Freshwater conservation planning in the far north of Ontario, Canada: identifying priority watersheds for the conservation of fish biodiversity in an intact boreal landscape
Abstract
Freshwater ecosystems show more biodiversity loss than terrestrial or marine systems. We present a systematic conservation planning analysis in the Arctic Ocean drainage basin in Ontario, Canada, to identify key watersheds for the conservation of 30 native freshwater fish, including four focal species: lake sturgeon, lake whitefish, brook trout, and walleye. We created species distribution models for 30 native fish species and accounted for anthropogenic impacts. We used the “prioritizr” package in R to select watersheds that maximize species targets, minimize impacts, and meet area-based targets based on the Convention on Biological Diversity commitment to protect 17% of terrestrial and freshwater areas by 2020 and the proposed target to protect 30% by 2030. We found that, on average, 17.4% and 29.8% of predicted species distributions were represented for each of the 30 species in the 17% and 30% area-based solutions, respectively. The outcomes were more efficient when we prioritized for individual species, particularly brook trout, where 24% and 36% of its predicted distribution was represented in the 17% and 30% solutions, respectively. Future conservation planning should consider climate change, culturally significant species and areas, and the importance of First Nations as guardians and stewards of the land in northern Ontario.
Introduction
We are in the midst of a global biodiversity crisis. A recent comprehensive report estimated that up to a million species face extinction, many within a few decades, unless there is drastic global action to conserve species and their habitats (Díaz et al. 2019). Increasing protected areas is one approach in conservation (Dudley and Stolton 2008). However, biodiversity loss continues (Butchart et al. 2010; Vörösmarty et al. 2010; Adams et al. 2015; Díaz et al. 2019) despite a global increase in land formally recognized as protected (sensu conservation targets) since 1933 when categories of protected areas were established and since 1994 when guidelines were adopted (Dudley 2008).
Attention to conserving biodiversity through area-based targets emerged in 1988, when 3%–4% of the planet’s land area was formally protected under government- or state-recognized legislation. The Convention on Biological Diversity (CBD) signed in 1992 (cbd.int/doc/c/efb0/1f84/a892b98d2982a829962b6371/wg2020-02-03-en.pdf) recognized the importance of protected areas. In 2010, the CBD established the 20 Aichi Biodiversity Targets (cbd.int/sp/targets/), including one (Aichi Target 11) to conserve at least 17% of terrestrial and freshwater areas and 10% of coastal and marine areas by 2020. In 2015, Canada released its national targets in response, in which Canada’s Pathway to Target 1 (conservation2020canada.ca/home) commits Canada to meet the Aichi Target 11 goal (biodivcanada.chm-cbd.net/2020-biodiversity-goals-and-targets-canada). Ontario set an additional area-based target of 50% protection in the far north (Wilkinson and Schulz 2012) as part of a broader campaign to protect 50% of the Canadian boreal forest biome (e.g., the Canadian Boreal Conservation Framework). There have been calls to increase the targets following 2020, including calls for 30% by 2030 (Dinerstein et al. 2019) or 50% by 2030 (e.g., natureneedshalf.org/). In response, the Canadian government has stated that Canada will adopt an expanded area-based goal of protecting 25% of land and water by 2025, while working towards a target of 30% by 2030, and encouraging other countries to follow suit (pm.gc.ca/en/mandate-letters/2019/12/13/minister-environment-and-climate-change-mandate-letter). Although the specific targets vary and are changeable, the popularity and widespread adoption of area-based targets suggest that protected areas have an important role to play, and that targets must be based on social and ecological requirements for biodiversity.
There have been calls for decades to develop rigorous metrics to assess the management and effectiveness of protected areas to achieve conservation goals (Parrish et al. 2003; Watson et al. 2014; Adams et al. 2015; Di Minin and Toivonen 2015), with more recent research on outcomes-based metrics rather than area-based targets (Visconti et al. 2019; but see Woodley et al. 2019). Since 2004, protected area governance has included Indigenous Peoples and local communities, with an emphasis on the need for protected areas to benefit all people (Borrini-Feyerabend et al. 2013; Zurba et al. 2019). Despite these steps, protected area planning and designation is still driven by economics, and disproportionately designated in areas where land is inexpensive, rather than based on biodiversity, ecosystem services, or other social and cultural values (Venter et al. 2014, 2018).
Inland waters and freshwater ecosystems have the highest rates of biodiversity loss, and these losses have accelerated since the 1970s (Díaz et al. 2019). Consequently, freshwater species are among the most imperiled, with an 84% decline in global freshwater vertebrate populations since 1970 compared with a 40% decline for terrestrial vertebrates and a 35% decline for marine vertebrates (Díaz et al. 2019). Despite the urgent status of freshwater biodiversity, conservation planning to protect freshwater ecosystems lags behind both terrestrial and marine efforts. Freshwater ecosystems occupy less than 1% of the earth’s surface, yet contain as much as 12% of all known species and a third of all vertebrate species (Juffe-Bignoli et al. 2016). Improvements in freshwater conservation can make a disproportionately large contribution in fighting the biodiversity crisis.
Conservation planning rarely includes explicit freshwater objectives or considers the unique characteristics of freshwater systems. For example, protected areas designed for terrestrial habitats or species may not adequately address the connectivity of freshwater systems, where impacts in headwaters can affect downstream habitats located hundreds of kilometres away and where changes to water flow, through damming or water extraction, can greatly alter the characteristics and habitats in freshwater networks (Nel et al. 2009; Linke et al. 2012; Juffe-Bignoli et al. 2016). Additionally, although terrestrial protected areas may provide some benefits to freshwater species (Abraham and Kelkar 2012; Hermoso et al. 2014; Britton et al. 2017; Chu et al. 2018), they are often inadequate for protecting freshwater biodiversity (Abell et al. 2011; Hermoso et al. 2014). Globally, freshwater biodiversity benefits when the design and implementation of protected areas explicitly includes freshwater considerations (Juffe-Bignoli et al. 2016).
Systematic conservation planning uses spatial optimization to meet explicit targets while evaluating trade-offs between a set of decision variables (Margules and Pressey 2000; Wilson et al. 2009). This approach is widely accepted as an effective method to identify areas for biodiversity conservation; however, it has only recently been applied in the context of freshwater (Hermoso et al. 2016). In this study, we use conservation prioritization to evaluate scenarios that conserve freshwater fish species in the Arctic Ocean drainage basin in Ontario, Canada, while considering freshwater connectivity and avoiding areas with high human disturbance.
We focus on the Arctic Ocean drainage basin in Ontario for several reasons. This region is more water than land (Wells et al. 2010), underscoring the importance of freshwater values in conservation planning. Furthermore, the freshwater ecosystems in this region are large, mainly intact, contain some of the largest free-flowing rivers left in the world (Grill et al. 2019), and are the third-largest wetland (Abraham and Keddy 2005). Potential future development in the region includes all-weather roads, transmission corridors, mineral extraction, hydropower, and expanding forestry. Thus, this area offers an important and rare opportunity to consider proactive conservation planning before development, which is more cost-effective than retroactive action (Fuller et al. 2007).
We focus on freshwater fish biodiversity because fish are ecologically and socially important. Ecologically, there are at least 50 species of native freshwater fish in the Arctic drainage basin in Ontario, a limited number of invasive species and pathogens, and low human impacts compared with other subarctic regions of Canada (Browne 2007; Chu et al. 2014). Socially, freshwater fish are highly valued by First Nations and local communities. First Nations have long-standing relationships with freshwater fish, which are constitutionally protected and critical components of food security and food sovereignty (Noble et al. 2016) and important for livelihoods through recreational and commercial fisheries (Cooke and Murchie 2015).
Using conservation prioritization, we evaluate 12 scenarios to meet two area-based targets (17% and 30%) relevant to Canada’s biodiversity commitments to protect 17% of terrestrial land and freshwater by 2020 and 30% by 2030. Our scenarios prioritize key areas for overall native fish biodiversity, and four focal fish species: lake sturgeon (Acipenser fulvenscens), lake whitefish (Coregonus clupeaformis), brook trout (Salvelinus fontinalis), and walleye (Sander vitreus). Since there are existing provincial and federal protected areas in northern Ontario, we also explore the contribution of these existing protected areas to conserve freshwater fish biodiversity.
Focussing on the Arctic Ocean drainage basin in Ontario, we examine three questions:
1.
What watersheds are selected to meet the 17% and 30% area-based targets while maximizing species distribution probabilities for 30 native fish species (i.e., native freshwater biodiversity), compared with four focal species individually (i.e., lake sturgeon, lake whitefish, brook trout, walleye)?
2.
How well are the species distribution probabilities for the four focal species represented in the scenarios that prioritize species distribution probabilities for all 30 native fish species and vice versa?
3.
How well does the existing protected areas network in northern Ontario contribute to the conservation of freshwater biodiversity?
