Range extension for the region of sympatry between the nudibranchs Hermissenda opalescens and Hermissenda crassicornis in the northeastern Pacific
Abstract
The mollusc nudibranch genus Hermissenda Bergh, 1879 was recently discovered to include three pseudocryptic species, dividing a single species H. crassicornis (sensu lato) into H. crassicornis Escholtz, 1831, H. opalescens J.G. Cooper, 1863, and H. emurai Baba, 1937. The species were distinguished by both genetic and morphological evidence, and the distribution of sampled animals suggested the three species had mostly distinct geographical ranges. Here, we report the presence of both H. crassicornis and H. opalescens in Barkley and Clayoquot Sounds, British Columbia, Canada, based on diagnostic characters and molecular data congruent with the differences described for these two species. This result extends the region of sympatry for the two species from northern California, USA, to, at least, Vancouver Island, British Columbia in 2016. Depending on how long this overlap has occurred, the possible northward expansion of H. opalescens would have implications for understanding the effects of short- or long-term environmental changes in ocean temperatures as well as complicating the interpretation of past neurobiological studies of H. crassicornis (sensu lato).
Introduction
The heterobranch sea slug genus Hermissenda Bergh, 1879 was recently determined to include three pseudocryptic species: H. crassicornis Escholtz, 1831; H. opalescens J.G. Cooper, 1863; and H. emurai Baba, 1937 (Lindsay and Valdés 2016). The three species were distinguished by genetic analyses, morphological characters, and geographic ranges in the North Pacific (Table 1). This division has resurrected the three species names originally described in the genus that had previously been unified into a single trans-Pacific species, H. crassicornis (sensu lato) (O’Donoghue 1922). Beyond the biogeographic and evolutionary implications of recognizing the three species anew, the discovery that H. crassicornis (sensu lato) was a species complex may have important ramifications for interpreting more than 40 years of neurobiological study of the animals (e.g., Alkon 1973, 1980; Crow 2004; Blackwell 2006; Cavallo et al. 2014; Gunaratne et al. 2014; Gunaratne and Katz 2016; Webber et al. 2017). It is possible that phenotypic heterogeneity amongst the species includes both neural and behavioural characters, and thus, care may be needed in integrating conclusions made in studies with animals from different locations. Gathering information on the respective species ranges is important for such retrospective work and would also be valuable in advance of further studies of Hermissenda (biogeographical, electrophysiological, or otherwise).
Table 1.
Character | H. crassicornis | H. opalescens | H. emurai |
---|---|---|---|
Range | Alaska–Northern California | Northern California–Sea of Cortez | Japan–Russian Far East |
Body shape | Short | Short | Elongate |
Body colour | Translucent to white | Translucent to white | Orange |
Longitudinal stripe between rhinophores | Orange (light to bright in hue) | Orange (light to bright in hue) | Orange (dark to almost red) |
Cerata background colour | Range from light to dark brown; may also be bright orange | Range from light to dark brown; may also be bright orange | Range from light to dark brown; may also be bright orange |
Cerata tip colour | Not included in description | May or may not contain reddish to brown tipping | May or may not contain reddish to brown tipping |
White cerata stripe | Present | Absent | Absent |
Cerata group gaps | Small | Small | Large |
The evidence presented by Lindsay and Valdés (2016) indicated that both H. crassicornis and H. opalescens are present along the Pacific coast of North America. H. opalescens is a more southern species, found between Baja, California, Mexico, and Bodega Bay, California, USA, whereas H. crassicornis ranges from Alaska to central California. Based on the specimens examined, the species’ ranges should overlap along a relatively short stretch of coastline in central and northern California. However, unverified photographic records from the world wide web suggest H. opalescens may be present farther north (inaturalist.org/taxa/494603-Hermissenda-opalescens). The photos show specimens lacking white stripes on their cerata, matching the only morphological character distinguishing H. opalescens (stripe absent) from H. crassicornis (stripe present), as described by Lindsay and Valdés (2016). The northwestern Pacific species, H. emurai, also lacks the white stripe, but has a different body shape, colouration, and different spacing between groups of cerata, eliminating it from consideration. Furthermore, our initial observations in the summer of 2016 suggested that H. crassicornis (with a stripe) and H. opalescens (without a stripe) were both common on the west coast of Vancouver Island, British Columbia, Canada. Thus, our goals for this study were (i) to survey these locations on Vancouver Island to determine which species were present, and (ii) to confirm whether any morphological differences between species were consistent with those noted by Lindsay and Valdés (2016).
