Thermal sensitivity and flow-mediated migratory delays drive climate risk for coastal sockeye salmon
Abstract
Climate change is subjecting aquatic species to increasing temperatures and shifting hydrologic conditions. Understanding how these changes affect individual survival can help guide conservation and management actions. Anadromous Pacific salmon (Oncorhynchus spp.) in some large river systems are acutely impacted by the river temperatures and flows encountered during their spawning migrations. However, comparatively little is known about drivers of en route mortality for salmon in smaller coastal watersheds, and climate impacts may differ across watersheds and locally adapted salmon populations. To understand the effects of climate on the survival of coastal sockeye salmon (Oncorhynchus nerka; hísn in Haíɫzaqv), we tagged 1785 individual fish with passive integrated transponders across four migration seasons in the Koeye River—a low-elevation watershed in coastal British Columbia—and tracked them during their relatively short migration (∼13 km) from river entry to spawning grounds. Overall, 64.7% of sockeye survived to enter the spawning grounds, and survival decreased rapidly when water temperatures exceeded 15 °C. The best-fitting model included an interaction between river flow and temperature, such that temperature effects were worse when flows were low, and river entry ceased at the lowest flows. Results revealed temperature-mediated mortality and migration delays from low water that may synergistically reduce survival among sockeye salmon returning to coastal watersheds.
Introduction
Variation in climate is a key determinant of species distributions (Parmesan and Yohe 2003; Perry et al. 2005), population productivity (Gjerdrum et al. 2003), age structure (Carlson and Quinn 2007), and rates of individual growth and survival (Ludwig et al. 2006; Martins et al. 2012a). The life cycles of migratory species link climate variability spanning vast distances as they traverse seasonal gradients through diverse habitats connecting abundant feeding areas with productive breeding grounds (Dingle 2014). Timing of migration, breeding, and other critical life-history events for these species has evolved to match climate conditions that maximize the survival and fitness of individuals (Cushing 1990; Bradshaw and Holzapfel 2008). Given their reliance on these varied and distant habitats, the energetic demands of migration and the importance of seasonal phenological transitions (Dingle 2014), migratory species may be especially vulnerable to climate change (Robinson et al. 2009). Given the paramount role of climate in survival among migratory species and the rapid ongoing progression of anthropogenic climate change (IPCC 2013), understanding how climate impacts migration and survival is foundational for conservation and management of these species.
In the coastal ecosystems of the Pacific Northwest of North America, anthropogenic warming is predicted to increase air temperatures and reduce precipitation during the summer and early fall months (Mote et al. 2003; Murdock and Spittlehouse 2011), altering water temperatures and flow during the freshwater phase of Pacific salmon (Oncorhynchus sp.) spawning migrations (Mantua et al. 2010; Murdock and Spittlehouse 2011; Isaak et al. 2018). Climate warming has the potential to undermine the long-term viability of cold-water fish populations such as salmon (Isaak et al. 2010; Crozier et al. 2019). Adult migration timing of populations can be adapted to the prevailing temperature and flow conditions within their natal watersheds (Hodgson and Quinn 2002; Beechie et al. 2006; Eliason et al. 2011). However, climate change has already increased water temperatures in many systems, pushing species to the limit of their thermal tolerance and driving shifts in migratory phenology (Quinn and Adams 1996; Reed et al. 2011; Kovach et al. 2013). In well-studied large river systems such as the Fraser River, where adult salmon make energetically costly migrations hundreds of kilometers upstream, extensive research has documented that high temperatures and high flows can increase en route mortality of salmon (Rand et al. 2006; Farrell et al. 2008; Martins et al. 2011). These relationships between survival and flow and temperature are now used to inform in-season fishery management, and fisheries harvest rates are reduced when environmental conditions are less favorable (Hague and Patterson 2007; MacDonald et al. 2010). Thus, there is a critical need to evaluate the behavioral and demographic consequences of increasing water temperatures for salmon populations and to incorporate this understanding of climate impacts into the management of fisheries.
