The role of management instruments in the diversion of organic municipal solid waste and phosphorus recycling
Abstract
Graphical Abstract

Introduction
Organic solid waste diversion management and policy: Europe and Canada
Organic solid waste diversion and policy: European Union
Organic solid waste diversion and policy: Ontario, Canada
Methods
Data collection and synthesis
Waste diversion in Europe
Waste diversion in Ontario
Waste diversion factors
Category | Group | Measurement |
---|---|---|
Socio-economic | Income | Mean income per capita |
Population | Total population | |
Population density | ||
Management | Economic incentives/disincentives | Landfill taxes and fees |
Incineration taxes and fees | ||
Pay-as-you-throw schemes | ||
Legislation and policy | Regional waste management regulations | |
Curbside bag limits | ||
Mandatory source separation of waste streams | ||
Centralized infrastructure | Presence of centralized infrastructure for residential source-separated organics |
P recycling in diverted organics
Data analysis: organic solid waste diversion
Relationship with organic waste diversion rate | |||
---|---|---|---|
Variable name | Variable labela | adjusted R2 | Significance |
Europe (OFMSW diversion) | |||
Income (International $/capitab) | IN | 0.506 | <0.001 |
Population density (No. inhabitants/km2) | PD | 0.011 | <1 |
Landfill charge (€/tonne) | LF | 0.435 | <0.001 |
Pay-as-you-throw | PAYT | 0.238 | <0.01 |
Legislation | LEG (0) | 0.232 | <0.05 |
LEG (1) | |||
LEG (2) | |||
Mandatory separation | MS | 0.097 | <0.1 |
Incineration tax | IT | 0.182 | <0.05 |
Incineration ban | IB | −0.028 | <1 |
Europe (Δ OFMSW diversion) | |||
Income (International $/capitab) | IN | 0.132 | <0.05 |
Population density (No. inhabitants/km2) | PD | −0.008 | <1 |
Landfill charge (€/tonne) | LF | 0.209 | <0.01 |
Pay-as-you-throw | PAYT | 0.09 | <0.1 |
Legislation | LEG (0) | −0.038 | — |
LEG (1) | −0.038 | <1 | |
LEG (2) | −0.038 | — | |
Mandatory separation | MS | −0.01 | <1 |
Incineration tax | IT | −0.036 | <1 |
Incineration ban | IB | 0.034 | <1 |
Ontario (OFMSW diversion) | |||
Income (CAD $/capita) | IN | 0.006 | <1 |
Population density (No. inhabitants/km2) | PD | 0.236 | <0.001 |
Population (inhabitants) | POP | 0.257 | <0.001 |
Landfill charge (CAD $/tonne) | LF | 0.065 | <0.01 |
Pay-as-you-throw | PAYT | 0.096 | <0.01 |
Bag limit | BL | 0.0145 | <1 |
Leaf and yard waste | LY | 0.211 | <0.001 |
Green bin | GB | 0.38 | <0.001 |
Infrastructure | INF (0) | 0.461 | <0.001 |
INF (1) | 0.461 | <0.001 | |
INF (2) | 0.461 | <0.001 | |
INF (3) | 0.461 | <0.001 | |
Implementation (No. years since implementation) | IMP | 0.358 | <0.001 |
Note: OFMSW, organic fraction of municipal solid waste.
Results


Regression analyses and key factors for organic waste diversion rates
Coefficient values | ||||||
---|---|---|---|---|---|---|
Dependent variable | Adjusted R2 | Model significance | Explanatory variablea | Coefficient estimate | t | p |
Europe | ||||||
OFMSW Diversion (DY2) | 0.5841 | <0.001 | Constant | −3.38 | −7.85 | <0.001 |
Income | 1.08E−4 | 5.25 | <0.001 | |||
Incineration tax | 0.78 | 2.11 | <0.05 | |||
Change in OFMSW Diversion | 0.2282 | <0.001 | Constant | −3.19 | −5.84 | <0.001 |
Income | 7.64E−5 | −5.84 | <0.01 | |||
Incineration ban | 1.40 | 1.95 | <0.1 | |||
Legislation (2) | −1.09 | −1.73 | <0.1 | |||
Ontario | ||||||
OFMSW Diversion (2013) | 0.5214 | <0.001 | Constant | −3.51 | −12.1 | <0.001 |
Population density | 5.46E−4 | 2.81 | <0.01 | |||
Pay-as-you-throw | 0.50 | 2.06 | <0.05 | |||
Infrastructure (1) | 1.11 | 3.68 | <0.01 | |||
Infrastructure (2) | 1.00 | 2.60 | <0.05 | |||
Infrastructure (3) | 2.72 | 7.65 | <0.001 |
Note: DY2, second diversion year; OFMSW, organic fraction of municipal solid waste.

The fate of P through centralized organic waste processing

Data availability and limitations
Discussion
Conclusions
Acknowledgements
References
Supplementary Material
- Download
- 966.75 KB
Information & Authors
Information
Published In
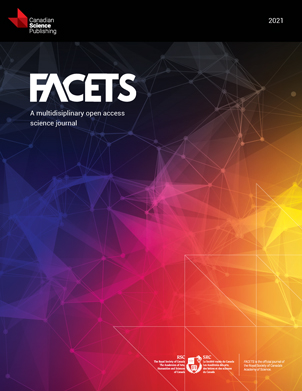
History
Copyright
Data Availability Statement
Key Words
Sections
Subjects
Authors
Author Contributions
Competing Interests
Metrics & Citations
Metrics
Other Metrics
Citations
Cite As
Export Citations
If you have the appropriate software installed, you can download article citation data to the citation manager of your choice. Simply select your manager software from the list below and click Download.