Materials and methods
Specimen collection
All animal collection and care followed the Canadian Council for Animal Care guidelines. Specimens were collected opportunistically from seven locations in Barkley Sound and one location in Clayoquot Sound, British Columbia (Fig. 1; Table S1). Collection sites included floating docks, intertidal zones, and subtidal habitats (sampled by SCUBA), spanning sites from 0 to 30 m in depth. Initial specimen collection occurred between 1–15 July 2016 at all sites, followed by further specimen collection at only a subset of sites in Barkley Sound between 25 August 2016 and 1 September 2016.
Fig. 1.

Measurements
Following collection, animals from different sites were individually segregated in mesh containers and kept in flow-through sea tables at Bamfield Marine Sciences Centre until measured. All individuals were photographed with either an Olympus STYLUS TG-3 Tough or a Pentax WG-3 camera. For morphometrics, photographs were taken of the dorsal, lateral, and ventral views (while specimens were crawling on a transparent surface). For each specimen, several morphological observations and measurements were tabulated, including ceratal and body colouration patterns, foot length (at the midline), foot width (at its widest point), foot area and perimeter (from a manual outline of the foot), and gap length between the first and second ceratal clusters (this was only measured in some specimens due to difficulties visualizing the gap). Specimens with broken tails were excluded from allometric analyses.
Software and statistical analyses
All measurements were made in ImageJ v1.50 and analyzed in R v3.3.0 with the ggplot2 package (Wickham 2009; R Core Team 2016). To assess whether foot width differed between the two species after adjusting for differences in foot length, we used analysis of covariance (ANCOVA) on log10-transformed data with species (identified based on the presence or absence of stripes on the cerata) and foot length as explanatory variables and foot width as the response variable (Jasieński and Bazzaz 1999).
DNA extraction, sequencing, and data analysis
Tissue samples preserved in 95% ethanol from five specimens matching the external colour pattern of both H. crassicornis (n = 3) and H. opalescens (n = 2) were obtained. One of each species was submitted to the Canadian Center for DNA Barcoding, University of Guelph, Canada (CCDB) for sequence analysis. DNA extraction, PCR amplification of the cytochrome c oxidase I (COI) barcode region, and DNA sequencing were performed at the CCDB following standard high-throughput protocols (Steinke et al. 2016). Complete voucher data, images, and GenBank accession numbers for these two specimens and all other Hermissenda records on BOLD (Ratnasingham and Hebert 2007) are available in the public dataset DS-HERMCOI under the DOI: https://doi.org/10.5883/DS-HERMCOI.
We processed the remaining three specimens ourselves. Genomic DNA was extracted using a DNeasy kit (Qiagen, Hilden, Germany) following the manufacturer protocols. Polymerase chain reaction (PCR) was used to amplify fragments of gene COI in a final volume of 50 μL per sample. Each PCR sample included deionized water, 10× DreamTaq buffer, 10 μmol/L dNTPs, 10 μmol/L universal primers HCO2198 (5’-TAAACTTCAGGGTGACCAAAAAATCA-3’) and LCOI490 (5’-GGTCAACAAATCATAAAGATATTGG-3’) (Folmer et al. 1994), and 5 mg/mL DreamTaq (Thermo Fisher Scientific, Waltham, Massachusetts, USA) to amplify the regions of interest. The samples underwent an initial denaturation step of 95 °C for 3 min followed by an annealing period of 35 cycles of 94 °C for 45 s, 50 °C for 45 s, and 72 °C for 2 min, and a final extension period of 72 °C for 10 min. Agarose gel electrophoresis with ethidium bromide was used to detect the presence of DNA. Sequencing was outsourced to Source Bioscience Inc. (Santa Fe Springs, California, USA). Sequences were assembled and edited using Geneious Pro R8 (Kearse et al. 2012) and deposited in GenBank (accession numbers MH137939–MH137941). The geographic distribution and structure of the haplotypes was visualized by producing a haplotype network using the program PopArt v. 1.7 (Leigh et al. 2015) using the TCS option. Sequences were compared with data from both species reported by Lindsay and Valdés (2016) and obtained from GenBank (Table S2).