Migratory salmon in smaller coastal watersheds may be more or less vulnerable to climate change than their large-river counterparts. While mortality during spawning migrations has been well documented in populations of sockeye salmon in the Fraser and Columbia Rivers (Crossin et al. 2008; Crozier et al. 2011; Martins et al. 2011), the extent and impact of temperature-mediated mortality in these smaller watersheds, such as those on the British Columbia coast, is virtually unknown. In the low-elevation coastal watersheds of Washington, British Columbia, and Alaska small- to medium-sized lakes support hundreds of populations of sockeye salmon. In British Columbia alone, these coastal watersheds support at least 120 unique stocks, more than half of the 214 lake-type sockeye salmon (juvenile rearing in lakes) populations in the province (Holtby and Ciruna 2007). These lakes often share common features such as rain-dominated hydrology and unproductive humic-stained waters (Stockner and McIsaac 1996). In coastal watersheds, sockeye salmon typically migrate during late spring and early summer, thereby avoiding late-summer water temperatures that can exceed 20 °C (Hodgson and Quinn 2002; Katinić et al. 2015), temperatures known to induce physiological stress and mortality among populations in the Fraser and Columbia Rivers (Crossin et al. 2008; Crozier et al. 2011; Eliason et al. 2011). Further, with small to medium catchment sizes (e.g., <300 km2), minimal snowmelt, and darkly colored water resulting from humic staining, coastal watersheds are likely to absorb solar energy more readily, making them sensitive to warming air temperatures (Lisi et al. 2015; Chezik et al. 2017). Smaller watersheds have more variable hydrology (Moore et al. 2015), and during summer drought periods many coastal systems experience low-flow conditions that limit movements by adult salmon (Quinn et al. 2015). However, migration distances for coastal salmon populations are typically shorter, perhaps only a few kilometers in length, compared with long-distance migrations in large rivers that impose greater energetic costs and cumulative thermal exposure. Local adaptation in thermal tolerance may also act to modulate population responses to warming (e.g., Eliason et al. 2011; Whitney et al. 2013). Importantly, these coastal sockeye salmon stocks support subsistence fisheries that are vital to the culture, economy, and food security of many remote and Indigenous communities. Despite their importance for the evolutionary legacy of their species, and the socio-cultural value of these populations, data are lacking to understand migratory behavior and climate sensitivity of sockeye salmon in coastal river systems.
Here we evaluate the potential vulnerability of coastal sockeye salmon to shifting hydrology and warming waters in a remote and undeveloped watershed. Specifically, we examine four years of tagging and survival data in a culturally important sockeye salmon population, the Koeye River of the Central Coast region of British Columbia, Canada. We worked with the Haíɫzaqv (Heiltsuk) Nation to build and operate a weir to tag adult sockeye salmon for subsequent mark-resight estimates of population size to inform management and harvest opportunities for food, social, and ceremonial purposes (Atlas et al. 2017). From 2016 to 2019, we installed and maintained an array of passive integrated transponder (PIT) antennas to track the migration and survival of 1785 sockeye salmon tagged and released at the weir. Using these data, we asked three questions: (i) How long does it take tagged sockeye salmon to reach Koeye Lake? (ii) What percentage of tagged stock survive to reach their spawning grounds, and what is the relationship between temperature, river level, and survival? (iii) Does river entry cease during periods of summer low water and cause migratory delays? By reconstructing detection histories for individual fish and modeling apparent survival in relation to the temperature and flow conditions encountered during their spawning migrations, we reveal new understanding of climate vulnerability for sockeye salmon in coastal watersheds. Estimates of temperature-mediated en route mortality will support forecasting of future climate impacts on sockeye populations in coastal ecosystems, underpinning adaptive management under climate change.
Methods
Study system
The Koeye River drains a watershed of 185 km2 on the mainland coast of British Columbia (Fig. 1). The hydrology of the watershed is transitional rain–snow dominant, with peak flows typically occurring during late fall and winter and a small pulse of snowmelt driven discharge during late spring. The watershed has two medium-sized lakes, Koeye Lake (450 ha) and Upper Koeye Lake (227 ha), which are lightly stained and mildly acidic (pH ∼ 6.7). Koeye Lake is located 6.2 km from tidewater at 53 m above sea level and supports all sockeye salmon spawning and rearing, as they are not believed to ascend the canyon downstream of Upper Koeye Lake. Importantly, the watershed is undeveloped, unlogged, and entirely protected within conservancy, a rarity among coastal watersheds of the Pacific Northwest.
Fig. 1.

Sockeye salmon return to the Koeye River from June through September. Most fish enter freshwater between mid-June and mid-July and hold over-summer in Koeye lake (Fig. 2). Spawning occurs in September and October in two tributaries of the lake, the Upper Koeye River and a smaller tributary that enters on the lake’s south shore—referred to hereafter as Left Tributary—that provides spawning habitat for a few hundred individuals annually. Lakeshore spawning is not believed to occur. Since the 1950s spawning sockeye salmon abundance in the Koeye River has ranged from a few thousand to 18 000; however, prior to 2013, population estimates were infrequent and unreliable (English et al. 2016).
Fig. 2.

Tagging and tracking
Since 2013, the Haíɫzaqv Nation and research partners have used a traditional-style cedar fish weir to capture and tag sockeye salmon in the Koeye River (Fig. 1), shortly after their freshwater entry (Atlas et al. 2017). In 2019, the weir was upgraded to a more durable floating aluminum fence, enabling higher capture efficiency at the weir site. Most years, the weir is installed in early June and operated until the end of July. Fish are captured in the trap box of the weir and in weekly seining events in the pool immediately downstream, anesthetized with tricaine mesylate and tagged with visually identifiable FLOY anchor tags (FLOY Tag, Seattle, Washington, USA) on both sides of the posterior dorsal muscle, and evaluated visually for recent wounds, net scarring, and major scale loss (Simon Fraser University Animal Care #1129B-14 and 1270B-14). Following twice-weekly pool seining, a panel is removed from the weir to minimize migration delays imposed by the fence. Only a small fraction of the total Koeye River sockeye return is captured and tagged at the weir. We aim to tag approximately 500 fish annually for mark-recapture estimates, which is <10% of the total return in most years. Tagged fish are subsequently resighted during repeated fall stream counts to generate estimates of spawner abundance.