Methods
Study area
The 714 730 km2 Arctic Ocean drainage basin in northern Ontario, Canada, contains nine major river basins (Figs. 1a and 1b) and some of the largest naturally flowing rivers remaining in the world (Dynesius and Nillson 1994). There are thousands of lakes, particularly in the Boreal Shield ecozone (Marshall and Jones 2011), and the largest wetland complex in North America is located in the Hudson Plains ecozone (Keddy et al. 2009), which is globally one of the most productive subarctic coastal wetland habitats (Far North Science Advisory Panel 2010; Abraham et al. 2011). In contrast to the Boreal Shield, there are few large, deep lakes in the Hudson Plains (Fig. 1b).
Fig. 1.

These freshwater ecosystems support at least 50 species of freshwater fish, making it the largest area of high fish biodiversity with low human impacts in Canada (Marshall and Jones 2011; Browne 2007). Walleye and northern pike (Esox lucius) are the most common top predators in the lakes and rivers flowing through the Boreal Shield ecozone; deeper lakes have lake whitefish, lake trout (Salvelinus namaycush), and cisco (Coregonus artedi). In the Hudson Plains ecozone, brook trout are often the top predator and some of the major rivers support lake sturgeon populations.
Here, we concentrate on 30 native freshwater fish species, as a measure of overall freshwater biodiversity, and four focal species: lake sturgeon, lake whitefish, brook trout, and walleye. The four species were selected because they are ecologically and socially important in the region. Ecologically, these four species are representative of the major fish community guilds (Browne 2007) and important “freshwaterscape species” identified previously through a site-based approach integrating knowledge of the characteristics, data gaps, and vulnerabilities of each species (McDermid et al. 2015). Socially, there are important recreational fisheries for walleye and brook trout in the road-accessible regions of northern Ontario and fly-in fisheries in the remote northern parts of Ontario (i.e., in the far north). Additionally, lake sturgeon is designated a species-at-risk at the provincial, federal, and international level (Haxton and Cano 2016) and is a culturally important species for First Nations and local communities.
The study area has two different management regimes: the Area of Undertaking, defined by commercial forestry, and the far north, which includes some of the most intact remaining boreal forest in the world (Brandt et al. 2013) (Fig. 1a). The far north has few all-season roads and a small industrial footprint, with a limited number of dams and mines, and mainly remote First Nations communities. However, there are ongoing economic interests in the far north, where the Ontario government and some First Nations are contemplating development projects, such as all-season road, mineral extraction (particularly in the so-called “Ring of Fire”, Hjartarson et al. 2014), hydropower facilities (Hatch Ltd. 2013), transmission lines (OMOE 2017), and expanding commercial forestry (OMOI and OMNDMF 2011; OMNR 2012). This, combined with proposed changes to the management of the far north by the Ontario government (OMNRF 2019a), means there is uncertainty about the conservation and protection of freshwater systems in the study area.
Watershed planning units and connectivity
The hydrological gradient in the study area flows in a northeast direction, from the Area of Undertaking into the far north of Ontario (Figs. 1a and b). Since the upstream effects of any development can be direct and cumulative on downstream watersheds (Pringle 2001, 2003; Freeman et al. 2007), we included the entire Arctic Ocean drainage basin in our conservation prioritization analysis (Fig. 1a) and developed a freshwater connectivity parameter.
First, we generated watershed planning units from six 30-m hydrology-enforced enhanced flow direction grids available from the Ontario Ministry of Natural Resources and Forestry (OMNRF) Ontario Integrated Hydrological Datasets. We used a threshold of 300 km2 to create a drainage network based on the modelled overland flow accumulation resulting in 1417 stream segments and corresponding watersheds (Fig. 1b) with an average area of 504 km2 (Maidmant 2002). We completed the processing using the ArcHydro 10.5.0.13 toolset (Esri Water Resources Team) in ArcGIS 10.5 (Esri, Redlands, CA, USA).
Second, we calculated the connectivity between connected watersheds based on the methodology in Hermoso et al. (2012). The exterior boundary, or boundary length modifier (which is the connectivity parameter used for terrestrial or marine conservation planning), is not an appropriate measure of freshwater connectivity because fish can only move upstream and downstream between planning units (Hermoso et al. 2011; Linke et al. 2012). Instead we measured the river length from each watershed outlet to the furthest downstream outlet (i.e., major river outlet into Hudson Bay), and calculated the distance between each pair of connected watersheds in each river basin.
Third, we used the inverse linear distance between watersheds as the connectivity parameter in the conservation prioritization analysis to penalize the selection of watersheds when adjacent upstream or downstream watersheds are not also selected (Hermoso et al. 2012). The inverse linear distance means there is a lower penalty applied to watersheds that are further away from each other, and a higher penalty is applied for adjacent watersheds when determining which watersheds should be selected to achieve the conservation target (Hermoso et al. 2012). A connectivity strength modifier of 2 was applied to the connectivity parameter to scale the range of values to the cost parameter (i.e., anthropogenic impacts index, as described below).
Assessing native freshwater fish biodiversity
Fish are the most commonly sampled freshwater biota in Ontario (Marshall and Jones 2011), but scientific surveys are limited within the study area, particularly in the far north. We developed species distribution models (SDMs) based on publicly available species occurrence, climate, and biophysical data sets. In the conservation prioritization analysis, the outputs from the SDMs were used as species representation targets.
We compiled species occurrence records from the Royal Ontario Museum (ROM) dated from 1920 to 2014 (Mandrak and Crossman 1992) and from three OMNRF databases dated from 1958–1995 and 2007–2012 (Dodge et al. 1985; Land Information Ontario 2009; Sandstrom et al. 2010). We selected the 30 most-common, native freshwater fish species that had sufficient occurrence records to make modelling feasible (Table S1).
We used eight climate and biophysical variables as predictor variables based on their ability to distinguish between biologically distinct fish responses and geographically distinct areas at different scales (Tables 1 and S2). The climate variables were derived from the AdaptWest 1961–1990 climate normal (AdaptWest Project 2015) to align with the time frame in which the majority of fish occurrence records were collected, and the biophysical variables were derived from the Land Facets Data for North America (Michalak et al. 2018). Variable choice was informed by selecting candidate variables that are ecologically meaningful for freshwater fish, describe unique aspects of habitat suitability, and avoid collinearity (Braunisch et al. 2013; Leroy et al. 2016; Supplementary Material 1).
Table 1.
Climate Variables | Biophysical Variables |
---|---|
• Mean annual solar radiation • Mean temperature of the warmest month • Summer precipitation (June to August) • Winter precipitation (December to February) | • Elevation (30 m Ontario Ministry of Natural Resources and Forestry Ontario Integrated Hydrological Datasets digital elevation model) • Soil order • Landforms (categorized slope and topographic position index) • Land facets (with nonadjusted elevation and a modified heat load index) |
We used maximum entropy distribution modelling (Maxent; Phillips et al. 2006, 2017) to fit SDMs because it is specifically designed for use with presence-only occurrence records (Elith et al. 2011; Phillips and Elith 2013). We used Maxent v3.3.3 in R and followed the best practices outlined by Smith (2020). For all models, we used linear and quadratic features and selected the regularization parameter (beta) using Akaike’s Information Criterion based on the methods described in Warren and Seifert (2011). Otherwise, we used the default settings.
Maxent generates species distribution probabilities, with values that range from 0 (lowest probability) to 1 (highest probability) across geographic space (Elith et al. 2006). Since our species distribution models generated probabilities at a 30-m grid resolution, which is smaller than the size of the watershed planning units, we attributed the mean species distribution probability for each species to each watershed planning unit (Fig. 2). These mean species distribution probabilities were used as the species representation targets in the conservation planning analysis. We also created a “freshwater biodiversity index” based on the overall mean species distribution probability for all 30 species in each watershed to highlight areas of high and low freshwater species distributions (Fig. 1d). This index was used for visualization only, and it was not used as a parameter in the subsequent analysis.
Fig. 2.

Anthropogenic impacts index
For the cost parameter in our conservation prioritization analysis, we created an anthropogenic impacts index based on 11 spatial data sets of human disturbances known to affect freshwater fish (Table 2). The anthropogenic impacts index was developed using a principal component analysis (PCA), which is a widely adopted method for distilling multifaceted information into a minimal set of uncorrelated input variables for use in subsequent analyses (Jolliffe and Cadima 2016).
Table 2.
Input variable | Data set source |
---|---|
Number of producing minesa | GSC 2017, OGS 2017 |
Producing mines densitya | GSC 2017, OGS 2017 |
Abandoned mines densitya | GSC 2017, Ontario MNDM 2017a |
Active aggregate sites densitya | Québec MRNF 2016, Ontario MNRF 2017a |
Inactive aggregate sites densityb | Québec MRNF 2016, Ontario MNRF 2017b |
Settlement density, including First Nation communities, towns, and citiesc | Natural Resources Canada 2017 |
Population densitya | Statistics Canada 2016, CIRNAC 2017 |
Percent of planning unit covered by anthropogenic land uses (including forestry, roads, mines, transmission lines, and other industrial activities)a | Global Forest Watch Canada 2014 |
Percent of planning unit covered by mining claimsa | Québec MRNF 2016, Ontario MNDM 2017b |
Number of damsc | Wildlife Conservation Society Canada, unpublished data |
Dam densityc | Wildlife Conservation Society Canada, unpublished data |
a
Indicates variables with the most influence in the first component of the principal components analysis (loadings between 0.32 and 0.38).
b
Indicates variables with moderate influence (loading = 0.26).
c
Indicates less influential variables (loadings between 0.10 and 0.16).