Results
Specimens of Hermissenda collected from both Barkley and Clayoquot Sounds included members of both H. crassicornis (sensu stricto) and H. opalescens (Fig. 2), based on the presence or absence of longitudinal white stripes on the anterior portion of the cerata (Tables 1 and 2). In July 2016, the proportion of individuals identified as H. crassicornis (with white ceratal stripes) was 81% (51 out of a total of 63) in Barkley Sound and 25% (8 out of a total of 32) in Clayoquot Sound were identified as H. crassicornis (Fig. S1). The remaining animals lacked white ceratal stripes and, thus, were identified as H. opalescens. Specimen collection in late August and early September 2016 in Barkley Sound recovered 96% (71 out of a total of 74) H. crassicornis and only 4% H. opalescens.
Fig. 2.

Table 2.
Character | H. crassicornis | H. opalescens |
---|---|---|
White stripe on cerata | Present | Absent |
Colour on tip of cerata | Orange | White |
Foot width relative to length | Narrower | Wider |
Our observations consistently showed one additional colouration difference between the species, apart from the diagnostic presence or absence of white ceratal stripes (Table 2). H. crassicornis had a tapered orange section at the distal ends of the cerata, with a very small translucent section at the apex. In contrast, in H. opalescens, the sections toward the distal ends of the cerata were distinctively white (again, with the small translucent section at the very tips). The two species otherwise shared patterns of colouration. The longitudinal stripe between the rhinophores was orange with a blue outline in all individuals of both species, whereas the background colour of the cerata varied from black to brown to orange among individuals of both species.
Measurements of various body dimensions also showed some consistent differences between the specimens we collected. Animals identified as H. opalescens were consistently larger than those identified as H. crassicornis (e.g., foot length with no stripe: 46 ± 2.3 mm, and with stripe: 29 ± 0.8 mm; mean ± SE) with minimal overlap in size between the two species in our sample. Foot shape differed between species (Fig. 3); relative to a standard foot length, H. crassicornis had proportionally narrower feet than H. opalescens (ANCOVA species effect: F1,112 = 677.99, P < 0.0001; Fig. 4), and the slope of the relationship between foot length and foot width did not differ between the species (ANCOVA interaction term: F1,112 = 1.42, P = 0.2364; Fig. 4). No other morphometric differences were evident.
Fig. 3.

Fig. 4.

COI sequence data revealed the existence of five different haplotypes among the five specimens sequenced. In the haplotype network analysis (Fig. 5), two of the sequences (from animals without ceratal stripes) clustered with other specimens of H. opalescens obtained by Lindsay and Valdés (2016) from Mexico and southern California. The other three sequences (from animals with white ceratal stripes) clustered with samples of H. crassicornis ranging from northern California to Alaska.
Fig. 5.