From 2016 to 2019, we installed and maintained an array of solar and fuel-cell powered PIT antennas (Oregon RFID, Portland, Oregon, USA) across the longitudinal extent of the Koeye watershed. Initially, pass-over antennas were installed at three locations: the top of tidal influence about 300 m upstream of the weir, the lake outlet, and the lower end of the Upper Koeye River, which is situated above Koeye Lake where ∼85% of spawning typically occurs. The length of the antennas at these three sites ranged from 25 to 30 m. Antennas were built using a loop of 12-gauge antenna wire, covered in irrigation hose and tied to a lead line using braided nylon twine. For pass-over antennas, the two sides of the antenna loop were held approximately 1 m apart to maximize read range, and anchored to the stream bed using duckbill stream anchors (MacLean Civil Products, Fort Mill, South Carolina, USA) driven into the substrate.
In 2017, we added a smaller (15 m) seasonally deployed pass-over antenna in the lower end of the Left Tributary of Koeye Lake. PIT antennas in the Koeye River below the lake were installed in spring, prior to the start of the spawning migration. Antennas above the lake were installed in late-August or early-September, prior to entry on spawning grounds. The configuration and operational schedule of PIT antennas in the tributaries of Koeye Lake varied slightly among years. For example, the antenna in the Left Tributary of Koeye Lake was not installed until 2017, and in that year a bear damaged the equipment at the Upper Koeye River site shutting down the antenna for 8 d in mid-September. All antenna sites were operated until late October when spawning and river entry is complete.
Sockeye salmon captured at the weir were tagged with PIT tags (23 or 32 mm HDX) (Oregon RFID) implanted in the dorsal muscle in addition to the FLOY tags. Using this network of antennas, we tracked the migration and survival of PIT-tagged adult sockeye salmon from freshwater entry to spawning grounds. Across the four years of study, we tagged and released a total of 1785 adult sockeye salmon at the weir (Table 1), redetecting 1079 in spawning tributaries above Koeye Lake. Daily mean temperatures and river levels on the day of tagging were estimated using a Hobo U20 water level and temperature logger (Onset Corporation, Bourne, Massachusetts, USA) deployed in the lower Koeye River approximately 500 m above the weir. Sex, length, and injuries for tagged fish were recorded during handling. Net and predator injuries were treated equally when analyzing their effects on survival.
Table 1.
Year | Dates of operation | Number tagged | Temp, °C (μ) | Mean φ o (%) | Total survival (%) |
---|---|---|---|---|---|
2016 | 8 June–2 July | 193 | 14.42 | 91.3 (82.6–97.9) | 64.7 (54.9–76.5) |
2017 | 10 June–1 August | 587 | 15.88 | 89.7 (87.1–92.1) | 66.2 (56.9–79.3) |
2018 | 15 June–26 July | 382 | 16.15 | 83.8 (79.8–87.5) | 64.6 (59.0–70.4) |
2019 | 10 June–8 August | 627 | 17.02 | 90.8 (88.3–93.0) | 63.1 (58.5–67.9) |
Data analysis
We estimated the effects of water temperature, sex, river level, injury, and tag size on the apparent survival of adult sockeye salmon from the weir to spawning grounds above Koeye Lake. To understand associations between environmental conditions and survival, we compared coefficient estimates and statistical support for a series of Bayesian logistic regression models. These models were pared down from a full model that included the interaction between river level and mean daily water temperature, their main effects, and the effects of sex, individual injuries, whether the fish was tagged in the weir trap box or seined in the downstream pool, and tag size on the probability of being detected on the spawning grounds. Continuous environmental covariates were centered and standardized by subtracting the mean and dividing by two standard deviations, and coefficient estimates are reported in this way to facilitate comparisons of effect sizes across covariates (Gelman 2008). We accounted for the nonindependence of fish tagged on the same day by fitting a cohort-level random intercept term and included a year-specific random effect to account for variation in the configuration and operational schedule of the antenna array among years.
(1)
Our model estimated the probability of survival for individual sockeye tagged in the four-year study (p i ). The model included a random intercept (α) for each cohort (j), continuous environmental covariates γ n with the estimated coefficient β γn , the effect of injury (β Inj), tagging location (β weir), categorical tag size (β T), and a year-specific random intercept term (ε year) (eq. (1)). The effects of sex (β sex) and temperature (β temp) were included in all models, given evidence that both are important for survival in other systems (Martins et al. 2012b). Tagging location (weir trap box or seine) was also included in the model based on evidence that fish tagged at the weir trap box had lower postrelease survival. Priors for α were drawn from a hyper-distribution with a normally distributed mean of zero and a variance term τ, which was one over the standard deviation squared. Standard deviations for τ were drawn from a uniform distribution bounded between 0 and 2. For β, we used uninformative normally distributed priors with a mean of zero and a standard deviation of 10.