Because of the limited human disturbance across much of the study area, there were many zero values in our initial data set (41% of the planning units). To reduce the impact of zero-inflation and improve the linearity of associations between variables, we used a log(x+1) transformation to standardize input variables before running the correlation based PCA (Quinn and Keough 2003). We used the “vegan” package (Oksanen et al. 2019), “permute” package (Simpson 2019), and “princomp” function in R to develop the PCA.
The first principal component from the PCA was assigned to each watershed and used as the “costs” in the conservation prioritizations (i.e., the prioritization analysis minimized the selection of watersheds with existing anthropogenic impacts) (Fig. 1c). The first axis captured 28.8% of the variation among watershed planning units, and a broken stick analysis determined it was the only meaningful component (Jackson 1993). This principal component was positively loaded with all 11 input variables (Table 2).
Existing protected areas
There are 169 existing provincial and federal protected areas covering 75 741 km2 (10.6% of the study area), summarized in the Conservation Areas Reporting and Tracking System (CARTS) (CCEA 2017) (Fig. 1a). In Ontario, these include conservation reserves, migratory bird sanctuaries, national parks and wildlife areas, provincial parks, dedicated protected areas in the far north, valued ecosystem and natural habitats, and wilderness areas. We identified protected area watersheds where greater than 50% of the watershed overlapped with an existing protected area (Fig. 1b). One-hundred and twenty watershed planning units met this threshold, covering 57 353 km2 (8% of the study area) and representing 26 protected areas (Table S3).
Conservation prioritization scenarios
Systematic conservation planning aims to maximize the representation of conservation targets, in our case species distribution probabilities, while minimizing constraints to achieve spatially optimized conservation outcomes (Margules and Pressey 2000). We used the “prioritizr” package v4.0.4 (Hanson et al. 2019) in R with the Gurobi solver (Gurobi Optimization, LLC) to solve a “minimum set problem” based on a range of species representation targets (i.e., species distribution probabilities), while minimizing the values of the two other parameters (i.e., freshwater connectivity and anthropogenic impacts, as described above). Prioritizr uses integer linear programming to solve for a true optimal solution, making it computationally more efficient than other popular packages such as Marxan, which uses simulated annealing to solve for a near-optimal solution (Beyer et al. 2016).
We examined six different scenarios to meet the 17% and 30% area-based targets, for a total of 12 conservation prioritization analyses (Table 3). We ran two biodiversity scenarios: (i) with no additional constraints (unseeded) and (ii) with the existing protected area watersheds “locked in” to the solution (seeded). The biodiversity scenarios maximized species representation targets for all 30 species simultaneously. We also ran unseeded scenarios for each of the four focal species: lake sturgeon, lake whitefish, brook trout, and walleye (Table 3).
Table 3.
Conservation target | ||
---|---|---|
Scenario | 17% area-based target | 30% area-based target |
Biodiversity | ||
30 species | Unseeded | Unseeded |
30 species | Seeded | Seeded |
Focal species scenario | ||
Lake Sturgeon | Unseeded | Unseeded |
Lake Whitefish | Unseeded | Unseeded |
Brook Trout | Unseeded | Unseeded |
Walleye | Unseeded | Unseeded |
In all scenarios, we first solved the minimum set problem for a range of species representation targets, from 10% to 90%, using an interval step of 10%. We used these results to determine the interval that straddled the 17% and 30% area-based conservation targets; and reran each scenario using a smaller interval step of 1% to find the optimal solution to meet each area-based target.
Results
We separate the results into three main findings based on our research questions. First, we identify the key areas for conservation of biodiversity and focal species; second, we discuss the performance of our models to maximize freshwater species representation targets under different scenarios; and third, we look at the conservation value of including the existing protected areas in the analysis.
Key areas for freshwater fish biodiversity conservation
In general, headwater planning units, coastal planning units, tributaries to large rivers, and watersheds with no upstream human disturbance were the most important areas in our solutions. Forty-seven watersheds were consistently selected in the solutions at the 17% area-based target, covering 3.7% of the study area; likewise, there were 190 watersheds consistently selected in the solutions at the 30% area-based target, covering 13.9% of the study area (Figs. 3a and 3b).
Fig. 3.

Importantly, the Kapiskau River watershed (located between the Attawapiskat and Albany River basins) and the southern tributaries to the lower Albany River were selected in all solutions. Furthermore, the biodiversity solutions consistently selected tributaries to the Severn River, sections of the Nelson River basin along the Ontario–Manitoba border, and watersheds at the headwater intersection of the Nelson, Severn, Winisk, and Albany River basin (Figs. 3c and 3d).
In the focal species solutions, there was a higher selection of watersheds in the Ekwan River basin, with these watersheds selected at the 17% area-based target for brook trout and at the 30% area-based target for lake sturgeon, lake whitefish, and walleye (Fig. 4). Watersheds in the Ekwan basin were not selected as extensively in the unseeded biodiversity solutions; however, they were selected in the seeded biodiversity solutions when they were “locked in” because of the inclusion of Polar Bear Provincial Park (Figs. 3c and 3d).
Fig. 4.

Freshwater species representation in different scenarios
Each biodiversity solution included a measure of the species representation for each the four focal species, and some species performed better or worse when evaluated independently. When lake sturgeon and brook trout were independently prioritized, they had higher species distribution probabilities represented relative to the biodiversity solutions (Table 4). Brook trout, a coldwater specialist, had the highest species distribution probability represented at the 17% and 30% area-based targets, with 24% and 36% of its predicted distribution represented in the brook trout solutions, respectively (Table 4). It also showed the most divergent results from the other focal species, with a greater proportion of coastal watersheds along Hudson Bay selected at the 17% area-based target in comparison with other species. Lake whitefish and walleye (habitat generalists) had the lowest species distribution probabilities represented in the individual species solutions, with probability values that were equal to, or lower than, the values represented in the biodiversity solutions (Table 4).
Table 4.
17% area-based target | 30% area-based target | |||||
---|---|---|---|---|---|---|
Biodiversity scenario | Focal species scenario | Biodiversity scenario | Focal species scenario | |||
Species | Unseeded (%) | Seeded (%) | Unseeded (%) | Unseeded (%) | Seeded (%) | Unseeded (%) |
30 species (mean) | 17.4 | 16.8 | N/A | 29.8 | 29.3 | N/A |
Lake Sturgeon | 17.4 | 16.0 | 19.0 | 29.9 | 28.9 | 28.0 |
Lake Whitefish | 17.6 | 17.0 | 17.0 | 30.3 | 29.5 | 26.0 |
Brook Trout | 16.0 | 17.0 | 24.0 | 27.0 | 27.0 | 36.0 |
Walleye | 17.9 | 17.2 | 17.0 | 30.6 | 29.9 | 27.0 |
Note
The mean value of the species distribution probability of all 30 species is reported for the biodiversity scenarios, and the individual values for the four focal species are reported in all scenarios. The unseeded scenarios had no constraints, and the seeded scenarios had the existing protected area watersheds locked in to the solutions. N/A, not applicable.
At both target levels, there was a higher mean species distribution probability represented for all 30 species in the unseeded biodiversity solutions compared with the seeded biodiversity solutions (17.4% vs. 16.8% at the 17% target; 29.8% vs. 29.3% at the 30% target) (Table 4). This relationship holds for lake sturgeon, lake whitefish, and walleye when looking at their individual species distribution probabilities represented in the biodiversity solutions (Table 4). However, brook trout showed the opposite trend where it had a higher species distribution probability represented in the seeded biodiversity solution (17.0%) relative to the unseeded solution (16.0%) at the 17% area-based target and no difference at the 30% area-based target (Table 4).
Conservation value of existing protected areas for freshwater fish biodiversity
A larger percentage of the existing protected area watersheds were selected in the unseeded biodiversity solution compared to the focal species solutions, with 38.3% and 58.6% of the protected area watersheds selected in the biodiversity solution at the 17% and 30% area-based targets, respectively (Fig. 3c). The smallest percentage of existing protected area watersheds were selected in the lake sturgeon solutions at both target levels, with only 14.3% and 47.0% selected in the solutions at the 17% and 30% area-based targets, respectively (Fig. 4c). At the 17% area-based target, the percent of existing protected area watersheds selected in the other three focal species solutions were 23.4% for lake whitefish, 24.6% for walleye, and 31.6% for brook trout. At the 30% area-based target, these values increased to 49.3% for brook trout, and 55.4% for lake whitefish and walleye (Figs. 4a, 4b, and 4d). Brook trout had the smallest increase (17.6%), while lake sturgeon had the largest increase (32.7%) in the percent of protected area watersheds selected at the two area-based targets.