Discussion and conclusions
We observed two species of Hermissenda on the west coast of Vancouver Island in the summer of 2016. The characteristics for each were consistent with those described for H. crassicornis (with white stripes on the cerata) and H. opalescens (without the stripes) (Lindsay and Valdés 2016) and field identification was confirmed with molecular data from five individuals belonging to the two species. Moreover, we identified two additional differences in morphology: colouration of the distal ends of the cerata (excluding the very tips of the cerata, which were translucent in both species), and foot shape (at least for larger individuals). In the habitats we sampled, H. crassicornis had white ceratal stripes and an orange section on the tips of their cerata, and were smaller overall with proportionately narrower feet. H. opalescens had no white stripes on their cerata, the distal sections of the cerata were white, and the animals were consistently larger with proportionately wider feet. This difference in foot shape may or may not be a consequence of genuine differences between the species. Most of the H. crassicornis specimens we collected were smaller than the H. opalescens specimens we collected, so we cannot be certain that the difference in foot shape is maintained at comparable sizes. Instead, it may be that a change in shape arises during the growth of both species, but only the H. opalescens we collected had grown large enough to display the wider foot shape. It is, therefore, possible that the different Hermissenda foot shapes are a consequence of comparing different stages in parallel ontogenies (or some other form of shared phenotypic plasticity). However, no changes in foot shape have been reported in past studies of adult growth of Hermissenda spp. (Harrigan and Alkon 1978). Further investigation of the foot shape of small H. opalescens and large H. crassicornis will resolve whether the species consistently differ in foot shape at all sizes.
The presence of H. opalescens on the west coast of Vancouver Island constitutes a range extension for the species, which was previously described as only occurring as far north as Bodega Bay, California. A number of other anecdotal reports have also recorded Hermissenda individuals lacking ceratal stripes in both Oregon and Washington (J. Goddard, B. Green, K. Fletcher, D. Miller, T. Prestholdt, personal communication, 2017; Fig. 1). One possible reason for this apparent range extension is that H. opalescens may always have had a more northerly range. Hermissenda populations rapidly increase and decrease, and earlier sampling efforts may simply have missed the blooms of H. opalescens north of California. However, photos taken before 2016 by ourselves and contributors to The Sea Slug Forum (seaslugforum.net) show only animals with white ceratal stripes in Washington, Oregon, and British Columbia (Lindsay and Valdés 2016). This suggests that the presence of H. opalescens in British Columbia is a novel phenomenon. Northward range shifts in the northeast Pacific have also been documented over recent years for several other nudibranchs (Goddard et al. 2016), mole crabs (Wonham and Hart 2018), copepods (Peterson et al. 2017), several species of fish (Auth et al. 2018; Halpin et al. 2018a), and cetaceans (Halpin et al. 2018b). In the summer of 2016, we also recorded anecdotal observations of other southern species in the waters near the Bamfield Marine Sciences Centre on Vancouver Island, including the nudibranch, Dirona picta MacFarland, 1905 (C. Tamis, R. Wyeth, personal observation, 2016), striped shore crabs, Pachygrapsus crassipes Randall, 1840 (C. Neufeld, T. Eastham, personal observation, 2016), and brown pelicans Pelecanus occidentalis Linnaeus, 1766 (S. Gray, personal communication, 2016).
If the geographic range of H. opalescens is shifting northward, several possible mechanisms could explain this phenomenon. Given the broad diet of Hermissenda spp. (Avila and Kuzirian 1995), distributions are probably not restricted by prey availability, but rather driven by other factors such as survival and dispersal of the planktonic larvae (Harrigan and Alkon 1978), combined with adult tolerances for abiotic conditions. Optimal laboratory culture conditions have been explored for both larvae and adults (Harrigan and Alkon 1978; Rutowski 1983; Avila et al. 1997, 1998; Avila 1998). (The primary source of animals for these past studies was near Monterey Bay, California, approximately 200 km southward of the region of overlap for the two species (Lindsay and Valdés 2016), and therefore we presume they apply to H. opalescens.) However, these studies do not explore environmental tolerances that might set geographic limits for larvae or adults. Thus, we have limited information to infer range limits for either species without further experimentation.