We compared models of apparent survival to spawning grounds using an information theoretic approach, computing widely applicable information criterion (WAIC) scores for each model using the R-package “loo” (Vehtari et al. 2018). We then evaluated evidence for effects of environmental conditions, sex, injury, and tag size on the probability of redetection on spawning grounds above Koeye Lake by comparing median coefficient estimates and credible intervals for each variable. For the suite of models receiving a high degree of support, we further estimated model predictive performance using an area under the curve approach implemented in the R-package “ROCR” (Sing et al. 2015) and tested the prediction accuracy at a 0.5 probability cutoff.
To estimate the migration rate and survival of sockeye salmon from the weir to Koeye Lake, we used detections at the lake outlet antenna site as indication of successful migration to over-summer holding areas in the lake. Survival estimates accounted for detection efficiency by estimating survival as part of a Bayesian multinomial probability statement, where N t is the number of fish tagged at the weir in a given year. This multinomial probability (Supplementary Material 1) accounted for all possible detection histories (e.g., N 1,1,1 for the number of fish detected at Outlet, Left Tributary, and Upper River) to estimate annual probabilities of survival to the lake outlet (phio,y), and transition into the Upper River (φ u,y) or Left Tributary (φ t,y) spawning areas.
Survival and detection efficiency were estimated by borrowing information across years and locations and by using prior information to constrain parameter estimates within reasonable bounds. Transition probabilities (survival) were estimated hierarchically assuming beta distributed probability. Alpha and beta priors for these transition probabilities were bounded between 2 and 5 for φ o and φ u. Examination of posteriors suggested different underlying distributions for the probability of transition into the Left Tributary (φ t) and we bounded uniform alpha priors for that site between 1.5 and 2.5 and beta priors between 6 and 9. Using these hierarchical transition probabilities we inferred the proportion of fish entering the Left Tributary in 2016 by drawing on data from the latter three years of the study.
Detection efficiency for the lake outlet for each year (ρ o,y) was estimated from the multinomial probability with uniform priors bounded between 0 and 1. After the first year of the study we corrected issues with electrical interference that had limited tag read ranges, and model estimates of detection efficiency were consistently >0.95 at the lake outlet site. Thus, for Upper River and Left Tributary sites at the upper extent of the array, where detection efficiency could not be estimated separately from survival probability, we used informative uniform priors bounded between 0.9 and 1 for detection efficiency. Because the Upper River antenna site lost power for 8 d in 2017 we likely missed between 15% and 30% of sockeye entering the Upper Koeye River. While some of these fish were undoubtedly detected later in the season during and after spawning, we bounded detection efficiency (ρ u,17) between 0.6 and 0.9 to account for uncertainty stemming from missed detections. Detection efficiency for the Left Tributary site in 2016 was fixed at 0. Overall survival for each year (S y) was then estimated as the product of survival probabilities to the lake outlet and into either of the spawning tributaries after subtracting the probability that a fish entered both spawning areas (eq. (2)).
All models were then run for 30 000 iterations in JAGS in the statistical program R (R Core Team 2017), using the rjags package (Plummer 2019), with three parallel chains, a burn in period of 20 000 iterations, and a thinning rate of five. Model convergence was evaluated visually using trace plots.
(2)
Results and discussion
Sockeye salmon spawning migrations began in early June with a peak in late June and early July (Fig. 2). Fish tagged at the weir migrated quickly to Koeye Lake taking an average of 3.73 d to reach the lake outlet; however, migration duration was highly variable and ranged from a minimum of 1.01 d to a maximum of 25.3 d. Across the four years, daily mean water temperatures during the migration period ranged from 11.5 to 20.5 °C, and median estimates of survival to Koeye Lake (φ o) ranged from 83.8% to 91.3% with an average of 88.9% (Table 1). Detection efficiency at the lake outlet site (ρ o) was lowest in the first year of the study (51.3%; CI 43.2%–59.6%) (all credible intervals reported are 95% CI); however, modifications to antennas and power systems designed to reduce electrical interference yielded dramatic improvements in detection efficiency in the latter three years of the study (mean = 97.9%; CI 96.0%–99.7%). Antennas installed at the Upper Koeye and Left Tributary sites indicated that on average 81.5% and 18.5% of spawners entered the two sites, respectively. Seventy-seven individuals (4.3% of fish) actually entered both spawning areas. An additional 24.2% mortality was estimated to have occurred during over-summer lake holding across the four years. These combined estimates of survival from tagging at the weir to Koeye Lake and from lake entry to spawning tributary entry yielded a mean survival to spawning of 64.7% (Table 1).
Bayesian logistic models of apparent survival to spawning, based on detection on antennas in spawning tributaries of Koeye Lake, revealed strong evidence for negative effects of elevated water temperature and injury on the probability of survival. Temperature, sex, injury, and tagging location (weir or pool seine) were included in all models receiving a high degree of support, and coefficient estimates for these variables did not overlap zero, indicating strong evidence of a negative effect on survival (Table S1). Two models received nearly equal WAIC support, one including only the main effects of temperature, sex, injury, and tagging location (Model 2), and another that also included the interaction between temperature and water level (Model 1). These models correctly predicted the survival of individual sockeye to spawning areas above Koeye Lake 65% and 64.9% of the time (Table 2). Parameter estimates for yearly random intercept terms trended towards lower detection probabilities in 2016 and 2017 when PIT antennas were not deployed in the Left Tributary and bears damaged the Upper River site for about a weak during September (Table S2).