A subset of the 49 watersheds overlapping Polar Bear Provincial Park in the Ekwan River, Winisk River, and Hudson Bay Coast basins was selected in all unseeded solutions, with the percentage of selected area ranging from 4.2% (for walleye) to 92.4% (for brook trout). The brook trout solutions had the largest selection of this protected area at both target levels, with 74.0% and 92.4% of the watersheds in Polar Bear Provincial Park selected at the 17% and 30% area-based targets, respectively (Fig. 4c). At the 30% area-based target, the selection of watersheds in Polar Bear Provincial Park was 20% lower in the unseeded biodiversity solution than in the focal species solutions.
Wabakimi Provincial Park (Albany River basin) was important in the unseeded biodiversity solutions, with 80.3% of the watersheds in Wabakimi Provincial Park selected at the 30% area-based target (Fig. 3c); however, it was less important for the four focal species. Specifically, there were no watersheds selected in Wabakimi for the brook trout and lake sturgeon solutions at the 17% area-based target, and only 4.0% and 4.9% of the watersheds in Wabakimi were selected for the brook trout and lake sturgeon solutions at the 30% area-based target (Fig. 4).
The Jog Lake Conservation Reserve (Albany River basin) and the North of the North French River Conservation Reserve (Moose River basin) were selected in all solutions at both area-based targets. Additionally, the Hannah Bay Migratory Bird Sanctuary, located on the Hudson Bay coast along the Ontario–Québec border, was selected in all solutions, except in the unseeded biodiversity solution at the 17% area-based target.
Other existing protected areas with moderate to high selection in all solutions, except brook trout, at the 30% area-based target included the protected areas along the Manitoba–Ontario border: Asatiwisipe Aki Traditional Use Planning Area, Atikaki Provincial Park, Little Grand Rapids Dedicated Protected Area, Weeskayjahk Ohtahzhoganeeng Protected Area, and Woodland Caribou Provincial Park. Combined, these protected areas are part of the Pimachiowin Aki UNESCO World Heritage site which extends over the Poplar, Berens, Pigeon, and Bloodvein rivers and is the first “mixed” World Heritage site in Canada (UNESCO 2018).
The majority of the watersheds in the Cat Lake – Slate Falls Dedicated Protected Area were selected in most solutions, but were not selected at the 17% area-based target for lake sturgeon. This area is important for freshwater conservation because it spans the intersection of the headwaters for the Severn, Winisk, Albany, and Nelson River basins. Of note, the watershed planning units in and around this area were selected in the unseeded freshwater fish biodiversity solutions to meet both area-based targets, indicating that they are of high conservation value even without being explicitly locked into the solution.
Discussion
Performance of conservation prioritization scenarios based on area-based targets
We found that the conservation prioritizations performed reasonably well in representing the predicted species distributions for all 30 species, and for key focal species when meeting the existing and aspirational federal area-based targets of 17% and 30% (Table 4). However, the conservation prioritizations were most effective in the individual brook trout solutions. Brook trout are considered an indicator of high-quality, least-disturbed fish habitat and require specialized habitats including clear, cool, well-oxygenated streams and lakes and groundwater upwellings for spawning (Haxton et al. 2020). Relative to other species in our study area, these habitats may correspond to areas with lower human disturbance (e.g., Ekwan River basin).
Overall, the southern headwaters in our study area have both higher levels of human disturbance (Fig. 1c), and higher freshwater fish biodiversity (Fig. 1d). Although a few specialist species occur along the coast of Hudson Bay (e.g., sea-run brook trout; Fig. 2c), for the most part, higher species distribution probabilities for the 30 native freshwater fish species occur more often in the southern, lake-dominated landscape of the Boreal Shield relative to the northern, wetland-dominated landscape of the Hudson Plains (Fig. 1d). This pattern at the regional scale reflects a broader global pattern where ecosystems supporting higher levels of biodiversity are also subject to higher human demand (Weinzettel et al. 2018). Despite the high species richness in the southern headwaters, watersheds in this region were only selected in the biodiversity solutions. We speculate that the lack of selection of the southern headwaters in the focal species solutions is due to the higher anthropogenic impacts in these watershed planning units, which outweighs the individual species representations, but there may be other factors that we have not considered or were not captured in our analysis.
The watersheds consistently selected in our analyses represent areas of relatively low human disturbance with few upstream impacts, moderate to high species distributions, or both. The results of the study support the use of a systematic conservation planning approach to identify watersheds where the costs associated with human disturbances are lower and freshwater fish biodiversity is maximized (Kukkala and Moilanen 2013).
Effectiveness of freshwater fish biodiversity solutions for the protection of focal species and vice versa
We found that there was overlap between the watershed planning units selected for freshwater fish biodiversity and for the focal species. However, there were some exceptions, particularly for brook trout. Brook trout is the most specialized focal species we examined, as indicated by their unique distribution patterns (Fig. 2). This result reinforces the need for unique habitat protections for specialists, since they are less likely to be adequately protected under approaches that optimize solutions for multiple species. Further, we note that while all our focal species have large ranges within our study area, area-based targets may not be meaningful for range-restricted focal species, and they may require different considerations.
Conversely, freshwater fish biodiversity protections may be more useful for generalist species. Our results suggest that by attempting to meet the needs of a diverse community of fishes, there is a risk of limiting the selection of specialized areas for a given focal species. This is a commonly observed trade-off in conservation planning, particularly when specialist species are also spatially rare or found in very low abundance (Hamaide et al. 2006). It is possible that optimizing for different elements of biodiversity, such as functional phylogenetics, could improve the achieved representation of niche space for different life history groups or species guilds (Strecker et al. 2011), and this should be a topic of further research.
While conservation strategies that maximize biodiversity outcomes are a pressing and prevalent objective for protected area planning, freshwater fish conservationists will continue to face the challenges of having to include approaches that also protect rare, endemic, and endangered species (Hamaide et al. 2006; Sowa et al. 2007). There is also a long history of focal species planning in commercial and recreational fisheries management, including government regulatory systems focussed on species at risk (Fisheries Act 1985; SARA 2002). Conservation planning has also focussed on “umbrella species” under the premise that because of their ecological requirements, their protection will have meaningful benefits for other fishes and communities (McDermid et al. 2015). Assessment of this assumption has been equivocal (Simberloff 1998; Roberge and Angelstam 2004).
However, area-based targets for biodiversity, in combination with special consideration for rare, endemic, and endangered species, have the potential to provide an effective approach. Lake sturgeon is a cultural keystone species and a species at risk, and key habitat for this species must be considered in any watershed or regional conservation planning process. Our solutions indicate that there was intermediate congruence between the lake sturgeon solutions and the biodiversity solutions as compared to the other focal species. The largest gaps in the lake sturgeon solutions (i.e., from the perspective of using lake sturgeon as an “umbrella” to conserve overall freshwater fish biodiversity) were in the Ekwan River basin, upper Albany River basin, and northwest portion of the Nelson River basin. Our results provide some support for including existing protected areas, in combination with targeting new protections around priority areas for lake sturgeon, to build an effective regional conservation plan for both this threatened species and overall fish biodiversity.
Effectiveness of existing protected areas to support freshwater fish biodiversity
The existing protected areas in the study area were designated through a variety of land use planning processes based primarily on ecological, geological, and other cultural heritage features (OMNRF 2019b). More recently, community-based land use planning with some First Nations in the far north have prioritized freshwater fishes, their habitat, and freshwater connectivity (e.g., in Dedicated Protected Areas). The results from our conservation prioritization approach can provide support to future planning processes and offer opportunities to consider other areas to conserve freshwater fish biodiversity when evaluating new protection designations or development projects.
When examining all 30 species, “locking in” the existing protected area watersheds produced similar results at the 17% and 30% area-based targets relative to allowing the analysis to optimally select from any watershed in the study area. However, the representation of species distribution probabilities for lake sturgeon, lake whitefish and walleye were higher when the conservation prioritization was not constrained, suggesting that there are additional watersheds not captured in the existing protected areas network where the conservation outcomes for these focal species could be increased.
Large portions of Wabakimi Provincial Park were selected in the freshwater biodiversity solutions (Figs. 3c and 3d), but not in the focal species solutions (Fig. 4). Wabakimi is in the southern headwaters, where there is a higher overall mean freshwater fish distribution (Fig. 1d) and higher anthropogenic impacts outside the protected area (Figs. 1b and 1c). This result demonstrates that in areas with more human disturbance, protected areas can be critical for preserving the remaining areas with lower human disturbance that support freshwater fish biodiversity (Fischer and Lindenmayer 2007).
Conversely, in the more intact northern parts of our study area, protected areas are not necessarily more intact than the rest of the landscape in which they are embedded (e.g., the matrix). In the absence of human disturbance and competing demands for land and water use, there remains an important opportunity to proactively prioritize areas for protection based on both high freshwater fish biodiversity, as well as focal species of ecological and social value.
Interestingly, the coastal rivers in the Ekwan River basin, which make up a large part of the eastern side of Polar Bear Provincial Park near Cape Henrietta Maria, were not heavily selected in the biodiversity solutions, but were extensively selected in the brook trout solutions at the 17% area-based target and in the lake sturgeon, lake whitefish, and walleye solutions at the 30% area-based target. Although this area does not have high biodiversity overall, it is intact and has important habitat for sea-run brook trout and moderate habitat for our other focal species (Far North Science Advisory Panel 2010). Thus, while the Ekwan River basin is not the highest priority for overall freshwater fish biodiversity, it is a key area for socially and ecologically important freshwater fish species and a potential climate refugia as southern waters begin to warm (Chetkiewicz et al. 2017).