An alternative approach could be to determine how conditions in northern regions are changing relative to historical conditions at southern locations with established records of H. opalescens. Long-term warming trends in the Pacific, periodic warming as part of El Nino events, or the short-term North Pacific marine heat wave of 2014–2015 (Johnstone and Mantua 2014; Di Lorenzo and Mantua 2016; Jacox et al. 2016) all could have allowed H. opalescens to settle and grow successfully in the normally colder waters of Vancouver Island. Historically, the average annual sea surface temperatures are 1–3 °C warmer near Monterey Bay than Vancouver Island (NASA Worldview, worldview.earthdata.nasa.gov/), matching the approximately +2 °C temperature anomaly that occurred in 2015 (Di Lorenzo and Mantua 2016). Changes in ocean current patterns are also important to consider, given they have been shown to be a key driver in nudibranch recruitment, at least in California (Schultz et al. 2011). Either an increase in northward advection of the California Current (Kosro 2002; Schwing et al. 2003) or changes in upwelling (Jacox et al. 2015) and cross-shelf advection (causing longer pelagic transport of larvae) could lead to northern range expansions, as long as no thermal tolerance limits were encountered. Further monitoring is needed to determine if H. opalescens populations persist, and analysis of historical records and archival specimens are needed to establish more clearly when and where H. opalescens has been present north of California.
Our observations have important implications for interpreting other studies of Hermissenda. At least temporarily, the region of potential sympatry between H. crassicornis and H. opalescens extended from northern California to Vancouver Island in 2016. Depending on how long this range overlap has existed, earlier studies purportedly of H. crassicornis may have in fact been studying H. opalescens or a mixture of the two species. In particular, interpretations of the extensive history of neurobiological research with animals collected from various locations in California should now consider the potential effects of species-specific differences in neural circuits or behaviour (e.g., Alkon 1973, 1980; Cavallo et al. 2014; Gunaratne et al. 2014; Gunaratne and Katz 2016; Webber et al. 2017). In the future, careful species identification is required for all studies of Hermissenda along the northeast Pacific coast of North America, and further sampling is needed to verify both the northern range boundary for H. opalescens and the southern range boundary for H. crassicornis.
Acknowledgements
We acknowledge the support of the director, university programs, and other staff of Bamfield Marine Sciences Centre, and the Natural Sciences and Engineering Research Council. We thank K. Murphy, P. O’Brien, E. Maltby, S. Johnston, R. Munger, C. Tamis, and S. Gray for help with specimen collections and J. Goddard, B. Green, K. Fletcher, D. Miller, and T. Prestholdt for information on H. opalescens presence in Oregon and Washington. We also thank two anonymous reviewers for their helpful reviews of this manuscript. Funding provided by the Natural Sciences and Engineering Research Council of Canada (grant no. RGPIN-2015-04957 to RCW) the National Institutes of Health (grant no. 5R25GM113748-02, supporting ALKE-P).
References
Alkon DL. 1973. Neural organization of a molluscan visual system. Journal of General Physiology, 61(4): 444–461.
Alkon DL. 1980. Cellular analysis of a gastropod (Hermissenda crassicornis) model of associative learning. The Biological Bulletin, 159(3): 505–560.
Auth TD, Daly EA, Brodeur RD, and Fisher JL. 2018. Phenological and distributional shifts in ichthyoplankton associated with recent warming in the northeast Pacific Ocean. Global Change Biology, 24(1): 259–272.
Avila C. 1998. Competence and metamorphosis in the long-term planktotrophic larvae of the nudibranch mollusc Hermissenda crassicornis (Eschscholtz, 1831). Journal of Experimental Marine Biology and Ecology, 231(1): 81–117.
Avila C, and Kuzirian AM. 1995. Natural diets for Hermissenda crassicornis mariculture. The Biological Bulletin, 189(2): 237–238.
Avila C, Grenier S, Tamse CT, and Kuzirian AM. 1997. Biological factors affecting larval growth in the nudibranch mollusc Hermissenda crassicornis (Eschscholtz, 1831). Journal of Experimental Marine Biology and Ecology, 218(2): 243–262.