Table 2.
Rank | Model | WAIC | SE | AUC | Prediction |
---|---|---|---|---|---|
1 | α j + β Temp Υ1 j : β Flow Υ2 j + β Sex Υ4 i + β Inj Υ3 i + β weir Υ6 i + ε year | 2318.9 | 25.5 | 0.669 | 0.650 |
2 | α j + β Temp Υ1 j + β Sex Υ4 i + β Inj Υ3 i + β weir Υ6 i + ε year | 2319.5 | 25.2 | 0.667 | 0.649 |
3 | α j + β Temp Υ1 j :β Sex Υ4 i + β Inj Υ3 i + β weir Υ6 i + ε year | 2320.3 | 25.4 | 0.670 | 0.645 |
4 | α j + β Temp Υ1 j + β Flow Υ2 j + β Sex Υ4 i + β Inj Υ3 i + β weir Υ6 i + ε year | 2320.5 | 25.4 | 0.668 | 0.649 |
5 | α j + β Temp Υ1 j :β Flow Υ2 j + β Sex Υ4 i + β Inj Υ3 i + β Tag Υ5 j + β weir Υ6 i + ε year | 2320.8 | 25.7 | 0.670 | 0.649 |
6 | α j + β Temp Υ1 j + β Sex Υ4 i + β Inj Υ3 i + β Tag Υ5 i + β weir Υ6 i + ε year | 2320.9 | 25.4 | 0.667 | 0.650 |
7 | α j + β Temp Υ1 j :β Inj Υ3 i + β Sex Υ4 i + β weir Υ6 i + ε year | 2321.3 | 25.3 | 0.668 | 0.649 |
8 | α j + β Temp Υ1 j :β Sex Υ4 i + β Inj Υ3 i + β Flow Υ2 j + β weir Υ6 i + ε year | 2321.3 | 25.5 | 0.670 | 0.645 |
9 | α j + β Temp Υ1 j :β Inj Υ3 i + β Sex Υ4 i + β Flow Υ2 j + β weir Υ6 i + ε year | 2322.4 | 25.4 | 0.668 | 0.649 |
10 | α j + β Temp Υ1 j + β Sex Υ4 i + β weir Υ6 i + ε year | 2328.7 | 24.4 | 0.665 | 0.646 |
Note
A cohort-specific intercept term α j was included in all models, as was a yearly intercept term (Epsilon). Models also included a range of environmental and individual predictors of survival (Y1–Y6) and their coefficients (β Temp,β Flow,β Sex,β Inj,β weir). Some models also included an interaction between predictors, denoted as “:”.
Higher water temperatures decreased apparent survival among sockeye salmon, and coefficient estimates were consistently negative for the temperature coefficient (Fig. 3;Table S1). However, the effect of temperature was modulated by the inclusion of interactions with flow, sex, or injury. For example, standardized coefficient estimates for temperature in models that included only main effects averaged −0.55 (CI −1.00 to −0.13) and never overlapped zero. In Model 1, which included an interaction between temperature and flow and received the greatest WAIC support, the estimated effect of increasing temperature was far greater but more uncertain (−1.75; CI −3.57 to −0.14) and was offset by a positive interaction between temperature and flow (1.88; CI −0.5 to 4.55). The interaction between temperature and flow meant that as flow decreased, the negative effects of warm temperature on survival were more acute (Figs. 3B–3D). Coefficient estimates for the main effect of flow was near zero in all models, with an average value of 0.04.
Fig. 3.

Mean temperature at tagging across the four years of study was 16.2 °C, and the two top models had similar median predictions of survival at this temperature. However, at the mean water level, Model 1 predicted higher survival at low temperatures and steeper declines in survival as temperatures increased (Table 3). For example, in Model 2, increasing temperature from 14 to 18 °C resulted in a decline in median predicted survival probability from 0.65 to 0.48 and 0.72 to 0.57 for females and males, respectively, that were tagged during pool seine events. By contrast, median predicted survival decreased with this same increase in temperature from 0.81 to 0.29 and 0.85 to 0.36 for females and males, respectively, in Model 1. When the interaction between water level and temperature was included, predicted survival among tagged sockeye salmon declined more steeply in response to temperature when flows were low. For example, a ∼30-cm increase in water depth from the lower quantile value of water levels among our tagging cohorts (0.48 m) to the upper quantile of water levels (0.74 m) resulted in an increase in predicted survival from 0.25 to 0.46 for males and from 0.19 to 0.38 for females at 18 °C (Figs. 3B and 3D). Few fish were tagged at the highest observed flows because these conditions often resulted in unimpeded passage at the weir.
Table 3.