Limitations
This study shows that protected area planning in an intact landscape with area-based targets has promise for identifying areas for further consideration in protecting freshwater fish biodiversity and key freshwater fish species. However, there are limitations to our approach.
First, species occurrence information in this landscape was limited, requiring that conservation planning be based on predicted species distribution probabilities for the freshwater fish species. Knowledge scarcity across remote northern regions in Canada has been previously identified as a threat to effective freshwater conservation now and in the future (Chu et al. 2003; Alofs et al. 2014; McDermid et al. 2015; Edwards et al. 2016). Our species distribution models, based on biased and infrequently sampled occurrence records, may not have captured aspects of fish life history or temporally discrete habitat needs that are important for actual on-the-ground protection. The prioritization assessments could also have missed key habitats at finer spatial scales, which could have significant consequences for representativeness and effectiveness in the solutions. For example, lake sturgeon, which is a migratory species, needs big intact rivers and access to a variety of micro-habitats at different times and frequencies (Lacho 2013).
Second, we examined only a small part of overall biodiversity by focussing on freshwater fish. From an ecosystem function perspective, conservation action should ideally maximize multi-faceted diversity (including genetic diversity, trophic diversity, etc.), both within and across clades of taxa (Strecker et al. 2011). Further, consideration of freshwater conservation should not stop at the biodiversity within water itself. Land–water linkages are an important determinant of freshwater ecosystem integrity and productivity (Tanentzap et al. 2014), and protected areas achieve the best results for overall biodiversity when both freshwater and terrestrial priorities are simultaneously considered (Amis et al. 2009).
Third, watershed planning units were only considered as part of the existing protected areas network when >50% of their area overlapped with a designated protected area, but there are a number of “waterway class” provincial parks in the study area where only the mainstem river and a small buffer is protected (Fig. 1a). These protected areas, ostensibly designed for freshwater activities, were not picked up or considered as “protected” in this analysis, because only a small proportion of the watershed planning unit is protected relative to the entire watershed. In this freshwater-dominated region, with extensive hydrological connectivity, it is not sufficient to protect only the large, open waterbodies or mainstem rivers. Impacts to smaller tributaries, wetlands, and groundwater, as well as terrestrial environments, accumulate and concentrate in downstream lakes and rivers. As such, planning for freshwater conservation and protection is only effective when accomplished at the watershed scale (Pringle 2001; Linke et al 2011).
Fourth, the assessment of protected areas was limited to publicly available and state-recognized protected areas. Areas protected by First Nations in their homelands are not publicly available as spatial boundaries, yet a number of First Nations in the study area have declared Indigenous Protected and Conserved Areas (IPCAs) within their traditional homelands (e.g., Zurba et al. 2019). For example, Kitchenuhmaykoosib Inninuwug (KI) First Nation is establishing an Indigenous Protected Area in the Fawn River Watershed (KI Homeland) that also supports the KI Water Declaration to protect a 13 025 km2 area of boreal lakes, rivers, forest, and wetlands, including 661 km2 Kitchenuhmaykoosib Aaki (Big Trout Lake), from any uses that harm their relationships with the land and water. Similarly, Moose Cree First Nation declared that the Kah-pana-yow Sîpi or Meh-ko-pwa-meh-ŝtik Sîpi (the North French River) watershed within their traditional homeland is protected from development. IPCAs are lands and waters where Indigenous communities have the primary role in protecting and conserving ecosystems through Indigenous laws, governance, and knowledge systems and they are increasingly recognized by the federal government and some provincial and territorial governments as an important pathway for meeting area-based targets and commitments to protection (e.g., ICE 2018). However, these declarations are not recognized by Ontario or Canada and are therefore not included within the CARTS database. In addition, many of the protected areas identified within CARTS were created without consultation and consent of First Nations, especially those established before 1982, and remain areas of contention in terms of conservation planning (ICE 2018). Consequently, our assessment of “protected areas” remains biased at this stage. Further, it remains unclear how dedicated protected areas identified by some communities in the far north of Ontario will be recognized and implemented moving forward as the provincial government reviews the Far North Act, 2010 (OMNRF 2019a).
Recommendations for implementation and further research
Regardless of how comprehensively biodiversity is considered, conservation planning must also consider cultural significance and the importance of Indigenous and local community stewardship. Planning for freshwater conservation is better positioned to succeed if it considers both the needs of biodiversity and the benefits of biodiversity to humans (sensu freshwater ecosystem services; Hermoso et al. 2016). In northern Ontario, this includes recognition and support for IPCAs and co-management of the land and water with First Nations communities. Protected area planning and management in northern Ontario must include First Nations communities who maintain relationships to the land and water, have inherent Treaty and Aboriginal rights, and through their stewardship will deliver positive conservation outcomes for provisioning and other ecosystem services that extend beyond Ontario to Canada and the world (Bennett et al. 2018, Far North Science Advisory Panel 2010). There are a growing number of examples where co-governance systems for freshwater and marine protected areas are generating positive social and ecological outcomes (Gelcich et al. 2018; Plotkin 2018). Area-based freshwater conservation approaches may be a useful tool in planning by including social, cultural, and governance factors.
With area-based conservation planning, it is important to set specific and achievable targets for protection. We considered 17% and 30% of the Arctic Ocean drainage basin in Ontario based on existing and aspirational Canadian targets (CBD 2010; Dinerstein et al. 2019). In reality, protection tends to be disproportionately high in remote areas (Pimm et al. 2018), so for Canada to achieve their targets, they will likely rely on remote northern areas like those examined in this analysis, compared with more developed southern areas where competing demands are already high. The approach developed in this paper will allow for the expansion of area-based targets according to Canada’s and Ontario’s commitments and an opportunity to support First Nations rights and responsibilities across their homelands.
Climate change has the potential to be a dominant factor in driving the conservation outcomes of freshwater systems and their biota (Keller 2007; Schindler and Lee 2010), and it is predicted to significantly alter the diversity and distributions of freshwater fish, particularly in northern regions (Chu et al. 2005; Edwards et al. 2016). Accordingly, conservation planning needs to consider climate change trajectories and their implications for protected area effectiveness and longevity (Felton et al. 2009; Pettorelli 2012). While we did not examine future climate predictions and their impacts on freshwater biodiversity, this research can form the basis upon which to develop approaches to integrate the projected shifts in species distributions over time to produce adaptive freshwater conservation plans (Garcia-Moreno et al. 2014) that consider the protection of key watersheds for the maintenance of habitat and connectivity long-term.
As systematic conservation planning continues to advance and form the basis for providing biodiversity protection, evaluation of implementation success and effectiveness will be critical. There is a lack of published information on the success of systematic conservation plans (McIntosh et al. 2018), including those with freshwater conservation goals (Adams et al. 2015; Hermoso et al. 2016). In the few instances where protected area performance has been assessed for freshwater biota, evidence of positive results has been inconsistent (Adams et al. 2015). A carefully designed monitoring and assessment scheme must be implemented for protected areas established for freshwater biodiversity, so that effectiveness can be evaluated and adaptive management can be employed where necessary. This must entail metrics measured over the long-term that can discern between design and management shortcomings (Hermoso et al. 2016) and appropriately account for natural ranges of variability, baseline shifts, and time lags (Adams et al. 2015). In northern Ontario, the development of community-based monitoring and Guardians programs also offers important opportunities to address First Nations governance in the research, monitoring, and conservation of freshwater fish.
In summary, systematic conservation planning for freshwater fish shows promise in an intact region of northern Ontario. Spatially optimized solutions to meet freshwater biodiversity and focal species representation targets can support efforts to deliver on area-based conservation targets, while also supporting Indigenous-led conservation and protection efforts and community-based approaches. But like with so many things, implementation will be key. There are many challenges to effective conservation outcomes for freshwater biota in the Arctic Ocean drainage basin, from competing land-use demands to mounting climate change vulnerabilities. However, with systematic conservation planning tools at the core, this largely intact and lived-in landscape presents a unique opportunity for implementation of proactive protection for freshwater ecosystems and services.
Acknowledgements
We wish to thank Drs. Virgilio Hermoso and Stephanie Januchowski-Hartley for teaching us the fundamentals of systematic conservation planning for freshwater ecosystems and for their original 2015 report that ultimately led to this paper. We thank Adam Smith for his helpful workshop on the use of Maxent at the North American Congress for Conservation Biology in 2016. We further thank Matt Strimas-Mackey for early advice on the implementation of prioritizr and Gurobi as our optimization solver. This work was funded by the generosity and ongoing support of the W. Garfield Weston Foundation.
References
Abell R, Thieme M, Ricketts TH, Olwero N, Ng R, Petry P, et al. 2011. Concordance of freshwater and terrestrial biodiversity. Conservation Letters, 4: 127–136.
Abraham KF, and Keddy CJ. 2005. The Hudson Bay Lowland. In The world’s largest wetlands. Edited by LH Fraser and PA Keddy. pp. 118–148.