Avila C, Tyndale E, and Kuzirian AM. 1998. Feeding behavior and growth of Hermissenda crassicornis (Mollusca: Nudibranchia) in the laboratory. Marine and Freshwater Behaviour and Physiology, 31(1): 1–19.
Bivand R, Lewin-Koh N, Pebesma E, Archer E, Baddeley A, Bearman N, et al. 2017a. maptools: tools for reading and handling spatial objects [online]: Available from CRAN.R-project.org/package=maptools.
Bivand R, Rundel C, Pebesma E, Stuetz R, and Hufthammer KO. 2017b. rgeos: interface to Geometry Engine—Open Source (‘GEOS’) [online]: Available from CRAN.R-project.org/package=rgeos.
Blackwell KT. 2006. Subcellular, cellular, and circuit mechanisms underlying classical conditioning in Hermissenda crassicornis. The Anatomical Record Part B: The New Anatomist, 289B(1): 25–37.
Brownrigg R, Wilks AR, and Becker RA. 2018. mapdata: extra map databases [online]: Available from CRAN.R-project.org/package=mapdata.
Cavallo JS, Hamilton BN, and Farley J. 2014. Behavioral and neural bases of extinction learning in Hermissenda. Frontiers in Behavioral Neuroscience, 8: 277.
Crow T. 2004. Pavlovian conditioning of Hermissenda: current cellular, molecular, and circuit perspectives. Learning and Memory, 11(3): 229–238.
Deckmyn A, Minka TP, Brownrigg R, Wilks AR, and Becker RA. 2018. maps: draw geographical maps [online]: Available from CRAN.R-project.org/package=maps.
Di Lorenzo E, and Mantua N. 2016. Multi-year persistence of the 2014/15 North Pacific marine heatwave. Nature Climate Change, 6(11): 1042–1047.
Folmer O, Black M, Hoeh W, Lutz R, and Vrijenhoek R. 1994. DNA primers for amplification of mitochondrial cytochrome c oxidase subunit I from diverse metazoan invertebrates. Molecular Marine Biology and Biotechnology, 3(5): 294–299.
Goddard JHR, Treneman N, Pence WE, Mason DE, Dobry PM, Green B, et al. 2016. Nudibranch range shifts associated with the 2014 warm anomaly in the Northeast Pacific. Bulletin, Southern California Academy of Sciences, 115(1): 15–40.
Gunaratne CA, and Katz PS. 2016. Comparative mapping of GABA-immunoreactive neurons in the buccal ganglia of nudipleura molluscs. The Journal of Comparative Neurology, 524(6): 1181–1192.
Gunaratne CA, Sakurai A, and Katz PS. 2014. Comparative mapping of GABA-immunoreactive neurons in the central nervous systems of nudibranch molluscs. The Journal of Comparative Neurology, 522(4): 794–810.
Halpin LR, Galbraith M, and Morgan KH. 2018a. The first swordfish (Xiphias gladius) recorded in coastal British Columbia. Northwestern Naturalist, 99(1): 63–65.
Halpin LR, Towers JR, and Ford JKB. 2018b. First record of common bottlenose dolphin (Tursiops truncatus) in Canadian Pacific waters. Marine Biodiversity Records, 11: 3.
Harrigan JF, and Alkon DL. 1978. Larval rearing, metamorphosis, growth and reproduction of the eolid nudibranch Hermissenda crassicornis (Eschscholtz, 1831) (Gastropoda: Opisthobranchia). The Biological Bulletin, 154(3): 430–439.
Hijmans RJ, van Etten J, Cheng J, Mattiuzzi M, Sumner M, Greenberg JA, et al. 2017. raster: geographic data analysis and modeling [online]: Available from CRAN.R-project.org/package=raster.
Jacox MG, Fiechter J, Moore AM, and Edwards CA. 2015. ENSO and the California Current coastal upwelling response. Journal of Geophysical Research: Oceans, 120(3): 1691–1702.
