The great salmon run: competition between wild and farmed salmon. A report prepared for TRAFFIC North America and the World Wildlife Fund. World Wildlife Fund, Washington D.C. 301 p.
Kohler AE, Kusnierz PC, Copeland T, Vendetti DA, Denny L, Gable J, et al. 2013. Salmon-mediated nutrient flux in selected streams of the Columbia River Basin, U.S.A. Canadian Journal of Fisheries and Aquatic Sciences, 70(3): 502–512.
Larkin PA. 1977. An epitaph for the concept of maximum sustained yield. Transactions of the American Fisheries Society, 106(1): 1–11.
Levi T, Dairmont CT, MacDuffee M, Mangel M, Pacquet P, and Williams CC. 2012. Using grizzly bears to assess harvest-ecosystem tradeoffs in salmon fisheries. PLoS Biology, 10(4): e1001303.
Lichatowich JA. 2013. Salmon, people, and place: a biologist’s search for salmon recovery. Oregon State University Press, Corvallis, Oregon.
Lichatowich JA, and Williams RN. 2009. Failures to incorporate science into fishery management and recovery programs: lessons from the Columbia River. American Fisheries Society Symposium, 70: 1005–1019.
Lichatowich JA, Williams R, Bakke B, Myron J, Bella D, McMillan B, et al. 2017. Wild pacific salmon: a threatened legacy. Bemis Printing, St. Helens, Oregon.
Liermann MC, Sharma R, and Parken CK. 2010. Using accessible watershed size to predict management parameters for Chinook salmon, Oncorhynchus tshawytscha, populations with little or no spawner-recruit data: a Bayesian hierarchical modelling approach. Fisheries Management and Ecology, 17: 40–51.
Mangel M, Marinovic B, Pomeroy C, and Croll D. 2002. Requiem for ricker: unpacking MSY. Bulletin of Marine Sciences, 70(2): 763–781.
Moore JW, McClure M, Rogers LA, and Schindler DE. 2010. Synchronization and portfolio performance of threatened salmon. Conservation Letters, 3: 340–348.
Moore JW, Yeakel JD, Peard D, Lough J, and Beere M. 2014. Life-history diversity and its importance to population stability and persistence of a migratory fish: steelhead in two large North American watersheds. Journal of Animal Ecology, 83(5): 1035–1046.
Moran P, Teel DJ, Banks MA, Beacham TD, Bellinger MR, Blankenship SM, et al. 2013. Divergent life-history races do not represent Chinook salmon coast-wide: the importance of scale in Quaternary biogeography. Canadian Journal of Fisheries and Aquatic Sciences, 70: 415–435.
NRC. 1996. Upstream. National Research Council, Washington D.C.
Parken CK, McNicol RE, and Irvine JR. 2006. Habitat-based methods to estimate escapement goals for data limited Chinook salmon stocks in British Columbia, 2004. Canadian Science Advisory Secretariat, Research Document 2006/083. Fisheries and Oceans Canada, Science Branch, Pacific Biological Station, Nanaimo, British Columbia.
Pestal G, Huang A-M, Cass A, and the FRSSI Working Group. 2011. Updated methods for assessing harvest rules for Fraser River sockeye salmon (Oncorhynchus nerka). Research Document 2011/133, Pacific Region, Canadian Science Advisory Secretariat, Ottawa, Ontario [online]: Available from dfo-mpo.gc.ca/csas-sccs/Publications/ResDocs-DocRech/2011/2011_133-eng.html.
Pikitch EK, Santora C, Babcock EA, Bakun A, Bonfil R, Conover DO, et al. 2004. Ecosystem-based fisheries management. Science, 305: 346–347.
Price MHH, Dairmont CT, Temple NF, and MacDuffee SM. 2008. Ghost runs: management and status assessment of Pacific salmon (Oncorhynchus spp.) returning to British Columbia’s central and north coast. Canadian Journal of Fisheries and Aquatic Sciences, 65: 2712–2718.
Price MHH, Gayeski N, and Stanford JA. 2013. Abundance of Skeena River chum salmon during the early rise of commercial fishing. Transactions of the American Fisheries Society, 142: 989–1004.
Price MHH, English KK, Rosenberger AG, MacDuffee M, and Reynolds JD. 2017. Canada’s wild salmon policy: an assessment of conservation progress in British Columbia. Canadian Journal of Fisheries and Aquatic Sciences, 74(10): 1507–1518.
Quinn TP. 2005. The behavior and ecology of pacific salmon and trout. University of Washington Press, Seattle, Washington.
Quinn TP, Kinnison MT, and Unwin MJ. 2001a. Evolution of chinook salmon (Oncorhynchus tshawytscha) populations in New Zealand: pattern, rate, and process. Genetica, 112–113: 493–513.
Quinn TP, Wetzel L, Bishop S, Overberg K, and Rogers DE. 2001b. Influence of breeding habitat on bear predation and age at maturity and sexual dimorphism of sockeye salmon populations. Canadian Journal of Zoology, 79: 1782–1793.