Abraham KF, McKinnon LM, Jumean Z, Tully SM, Walton LR, and Stewart HM. 2011. Hudson plains ecozone: status and trends assessment. Canadian biodiversity: ecosystem status and trends 2010. Canadian Council of Resource Ministers, Ottawa, Canada, Technical Ecozone Report, 445 pp.
Abraham RK, and Kelkar N. 2012. Do terrestrial protected areas conserve freshwater fish diversity? Results from the Western Ghats of India. Oryx, 46(4): 544–553.
Adams VM, Setterfield SA, Douglas MM, Kennard MJ, and Ferdinands K. 2015. Measuring benefits of protected area management: trends across realms and research gaps for freshwater systems. Philosophical Transactions, 370: 20140274.
AdaptWest Project. 2015. Gridded current and projected climate data for North America at 1 km resolution, interpolated using the ClimateNA v5.10 software (T. Wang et al., 2015) [data set]: Available from adaptwest.databasin.org/pages/adaptwest-climatena.
Alofs KM, Liverpool EA, Taphorn DC, Bernard CR, and Lopez-Fernandez H. 2014. Mind the (information) gap: the importance of exploration and discovery for assessing conservation priorities for freshwater fish. Diversity and Distributions, 20: 107–113.
Amis MA, Rouget M, Lotter M, and Day J. 2009. Integrating freshwater and terrestrial priorities in conservation planning. Biological Conservation, 142: 2217–2226.
Bennett NJ, Whitty TS, Finkbeiner E, Pittman, J, Bassett H, Gelcich S, et al. 2018. Environmental stewardship: a conceptual review and analytical framework. Environmental Management, 61: 597–614.
Beyer HL, Dujardin Y, Watts ME, and Possingham HP. 2016. Solving conservation planning problems with integer linear programming. Ecological Modelling, 328: 14–22.
Borrini-Feyerabend G, Dudley N, Jaeger TBL, Pathak Broome N, Phillips A, and Sandwith T. 2013. Governance of protected areas: from understanding to action. Best Practice Protected Area Guidelines Series No. 20. IUCN, Gland, Switzerland, xvi + 124pp. Retrieved from: iucn.org/content/governance-protected-areas-understanding-action-0
Butchart SHM, Walpole M, Collen B, van Strien A, Scharlemann, JPW, Almond REA, et al. 2010. Global biodiversity: indicators of recent declines. Science, 328(5982): 1164–1168.
Brandt JP, Flannigan MD, Maynard DG, Thompson ID, and Volney WJA. 2013. An introduction to Canada’s boreal zone: ecosystem processes, health, sustainability, and environmental issues. Environmental Reviews, 21: 207–226.
Braunisch V, Coppes J, Arlettaz R, Suchant R, Schmid H, and Bollmann K. 2013. Selecting from correlated climate variables: a major source of uncertainty for predicting species distributions under climate change. Ecography, 36: 971–983.
Britton AW, Day JJ, Doble CJ, Ngatunga BP, Kemp KM, and Carbone C. 2017. Terrestrial-focused protected areas are effective for conservation of freshwater fish diversity in Lake Tanganyika. Biological Conservation, 212: 120–129.
Browne DR. 2007. Freshwater fish in Ontario’s boreal: Status, conservation and potential impacts of development. Wildlife Conservation Society Canada Conservation Report No. 2. 100 p.
Canadian Council on Ecological Areas (CCEA). 2017. Conservation areas reporting and tracking system (CARTS). v. 2017.12.31 [data set]: Available from ccea.org/download-carts-data/.
Chetkiewicz C, Carlson M, O’Connor C, Edwards B, Southee M, and Sullivan M. 2017. Assessing the potential cumulative impacts of land use and climate change on freshwater fish in northern Ontario. Wildlife Conservation Society Canada Conservation Report No. 11. 138 p.
Chu C, Minns CK, and Mandrak NE. 2003. Comparative regional assessment of factors impacting freshwater fish biodiversity in Canada. Canadian Journal of Fisheries and Aquatic Sciences, 60: 624–634.
Chu C, Mandrak NE, and Minns CK. 2005. Potential impacts of climate change on the distributions of several common and rare freshwater fishes in Canada. Diversity and Distributions, 11: 299–310.
Chu C, Minns CK, Lester NP, Mandrak NE, and Rosenfeld J. 2014. An updated assessment of human activities, the environment, and freshwater fish biodiversity in Canada. Canadian Journal of Fisheries and Aquatic Sciences, 72: 1–14.
Chu C, Ellis L, and de Kerckhove DT. 2018. Effectiveness of terrestrial protected areas for conservation of lake fish communities. Conservation Biology, 32(3): 607–618.
Convention on Biological Diversity (CBD). 2010. COP-10 decision X/2. Secretariat of the Convention on Biological Diversity, Nagoya.
Cooke SJ, and Murchie KJ. 2015. Status of aboriginal, commercial and recreational inland fisheries in North America: past, present and future. Fisheries Management and Ecology, 22: 1–13.
Crown-Indigenous Relations and Northern Affairs Canada (CIRNAC). 2017. First Nation Profiles [data set]: Available from fnp-ppn.aadnc-aandc.gc.ca/fnp/Main/index.aspx?lang=eng.
Di Minin E, and Toivonen T. 2015. Global protected area expansion: creating more than paper parks. BioScience, 65(7): 637–638.
Díaz S, Settele J, Brondízio E, Ngo HT, Guèze M, Agard J, et al. 2019. Summary for policymakers of the global assessment report on biodiversity and ecosystem services of the Intergovernmental Science-Policy Platform on Biodiversity and Ecosystem Services. Plenary of the Intergovernmental Science-Policy Platform on Biodiversity and Ecosystem Services, Seventh session, Paris, 29 April–4 May 2019. 39 p.
Dinerstein E, Vynne C, Sala E, Joshi AR, Fernando S, Lovejoy TE, et al. 2019. A global deal for nature: guiding principles, milestones, and targets. Science Advances, 5(4): p.eaaw2869.
Dodge DP, Goodchild GA, MacRitchie I, Tilt JC, and Waldriff DG. 1985. Manual of instructions: aquatic habitat inventory surveys. Policy FI.2.03.01. Ontario Ministry of Natural Resources, Fisheries Branch. 172 p.
Dudley N (Editor). 2008. Guidelines for applying protected area management categories. In IUCN WCPA best practice guidance on recognising protected areas and assigning management categories and governance types by Sue Stolton, Peter Shadie and Nigel Dudley, Best Practice Protected Area Guidelines Series No. 21, IUCN, Gland, Switzerland. x + 86pp.
Dudley N, and Stolton S (Editors). 2008. Defining protected areas: an international conference in Almeria, Spain. IUCN, Gland, Switzerland. 220 pp.
Dynesius M, and Nillson C. 1994. Fragmentation and flow regulation of river systems in the northern third of the world. Science, 266(5186): 753–762.
Edwards BA, Southee FM, and McDermid JL. 2016. Using climate and a minimum set of local characteristics to predict the future distributions of freshwater fish in Ontario, Canada, at the lake-scale. Global Ecology and Conservation, 8: 71–84.
Elith J, Graham CH, Anderson P, Dudík R, Ferrier M, Guisan A, et al. 2006. Novel methods improve prediction of species’ distributions from occurrence data. Ecography, 29(2): 129–151.
Elith J, Phillips SJ, Hastie T, Dud M, Chee YE, and Yates CJ. 2011. A statistical explanation of MaxEnt for ecologists. Diversity and Distributions, 17(1): 43–57.
Far North Science Advisory Panel. 2010. Science for a changing far north. The report of the far north science advisory panel. A report submitted to the Ontario Ministry of Natural Resources. 197 p.
Felton A, Fischer J, Lindenmayer DB, Montague-Drake R, Lowe AR, Saunders D, et al. 2009. Climate change, conservation and management: an assessment of the peer-reviewed scientific journal literature. Biodiversity and Conservation, 18(8): 2243–2253.
Fischer J, and Lindenmayer DB. 2007. Landscape modification and habitat fragmentation: a synthesis. Global Ecology and Biogeography, 16: 265–280.
Fisheries Act (R.S.C. 1985, c. F-14). An Act respecting fisheries [online]: Available from laws-lois.justice.gc.ca/eng/acts/f-14.
Freeman MC, Pringle CM, and Jackson CR. 2007. Hydrologic connectivity and the contribution of stream headwaters to ecological integrity at regional scales. Journal of the American Water Resources Association, 43(1): 5–14.
Fuller T, Sánchez-Cordero V, Illoldi-Rangel P, Linajb M, and Sarkar S. 2007. The cost of postponing biodiversity conservation in Mexico. Biological Conservation, 134: 593–600.
Garcia-Moreno J, Harrison IJ, Dudgeon D, Clausnitzer V, Darwall W, Farrel T, et al. 2014. Sustaining freshwater biodiversity in the anthropocene. In The global water system in the anthropocene. Edited by A Bhaduri, J Bogardi, J Leentvaar, and S Marx. pp. 247–270.