Jacox MG, Hazen EL, Zaba KD, Rudnick DL, Edwards CA, Moore AM, et al. 2016. Impacts of the 2015–2016 El Niño on the California Current System: early assessment and comparison to past events. Geophysical Research Letters, 43(13): 7072–7080.
Jasieński M, and Bazzaz FA. 1999. The fallacy of ratios and the testability of models in biology. Oikos, 84(2): 321–326.
Johnstone JA, and Mantua NJ. 2014. Atmospheric controls on northeast Pacific temperature variability and change, 1900–2012. Proceedings of the National Academy of Sciences of the USA, 111(40): 14360–14365.
Kearse M, Moir R, Wilson A, Stones-Havas S, Cheung M, Sturrock S, et al. 2012. Geneious Basic: an integrated and extendable desktop software platform for the organization and analysis of sequence data. Bioinformatics, 28(12): 1647–1649.
Kosro PM. 2002. A poleward jet and an equatorward undercurrent observed off Oregon and northern California, during the 1997–98 El Niño. Progress in Oceanography, 54(1): 343–360.
Leigh JW, Bryant D, and Nakagawa S. 2015. popart: full-feature software for haplotype network construction. Methods in Ecology and Evolution, 6(9): 1110–1116.
Lindsay T, and Valdés Á. 2016. The model organism Hermissenda crassicornis (Gastropoda: Heterobranchia) is a species complex. PLoS ONE, 11(4): e0154265.
O’Donoghue CH. 1922. Notes on the taxonomy of nudibranchiate Mollusca from the Pacific Coast of North America. I. On the identification of Cavolina (i.e. Hermissenda crassicornis of Eschscholtz. The Nautilus, 35: 74–77.
Peterson WT, Fisher JL, Strub PT, Du X, Risien C, Peterson J, et al. 2017. The pelagic ecosystem in the Northern California Current off Oregon during the 2014–2016 warm anomalies within the context of the past 20 years. Journal of Geophysical Research: Oceans, 122(9): 7267–7290.
R Core Team. 2016. R: a language and environment for statistical computing. Vienna, Austria: R Foundation for Statistical Computing [online]: Available from r-project.org.
R Core Team. 2017. R: a language and environment for statistical computing. Vienna, Austria: R Foundation for Statistical Computing [online]: Available from r-project.org.
Ratnasingham S, and Hebert PDN. 2007. bold: The Barcode of Life Data System. Molecular Ecology Notes, 7(3): 355–364. [online]: Available from barcodinglife.org.
Rutowski RL. 1983. Mating and egg mass production in the aeolid nudibranch Hermissenda crassicornis (Gastropoda: Opisthobranchia). The Biological Bulletin, 165(1): 276–285.
Schultz ST, Goddard JHR, Gosliner TM, Mason DE, Pence WE, McDonald GR, et al. 2011. Climate-index response profiling indicates larval transport is driving population fluctuations in nudibranch gastropods from the northeast Pacific Ocean. Limnology and Oceanography, 56(2): 749–763.
Schwing FB, Palacios DM, Bograd SJ, and Pacific Grove CA. 2003. El Niño impacts on the California current ecosystem. US CLIVAR Newsletter, 3(2): 5–8.
Soetaert K. 2018. shape: functions for plotting graphical shapes, colors [online]: Available from CRAN.R-project.org/package=shape.
Steinke D, Prosser SWJ, and Hebert PDN. 2016. DNA barcoding of marine metazoans. In Marine genomics. Edited by S Bourlat. Humana Press, New York City, New York. pp. 155–168.
Webber MP, Thomson JWS, Buckland-Nicks J, Croll RP, and Wyeth RC. 2017. GABA-, histamine-, and FMRFamide-immunoreactivity in the visual, vestibular and central nervous systems of Hermissenda crassicornis. The Journal of Comparative Neurology, 525(16): 3514–3528.
Wickham H. 2009. ggplot2: elegant graphics for data analysis. Springer Verlag, New York City, New York.