Radke HD, and Carter CN. 2009. Economic effects and social implications from Federal Mitchell Act funded hatcheries. Summary paper prepared for Native Fish Society. Native Fish Society, Portland, Oregon.
Sainsbury KJ, Punt AE, and Smith ADM. 2000. Design of operational management strategies for achieving fishery ecosystem objectives. ICES Journal of Marine Science, 57: 731–741.
Salvolainen O, Lascoux M, and Merilä J. 2013. Ecological genomics of local adaptation. Nature Reviews Genetics, 14: 807–820.
Satterthwaite WH, and Carlson SM. 2015. Weakening portfolio effect strength in a hatchery supplemented Chinook salmon population complex. Canadian Journal of Fisheries and Aquatic Sciences, 72(12): 1860–1875.
Schindler DE, Scheuerell MD, Moore JW, Gende SM, Francis TB, and Palen WJ. 2003. Pacific salmon and the ecology of coastal ecosystems. Frontiers in Ecology and the Environment, 1: 31–37.
Schindler DE, Hilborn R, Chasco B, Boatright CP, Quinn TP, Rogers LA, et al. 2010. Population diversity and the portfolio effect in an exploited species. Nature, 465: 609–612.
Seeb LW, Antonovich A, Banks MA, Beacham TD, Bellinger MR, Blankenship SM, et al. 2007. Development of a standardized DNA database for Chinook salmon. Fisheries, 32(11): 540–552.
Stanford JA, Lorang MS, and Hauer FR. 2005. The shifting habitat mosaic of river ecosystems. Internationale Vereinigung für Theoretische und Angewandte Limnologie: Verhandlungen, 29: 123–136.
Steele CA, Anderson EC, Ackerman MW, Hess MA, Campbell NR, Narum SR, et al. 2013. A validation of parentage-based tagging using hatchery steelhead in the Snake River basin. Canadian Journal of Fisheries and Aquatic Sciences, 70: 1046–1054.
Taylor EB. 1991. A review of local adaptations in Salmonidae with specific references to Pacific and Atlantic salmon. Aquaculture, 98: 185–207.
Waples RS, and Gaggiotti O. 2006. What is a population? An empirical evaluation of some genetic methods for identifying the number of gene pools and their degree of connectivity. Molecular Ecology, 15: 1419–1439.
Waples RS, Pess GR, and Beechie T. 2008. Evolutionary history of Pacific salmon in dynamic environments. Evolutionary Applications, 1: 189–206.
Waples RS, Luikart G, Faulkner JR, and Tallmon DA. 2013. Simple life-history traits explain key effective population size ratios across diverse taxa. Proceedings of the Royal Society B, Biological Sciences, 280: 20131339.
Williams R, Krkošek M, Ashe E, Branch TA, Clark S, Hammond PS, et al. 2011. Competing conservation objectives for predators and prey: estimating killer whale prey requirements for Chinook salmon. PLoS ONE, 6(11): e26738.
Wood CC. 1987. Predation of juvenile Pacific salmon by the common merganser (Mergus merganser) on eastern Vancouver Island. I: predation during the seaward migration. Canadian Journal of Fisheries and Aquatic Sciences, 44: 941–949.
Information & Authors
Information
Published In
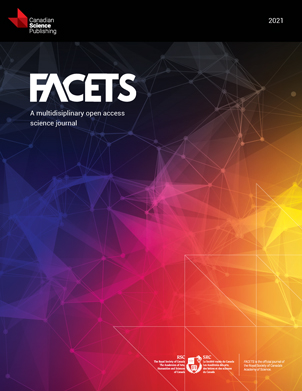
FACETS
Volume 3 • Number 1 • October 2018
Pages: 300 - 314
Editor: Steven J. Cooke
History
Received: 30 December 2016
Accepted: 14 December 2017
Version of record online: 19 March 2018
Copyright
© 2018 Gayeski et al. This work is licensed under a Creative Commons Attribution 4.0 International License (CC BY 4.0), which permits unrestricted use, distribution, and reproduction in any medium, provided the original author(s) and source are credited.
Data Availability Statement
All relevant data are within the paper.
Key Words
Sections
Subjects
Authors
Author Contributions
All conceived and designed the study.
All contributed resources.
NG drafted or revised the manuscript.
Competing Interests
The authors have declared that no competing interests exist.
Metrics & Citations
Metrics
Other Metrics
Citations
Cite As
Nick Gayeski, Misty MacDuffee, and Jack A. Stanford. 2018. Criteria for a good catch: A conceptual framework to guide sourcing of sustainable salmon fisheries. FACETS.
3(1): 300-314. https://doi.org/10.1139/facets-2016-0078
Export Citations
If you have the appropriate software installed, you can download article citation data to the citation manager of your choice. Simply select your manager software from the list below and click Download.
Cited by
1. Responsible sourcing in the food industry: a scoping review
2. Indigenous Systems of Management for Culturally and Ecologically Resilient Pacific Salmon (
Oncorhynchus
spp.) Fisheries
3. Modified Commercial Fish Trap to Help Eliminate Salmonid Bycatch Mortality
4. Survival of Salmonids from an Experimental Commercial Fish Trap