Model 1: Temp: Flow + Sex + Injury | Model 2: Temp + Sex + Injury | ||||||||
---|---|---|---|---|---|---|---|---|---|
Female | Male | Female | Male | ||||||
Injury (°C) | No | Yes | No | Yes | No | Yes | No | Yes | |
Median | μ (16.2) | 0.56 | 0.38 | 0.64 | 0.47 | 0.54 | 0.36 | 0.62 | 0.44 |
survival | 10 | 0.79 | 0.65 | 0.84 | 0.72 | 0.98 | 0.95 | 0.98 | 0.97 |
14 | 0.65 | 0.48 | 0.72 | 0.56 | 0.81 | 0.67 | 0.85 | 0.74 | |
18 | 0.48 | 0.31 | 0.57 | 0.39 | 0.29 | 0.16 | 0.36 | 0.21 | |
22 | 0.32 | 0.18 | 0.39 | 0.24 | 0.04 | 0.02 | 0.05 | 0.03 |
Note
For Model 1, which included an interaction between flow and temperature, flow was set to the mean value. All estimates of survival are for fish tagged during pool seine events.
Female fish had a significantly lower probability of survival to spawning grounds (coefficient = −0.32, range of −0.52 to −0.12) than males, with a median estimate of 13% lower survival among females at the mean tagging temperature. Parameter estimates for the effect of injury was also consistent across models and averaged −0.72 (CI −1.13 to −0.33); on average injured fish had a 32% lower probability of surviving to spawning grounds. Likewise, coefficient estimates for the effect of tagging in the weir trap box—as opposed to during pool seining events—were consistently negative (coefficient = −0.27, range of −0.56 to 0.01). Among the 1785 individuals handled in the study, 119 had recent injuries from predators or gill nets. There was no evidence for differences in detection with tag size, and in all cases models without tag size received higher support (Tables 2 and S1).
The risk posed by warm water to migrating fish was reduced by a dramatic cessation in migration when flows were lowest and water temperatures were warmest. River entry as indexed by daily tagging numbers declined sharply when the gauge height dropped below 0.4 m, and only 11 fish were tagged under these low water conditions despite the fact that water levels fell below 0.4 m on 26 d across the four years of tagging (Fig. 4). This cessation of river entry meant that very few fish encountered the most inhospitable river conditions. In total only 201 of 1785 sockeye were tagged at temperatures above 18 °C, and these conditions occurred on day 31 of the 237 d of weir operation from 2016 to 2019. Thus, uncertain model fits at high temperatures were partially the result of low tagging numbers when temperatures exceeded 18 °C.
Fig. 4.

Overall, we found strong evidence for a rapid increase in mortality among migrating sockeye salmon when temperatures surpassed 15 °C and when water levels were lowest. The thermal sensitivity of sockeye salmon in the Koeye River therefore appears comparable with that of sockeye populations in the Fraser Basin (Martins et al. 2011). Thus, temperature-mediated mortality appears to be a potentially important source of climate vulnerability for coastal sockeye salmon despite their shorter summer migrations, possibly due to lower aerobic scope among coastal populations (Eliason et al. 2011).
We found higher risk of en route mortality among female sockeye, with females having 13% lower apparent survival compared with males at the mean tagging temperature. These findings are consistent with previous research revealing greater temperature sensitivity among female salmon (Martins et al. 2012b). Injuries from fishing nets or predators can also have lasting negative consequences for the physiological status, infection risk, and survival of migrating adult salmon and may reduce reproductive success among fish that survive to the spawning grounds (Baker and Schindler 2009;Bass et al. 2018;Teffer et al. 2018;Cook et al. 2019). We found that injured fish had a 32% lower probability of survival. Importantly, handling and tagging are likely associated with elevated mortality risk, particularly when water temperatures are high (Kieffer 2000;English et al. 2005). The observed relationship between survival rate and temperature in our study was most comparable with fish that were handled shortly after freshwater entry by Martins et al. (2011). Thus, rates of temperature-mediated en route mortality among unhandled fish in the Koeye River may be lower than we report here. Imperfect detection of survival to spawning grounds likely increases uncertainty in estimated temperature effects. However, it is unlikely to bias overall temperature and survival trends, since the probability of detection during spawning ground entry is unrelated to the temperature experienced during summer migration.
Mortality of migrating sockeye salmon occurred both during their lower river migration and during over-summer lake holding. On average, 31.5% of mortality occurred between tagging and the lake outlet, and the remaining 68.5% of mortality occurred in the lake prior to spawning ground entry, suggesting that both immediate survival through lower river migrations and delayed mortality related to physiological stress and condition can contribute to reduced spawning success. In general, sockeye salmon migrated through the lower river to Koeye Lake in just a few days, with an average migration time of 3.73 d. These relatively short migrations to Koeye Lake likely reduce the risk of predation or acute temperature-driven mortality, since fish can find and use cooler water in the hypolimnion of Koeye Lake for over-summer thermal refugia (W. Atlas, unpublished data). On average fish in the Koeye River entered spawning tributaries 88 d after tagging at the weir (range = 42–129 d). There are no fisheries in Koeye Lake. However, the metabolic and physiological consequences of exposure to high temperatures or other stressors clearly play out over a prolonged period in adult sockeye salmon, and delayed mortality following high temperature, handling stress or injury, has been reported in other sockeye populations (Crossin et al. 2008;Baker and Schindler 2009;Bass et al. 2018).