Gelcich S, Martínez-Harms MJ, Tapia-Lewin S, Vasquez-Lavin F, and Ruano-Chamorro C. 2018. Comanagement of small-scale fisheries and ecosystem services. Conservation Letters, 12(2): e12637.
Geological Survey of Canada (GSC). 2017. “A” Series Map 900A, (ed. 66). Lands and Minerals Sector, National Energy Board.
Global Forest Watch Canada. 2014. Human access of Canada’s landscape [data set]: Available from databasin.org/datasets/0c54d369b225471ea7e9f7999ce94cc0.
Grill G, Lehner B, Thieme M, Geene B, Tickner D, Antonelli F, et al. 2019. Mapping the world’s free-flowing rivers. Nature, 569: 215–221.
Hanson JO, Schuster R, Morrell M, Strimas-Mackey M, Watts ME, Arcese P, et al. 2019. prioritizr: Systematic Conservation Prioritization in R. R package version 4.0.4 [software package]: Available from github.com/prioritizr/prioritizr.
Hamaide B, ReVelle CS, and Malcolm SA. 2006. Biological reserves, rare species and the tradeoff between species abundance and species diversity. Ecological Economics, 56(4): 570–583.
Hatch Ltd. 2013. Northern hydro assessment waterpower potential in the Far North of Ontario. Commissioned by Ontario Waterpower Association, financial support from the Ontario Government. H345182-0000-00-124-0002, Rev. 3. 5 p.
Haxton TJ, and Cano TM. 2016. A global perspective of fragmentation on a declining taxon—the sturgeon (Acipenseriformes). Endangered Species Research, 31: 203–210.
Haxton T, Ball H, and Armstrong K. 2020. Expert opinion on the status and stressors of brook trout, Salvelinus fontinalis, in Ontario. Fisheries Management and Ecology, 27(2): 111–122.
Hermoso V, Linke S, Prenda J, and Possingham HP. 2011. Addressing longitudinal connectivity in the systematic conservation planning of fresh waters. Freshwater Biology, 56: 57–70.
Hermoso V, Kennard MJ, and Linke S. 2012. Integrating multidirectional connectivity requirements in systematic conservation planning for freshwater systems. Diversity and Distributions. 18: 448–458.
Hermoso V, Filipe AF, Segurado P, and Beja P. 2014. Effectiveness of a large reserve network in protecting freshwater biodiversity: a test for the Iberian Peninsula. Freshwater Biology, 60(4): 698–710.
Hermoso V, Abell R, Linke S, and Boon P. 2016. The role of protected areas for freshwater biodiversity conservation: challenges and opportunities in a rapidly changing world. Aquatic Conservation, 26(S1): 3–11.
Hjartarson J, McGuinty L, Boutilier S, and Majernikova E. 2014. Beneath the surface: Uncovering the economic potential of Ontario’s Ring of Fire. Ontario Chamber of Commerce. 35 p.
Indigenous Circle of Experts (ICE). 2018. We rise together. Retrieved from: bit.ly/2GVU0TU.
Jackson DA. 1993. Stopping rules in principal components analysis: a comparison of heuristical and statistical approaches. Ecology, 74(8): 2204–2214.
Jolliffe IT, and Cadima J. 2016. Principal component analysis: a review and recent developments. Philosophical Transactions of the Royal Society A, 374: 2015.0202.
Juffe-Bignoli D, Harrison I, Butchart SHM, Flitcroft R, Hermoso V, Jonas H, et al. 2016. Achieving Aichi Biodiversity Target 11 to improve the performance of protected areas and conserve freshwater biodiversity. Aquatic Conservation: Marine and Freshwater Ecosystems, 26(Suppl. 1): 133–151.
Keddy PA, Fraser LH, Solomeshch AI, Junk WJ, Campbell DR, Arroyon MTK, et al. 2009. Wet and wonderful: the world’s largest wetlands are conservation priorities. BioScience, 59(1): 39–51.
Keller W. 2007. Implications of climate warming for Boreal Shield lakes: a review and synthesis. Environmental Reviews, 15: 99–112.
Kukkala AS, and Moilanen A. 2013. Core concepts of spatial prioritisation in systematic conservation planning. Biological Reviews, 88: 443–464.
Land Information Ontario. 2009. Natural Resource Values Information System (NRVIS) data class user guide for aquatic resource area summary and aquatic resource area survey. Version 2009.1. Ontario Ministry of Natural Resources, Fisheries Management Branch, 49 p.
Lacho CD. 2013. Movement and habitat use of lake sturgeon (Acipencer fulvescens) in the South Saskatchewan River system. M.Sc. Thesis, University of Lethbridge, Lethbridge, Alberta. 90 p.
Leroy B, Meynard CN, Bellard C, and Courchamp F. 2016. virtualspecies, an R package to generate virtual species distributions. Ecography, 39(6): 599–607.
Linke S, Turak E, and Nel J. 2011. Freshwater conservation planning: the case for systematic approaches. Freshwater Biology, 56: 6–20.
Linke S, Kennard MJ, Hermoso V, Olden JD, Stein J, and Pussey BJ. 2012. Merging connectivity rules and large-scale condition assessment improves conservation adequacy in river systems. Journal of Applied Ecology, 49: 1036–1045.
Maidmant D. 2002. ArcHydro GIS for water resources. ESRI Press, Redlands, CA. 220 p.
Mandrak NE, and Crossman EJ. 1992. A checklist of Ontario freshwater fishes annotated with distribution maps. Royal Ontario Museum Life Sciences Miscellaneous Publication, Toronto, ON. 176 p.
Marshall TR, and Jones NE. 2011. Aquatic ecosystems in the Far North of Ontario state of knowledge. Ontario Ministry of Natural Resources. 43 p.
Margules CR, and Pressey RL. 2000. Systematic conservation planning. Nature, 405: 243–253.
McDermid J, Browne D, Chetkiewicz C-L, and Chu C. 2015. Identifying a suite of surrogate freshwaterscape fish species: a case study of conservation prioritization in Ontario’s Far North, Canada. Aquatic Conservation: Marin and Freshwater Ecosystems, 25(6): 855–873.
McIntosh EJ, Chapman S, Kearney SG, Williams B, Althor G, Thorn JPR, et al. 2018. Absence of evidence for the conservation outcomes of systematic conservation planning around the globe: a systematic map. Environmental Evidence, 7: 22.
Michalak JL, Carroll C, Nielsen SE, and Lawler JJ. 2018. Land facet data for North America at 100m resolution [data set]. Zenodo.
Natural Resources Canada. 2017. Canadian geographical names database [data set]: Available from nrcan.gc.ca/earth-sciences/geography/download-geographical-names-data/9245.
Nel JL, Roux DJ, Abell R, Ashton PJ, Cowling RM, Higgins JV, et al. 2009. Progress and challenges in freshwater conservation planning. Aquatic Conservation, 19(4): 474–485.
Noble M, Duncan P, Perry D, Prosper K, Rose D, Schnierer S, et al. 2016. Culturally significant fisheries: keystones for management of freshwater social-ecological systems. Ecology and Society, 21(2): 22.
Oksanen J, Blanchet FG, Friendly M, Kindt R, Legendre P, McGlinn D, et al. 2019. vegan: Community ecology package. R package version 2.5-4 [software package]: Available from cran.r-project.org/web/packages/vegan/index.html.
Ontario Geological Survey (OGS). 2017. Mineral deposit inventory (May 2017 update) [data set]: Available from geologyontario.mndm.gov.on.ca/index.html.
Ontario Ministry of Energy (MOE). 2017. Ontario’s long term energy plan 2017 [online]: Available from ontario.ca/document/2017-long-term-energy-plan.
Ontario Ministry of Northern Development and Mines (MNDM). 2017a. Abandoned mines information system (AMIS) database (November 2016 update) [data set]: Available from geologyontario.mndm.gov.on.ca/index.html.
Ontario Ministry of Northern Development and Mines (MNDM). 2017b. Mining claims database [data set]: Available from mndm.gov.on.ca/en/mines-and-minerals/applications/ogsearth/mining-claims.
Ontario Ministry of Infrastructure (MOI) and Ontario Ministry of Northern Development, Mines and Forestry (MNDMF). 2011. Growth plan for northern Ontario. Queen’s Printer for Ontario. 76 p.
Ontario Ministry of Natural Resources (MNR). 2012. Forest management plan for the whitefeather forest [online]: Available from efmp.lrc.gov.on.ca/eFMP/file?fid=239357.
Ontario Ministry of Natural Resources and Forestry (MNRF). 2017a. Aggregate site authorized active [data set]: Available from geohub.lio.gov.on.ca/datasets/aggregate-site-authorized-active.
Ontario Ministry of Natural Resources and Forestry (MNRF). 2017b. Aggregate site authorized inactive [data set]: Available from geohub.lio.gov.on.ca/datasets/aggregate-site-authorized-inactive.
Ontario Ministry of Natural Resources and Forestry (MNRF). 2019a. Proposal in support of the province’s review of the Far North Act [online]: Available from ero.ontario.ca/notice/013-4734.
Ontario Ministry of Natural Resources and Forestry (MNRF). 2019b. State of Ontario’s protected areas report [online]: Available from ontario.ca/page/state-ontarios-protected-areas-report.