Wickham H, and RStudio. 2017. scales: scale functions for visualization [online]: Available from CRAN.R-project.org/package=scales.
Wonham MJ, and Hart MW. 2018. El Niño range extensions of pacific sand crab (Emerita analoga) in the Northeastern Pacific. Northwest Science, 92(1): 53–60.
Supplementary Materials
Supplementary Material 1 (DOCX / 29.6 KB)
- Download
- 36.05 KB
Supplementary Material 2 (JPG / 17.6 KB)
- Download
- 17.68 MB
Information & Authors
Information
Published In
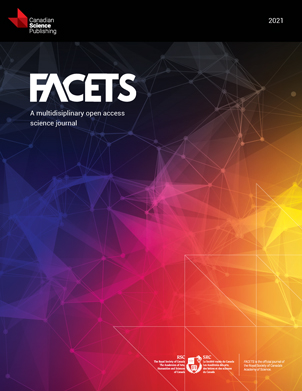
FACETS
Volume 3 • Number 1 • October 2018
Pages: 764 - 776
Editor: Jeremy Kerr
History
Received: 30 May 2017
Accepted: 25 May 2018
Version of record online: 19 July 2018
Copyright
© 2018 Merlo et al. This work is licensed under a Creative Commons Attribution 4.0 International License (CC BY 4.0), which permits unrestricted use, distribution, and reproduction in any medium, provided the original author(s) and source are credited.
Data Availability Statement
All relevant data are within the paper, in GenBank (ncbi.nlm.nih.gov/genbank/; accession numbers MH137939–MH137941), and on BOLD (complete voucher data, images, and GenBank accession numbers for the two specimen samples from this study sent to the Canadian Center for DNA Barcoding (see Methods) and all other Hermissenda records on BOLD (Ratnasingham and Hebert 2007) are available in the public dataset DS-HERMCOI under the DOI: https://doi.org/10.5883/DS-HERMCOI).
Key Words
Sections
Subjects
Plain Language Summary
Californian opalescent sea slugs spreading northwards to British Columbia, at least temporarily
Authors
Author Contributions
EMM, KAM, NBS, CJN, TME, and RCW conceived and designed the study.
EMM, KAM, NBS, ALKE-P, DS, and PDNH performed the experiments/collected the data.
EMM, KAM, NBS, CJN, ALKE-P, AV, and RCW analyzed and interpreted the data.
DS, PDNH, AV, and RCW contributed resources.
EMM, KAM, NBS, CJN, TME, AV, and RCW drafted or revised the manuscript.
Competing Interests
The authors have declared that no competing interests exist.
Metrics & Citations
Metrics
Other Metrics
Citations
Cite As
Emily M. Merlo, Kathryn A. Milligan, Nola B. Sheets, Christopher J. Neufeld, Tao M. Eastham, A.L. Ka’ala Estores-Pacheco, Dirk Steinke, Paul D.N. Hebert, Ángel Valdés, and Russell C. Wyeth. 2018. Range extension for the region of sympatry between the nudibranchs Hermissenda opalescens and Hermissenda crassicornis in the northeastern Pacific. FACETS.
3(1): 764-776. https://doi.org/10.1139/facets-2017-0060
Export Citations
If you have the appropriate software installed, you can download article citation data to the citation manager of your choice. Simply select your manager software from the list below and click Download.
Cited by
1. Why didn’t the nudibranch cross the ocean? Understanding biogeographic and evolutionary relationships of
Hermissenda
(Nudibranchia: Myrrhinidae) Bergh, 1878
2. Looking at the Nudibranch Family Myrrhinidae (Gastropoda, Heterobranchia) from a Mitochondrial ‘2D Folding Structure’ Point of View
3. The return of the clown: pseudocryptic speciation in the North Pacific clown nudibranch, Triopha catalinae (Cooper, 1863) sensu lato identified by integrative taxonomic approaches
4. High Heat Tolerance Is Negatively Correlated with Heat Tolerance Plasticity in Nudibranch Mollusks