This population of coastal sockeye salmon migrate upstream prior to peak river temperatures in most years. While this early migration may minimize the risk of exposure to warm river temperatures it does comes at an energetic cost, since fish forgo foraging opportunities in the ocean and do not feed in freshwater (Quinn et al. 2015;Katinić et al. 2017). Despite these forgone growth opportunities, early migration and prolonged lake holding is characteristic of many coastal sockeye salmon populations (Katinić et al. 2015) and may increase reproductive success by allowing sockeye to behaviorally thermoregulate by moving across temperature gradients in lake hypolimnions (Newell and Quinn 2005;Roscoe et al. 2010). Early arriving fish with prolonged lake holding can more readily regulate their thermal exposure, contributing to longer spawning ground residence and lower egg retention, indicative of higher reproductive output (Minke-Martin et al. 2018).
It appears that flow may mediate the impacts of temperature on survival. We predicted higher mortality at lower river levels, as migrating salmon in smaller river systems can be vulnerable to predation or become stranded in low and warming pools when water levels decrease rapidly (Carlson and Quinn 2007). Unlike the Fraser and other large river systems where the en route survival of sockeye salmon is negatively impacted by the energetic costs associated with migrating during high flows (Rand et al. 2006), survival during the relatively short migrations of many coastal populations of sockeye salmon is likely bolstered by higher water levels. WAIC support was highest for a model (Model 1) that included an interaction between river temperature and flow, with median coefficient estimates supporting the conclusion that higher water levels moderate the negative effects of warmer temperatures. The uncertain effects of water level on survival may reflect the relatively narrow range of flows that most fish were tagged at or interannual differences in the overall risk of en route mortality that were driven by unmeasured factors (e.g., differences in pathogen prevalence). Regardless, the trend towards higher survival at higher flow highlights key differences between the impacts of climate-induced changes in hydrology on salmon in coastal rivers like the Koeye and larger rivers like the Fraser.
Low river flows not only seemed to exacerbate temperature-induced mortality but also delayed migration, as river entry by adult sockeye salmon stopped during low water. This observation has important implications for how we understand and forecast climate impacts in populations of coastal sockeye salmon. Climate models predict warmer and drier summers in the coastal Pacific Northwest (Murdock and Spittlehouse 2011). However, if sockeye in the Koeye River and other coastal river systems delay freshwater entry because of low water and remain in coastal marine waters longer, increased vulnerability to predation and fisheries may act as proximate drivers of mortality and reduced population viability. During the summer of 2018, the Central Coast region experienced a prolonged drought, with <6 mm of rainfall between 8 July and 24 August (Hakai Institute, unpublished data). Drought conditions overlapped with the latter half of the sockeye spawning migration, and in late August we observed large numbers of sockeye displaying spawning colors while holding in saltwater at the mouth of the nearby Namu River, almost a month before spawning typically begins.
As climate warms and summer droughts intensify, the interactive effects of increased temperature stress and migratory delays in saltwater could reduce survival among coastal sockeye salmon arriving at their natal river mouths at historically optimal times. Steep declines in the probability of individual survival when river temperatures exceed 15 °C indicate that coastal sockeye salmon have relatively low thermal tolerance (Eliason et al. 2011), despite the regularity of low, warm water conditions that often surpass this threshold during mid-summer. The cessation of migration during the lowest water conditions suggests that behavioral mechanisms may drive migratory delays, allowing fish to avoid the most stressful river conditions. However, waiting for summer rains to increase river levels and reduce water temperatures may increase the risk of predation, capture in fisheries, or injury during prolonged marine holding, reducing the overall likelihood of survival (Morita 2019). Such impacts of climate-induced delays in migrations could be exacerbated by increasing marine mammal populations (Olesiuk 2010;Chasco et al. 2017;Nelson et al. 2019). The dual impacts of temperature and prolonged saltwater holding are likely to have consequences for population productivity and sustainable harvest opportunities, as well as the long-term viability of hundreds of sockeye salmon populations across the coastal watersheds of Washington, British Columbia, and Alaska. Thus, management strategies that account for migration conditions by reducing fishing pressure during prolonged periods of low-warm water may be necessary to mitigate the effects of migration delays resulting from changing climate conditions. Similar climate-linked management models have been developed for the Fraser River, with reductions in harvest rates when temperatures reach levels predicted to elevate the risk of en route mortality among adult sockeye salmon (Hague and Patterson 2007;MacDonald et al. 2010).
Conclusions
Our observations that contemporary climatic variability is driving substantial mortality of migrating sockeye salmon in this intact coastal watershed is important for several reasons. First, these findings reveal that coastal sockeye salmon are also vulnerable to climate change despite their short migrations and maritime climates. Thus, climate risks extend beyond large river systems with long migrations and higher cumulative thermal exposure (Patterson et al. 2007). Second, it is important to emphasize that the study watershed is entirely undeveloped, having never been logged; thus, it has not suffered from land-use driven temperature increases and altered flow regimes (Tschaplinski and Pike 2017;Gronsdahl et al. 2019). Previous observations of temperature-induced mortality in migratory salmon generally originate from watersheds that have suffered from substantial human land-use alterations (Martins et al. 2011;Barnett et al. 2020). We find that even watersheds with intact habitat are vulnerable to oncoming climate changes, and sockeye salmon in coastal systems that have been logged or otherwise degraded are likely even at greater climate risk than we observed here.