Parrish JD, Braun DP, and Unnasch RS. 2003. Are we conserving what we say we are? Measuring ecological integrity within protected areas. BioScience, 53(9): 851–860.
Pettorelli N. 2012. Climate change as a main driver of ecological research. Journal of Applied Ecology, 49: 542–545.
Pimm SL, Jenkins CN, and Li BV. 2018. How to protect half of Earth to ensure it protects sufficient biodiversity. Science Advances, 4(8): eaat2616.
Phillips SJ, Anderson RP, and Schapire RE. 2006. Maximum entropy modeling of species geographic distributions. Ecological Modelling, 190(3-4): 231–259.
Phillips SJ, and Elith J. 2013. On estimating probability of presence from use–availability or presence–background data. Ecology, 94(6): 1409–1419.
Phillips SJ, Dudik M, and Schapire RE. 2017. Maxent software for modeling species niches and distributions (Version 3.3.3) [software package]: Available from biodiversityinformatics.amnh.org/open_source/maxent/.
Plotkin R. 2018. Tribal parks and indigenous protected and conserved areas: lessons from B.C. David Suzuki Foundation. 56 p.
Pringle CM. 2001. Hydrologic connectivity and the management of biological reserves: a global perspective. Ecological Applications, 11(4): 981–998.
Pringle CM. 2003. What is hydrologic connectivity and why is it ecologically important? Hydrological Processes, 17: 2685–2689.
Québec Ministère des Ressources naturelles et de la Faune (MRNF). 2016. Mining rights digital data [data set]: Available from mrn.gouv.qc.ca/public/Gestim/telechargements/Province_shape/.
Quinn GP, and Keough MJ. 2003. Experimental design and data analysis for biologists. University Press, Cambridge, UK.
Roberge J-M, and Anglstam P. 2004. Usefulness of the umbrella species concept as a conservation tool. Conservation Biology, 18(1): 76–85.
Sandstrom S, Rawson M, and Lester N. 2010. Manual of instructions for broad-scale fish community monitoring; using large mesh gillnets and small mesh gillnets. Version 2010.1. Ontario Ministry of Natural Resources, Peterborough ON, 34 p. + appendices.
Schindler DW, and Lee PG. 2010. Comprehensive conservation planning to protect biodiversity and ecosystem services in Canadian boreal regions under a warming climate and increasing exploitation. Biological Conservation, 143(7): 1571–1586.
Simberloff D. 1998. Flagships, umbrellas, and keystones: is single-species management passé in the landscape era? Biological Conservation, 83: 247–257.
Simpson GL. 2019. permute: Functions for Generating Restricted Permutations of Data. R package version 0.9-5 [software package]: Available from cran.r-project.org/web/packages/permute/index.html.
Smith AB. 2020. Best practices in species distribution modeling: workshop in R. Available from earthskysea.org/best-practices-in-species-distribution-modeling-a-workshop-in-r/.
Sowa SP, Annis G, Morey ME, and Diamond DD. 2007. A gap analysis and comprehensive conservation strategy for riverine ecosystems of Missouri. Ecological Monographs, 77: 301–334.
Species at Risk Act (SARA) (S.C. 2002, c.29). An Act respecting the protection of wildlife species at risk in Canada [online]: Available from laws-lois.justice.gc.ca/eng/acts/s-15.3/.
Statistics Canada. 2016. Age (in single years) and average age (127) and sex (3) for the population of Canada, provinces and territories, census divisions and census subdivisions, 2016 census – 100% data. Data table 98-400-X2016004 [data set]: Available from www12.statcan.gc.ca/census-recensement/2016/dp-pd/index-eng.cfm.
Strecker AL, Olden JD, Whittler JB, and Paukert CP. 2011. Defining conservation priorities for freshwater fishes according to taxonomic, functional, and phylogenetic diversity. Ecological Applications, 21(8): 3002–3013.
Tanentzap AJ, Szkokan-Emilson EJ, Kielstra BW, Arts MT, Yan ND, and Gunn JM. 2014. Forests fuel fish growth in freshwater deltas. Nature Communications, 5: 4077.
UNESCO. 2018. Decisions adopted during the 42nd session of the World Heritage Committee (Manama, 2018). WHC/18/42.COM/18.
Venter O, Fuller RA, Segan DB, Carwardine J, Brooks T, Butchart SH, et al. 2014. Targeting global protected area expansion for imperiled biodiversity. PLOS Biology, 12(6): e1001891.
Venter O, Magrach A, Outram N, Klein CJ, Possingham HP, Di Marco M, et al. 2018. Bias in protected‐area location and its effects on long‐term aspirations of biodiversity conventions. Conservation Biology, 32(1): 127–134.
Visconti P, Butchart SHM, Brooks TM, Langhammer PF, Marnewick D, Vergara S, et al. 2019. Protected area targets post-2020. Science, 364: 239–241.
Vörösmarty CJ, McIntyre PB, Gessner MO, Dudgeon D, Prusevich A, Green P, et al. 2010. Global threats to human water security and river biodiversity. Nature, 467(7315): 555–561.
Warren DL, and Seifert SN. 2011. Ecological niche modelling in Maxent: the importance of model complexity and the performance of model selection criteria. Ecological Applications 21(2): 335–342.
Watson JEM, Dudley N, Segan DB, and Hockings M. 2014. The performance and potential of protected areas. Nature, 515(7525): 67–73.
Wells J, Roberts D, Lee P, Cheng R, and Darveau M. 2010. A forest of blue—Canada’s boreal forest: the world’s waterkeeper. International Boreal Conservation Campaign, Seattle. 74 pp.
Weinzettel J, Vačkář D, and Medková H. 2018. Human footprint in biodiversity hotspots. Frontiers in Ecology and the Environment, 16(8): 447–452.
Wilkinson CJA, and Schulz T. 2012. Planning the Far North in Ontario, Canada: an examination of the “Far North Act, 2010”. Natural Areas Journal, 32(3), 310–315.
Wilson KA, Cabeza M, and Klein CJ. 2009. Fundamental concepts of spatial conservation prioritization. In Spatial conservation prioritization. Edited by A Moilanen, KA Wilson, and H Possingham. Oxford University Press. pp. 16–27.
Woodley S, Locke H, Laffoley D, MacKinnon K, Sandwith T, and Smart J. 2019. A review of evidence for area-based conservation targets for the post-2020 global biodiversity framework. Parks, 25(2): 31–46.
Zurba M, Beazley K, English E, and Buchmann-Duck J. 2019. indigenous protected and conserved areas (IPCAs), Aichi Target 11 and Canada’s pathway to target 1: focusing conservation on reconciliation. Land, 8(1).
Supplementary material
Supplementary Material 1 (DOCX / 32.7 KB)
- Download
- 32.71 KB
Information & Authors
Information
Published In
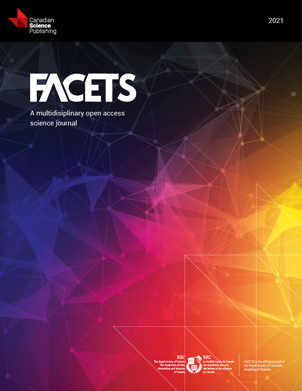
FACETS
Volume 6 • Number 1 • January 2021
Pages: 90 - 117
Editor: Brett Favaro
History
Received: 2 March 2020
Accepted: 11 September 2020
Version of record online: 28 January 2021
Copyright
© 2021 Southee et al. This work is licensed under a Creative Commons Attribution 4.0 International License (CC BY 4.0), which permits unrestricted use, distribution, and reproduction in any medium, provided the original author(s) and source are credited.
Data Availability Statement
All relevant data are within the paper and in the Supplementary Material.
Key Words
Sections
Subjects
Authors
Author Contributions
FMS, BAE, and CMO conceived and designed the study.
FMS, BAE, and CMO performed the experiments/collected the data.
FMS, BAE, and CMO analyzed and interpreted the data.
All drafted or revised the manuscript.
Competing Interests
The authors have declared that no competing interests exist.
Metrics & Citations
Metrics
Other Metrics
Citations
Cite As
F. Meg Southee, Brie A. Edwards, Cheryl-Lesley B. Chetkiewicz, and Constance M. O’Connor. 2021. Freshwater conservation planning in the far north of Ontario, Canada: identifying priority watersheds for the conservation of fish biodiversity in an intact boreal landscape. FACETS.
6: 90-117.
https://doi.org/10.1139/facets-2020-0015
Export Citations
If you have the appropriate software installed, you can download article citation data to the citation manager of your choice. Simply select your manager software from the list below and click Download.
Cited by
1. Occupancy, movement, and behaviour of namew (lake sturgeon Acipenser fulvescens) in an intact river in Canada
2. Global trends in geospatial conservation planning: a review of priorities and missing dimensions
3. Safety Net Ontario: Ontario’s outsized role in the “Global Safety Net” for climate and biodiversity
4. Protecting boreal caribou habitat can help conserve biodiversity and safeguard large quantities of soil carbon in Canada
5. A systematic review of the global freshwater mussel restoration toolbox