Adaptive shifts in migration timing are likely to be an important process in maintaining viable populations of sockeye salmon given projected climate warming (Hague et al. 2011;Reed et al. 2011). Migration timing is highly heritable (Carlson and Seamons 2008), and species phenology may be more capable of rapid contemporary evolution than thermal tolerance (Bradshaw and Holzapfel 2008). Sockeye salmon grow slowly during their last summer of marine life, thus the fitness tradeoffs between early migration reducing mortality risk and additional marine growth associated with later migration likely favor earlier migration (Katinić et al. 2017). Within coastal populations there is precedent for migrations earlier than is currently observed among sockeye salmon in the Koeye River (Hodgson and Quinn 2002). For example, most populations of sockeye salmon in Haida Gwaii migrate earlier, beginning in April and May (Katinić et al. 2015), and some populations in smaller, low-elevation watersheds within Haíɫzaqv territory begin their freshwater migrations as early as late April (W. Housty personal communication, 2019). Given this precedent for earlier-run timing, and ongoing examples of adaptive shifts in sockeye salmon migration timing (e.g., Quinn and Adams 1996), climate warming and the loss of late-spring snowmelt may drive populations in many watersheds towards earlier migrations. Alternatively, some sockeye populations in the lower Fraser River with short migrations have traditionally delayed freshwater entry until September when river temperatures cool (Hinch et al. 2012). Thus, climate warming may push some portion of sockeye salmon migrations later, particularly if summer droughts preclude river entry during the latter half of the run.
To meet and manage oncoming climate impacts species conservation and management should seek to maintain diverse phenotypes by minimizing artificial selection imposed by harvest or other human activities (Allendorf and Hard 2009). Maintaining phenotypic diversity and population size underpins the potential for adaptation to drive evolutionary rescue in populations threatened by changes in climate, promoting the persistence and recovery of species in the face of ongoing anthropogenic climate change (Rice and Emery 2003;Carlson et al. 2014). Moreover, understanding climate impacts on migrating adult salmon can inform climate-resilient harvest regimes, reducing fishery intensity in warm and dry years where more salmon are expected to perish. Quantifying climate impacts and risks during animal migrations is a critical element of management and conservation efforts seeking to promote adaptation and resilience under climate change. Moving forward, these data will support development of adaptive-harvest management plans for Haíɫzaqv sockeye salmon fisheries.
Acknowledgements
This project was supported financially by the Tula Foundation, TIDES Canada, the Pacific Salmon Foundation, the Vancouver Foundation, and the McLean Foundation. The authors would like to acknowledge the many people involved in this project. We thank Morgan Hocking for his initial motivation and instigation of efforts to improve sockeye salmon monitoring in the Koeye River. Jess Housty contributed to grant writing and project visioning throughout. Louisa Housty and Larry Jorgenson provided vital logistical and administrative support. Salmon program technicians contributed tremendously to weir building and daily project operations including Richard Wilson-Hall, Jeremy Jorgenson, Jared Reid, Bryant DeRoy, Robert Duncan, Howard Duncan, Liam Nazarek, Jefferson Brown, Ray Wilson, Scott Lawson, Triston Johnson, Colin Reid, Robert Johnson, Chris Johnson, Jacey Humchitt, Jessel Housty, Leon Shaw, Blake Carpenter, Randall Housty, Ethan Gladstone, and Cecil Brown. We would also like to extend thanks to the many volunteers who participated in salmon tagging and counts including Julian Heavyside, Olivia Leigh Nowak, Phil Climie, Yohanna Gordon-Walker, Ilja Herb, Aaron Ditchfield, Alex Laliberte, Simon Aufderheide, Chris Deeg, Gaelen Krause, Damien Jannet, and Jade Steele. Grant Callegari contributed time and labour to the weir design and construction. Maurice Coulter-Boisvert contributed plans and design consultation for the aluminum weir. Colby Owen developed and installed the power systems for the PIT antennas, Shawn Hateley and Ingmar Lee assisted with installation of RFID antennas and power systems. Thanks to Audrey Béliveau and Carl Schwarz for Bayesian modeling advice and early help in JAGS. Also, a special thanks to Laurie Wood for administrative and budget support. Will Atlas received funding support through a Hakai Fellowship at Simon Fraser. Jonathan Moore is supported by the Liber Ero Foundation.
References
Allendorf FW, and Hard JJ.2009.Human-induced evolution caused by unnatural selection through harvest of wild animals.Proceedings of the National Academy of Sciences of the United States of America,106(Suppl. 1):9987–9994.
Atlas WI,Housty WG,Béliveau A,DeRoy B,Callegari G,Reid M,et al. 2017.Ancient fish weir technology for modern stewardship: lessons from community-based salmon monitoring.Ecosystem Health and Sustainability,3(6):1341284.
Baker MR, and Schindler DE.2009.Unaccounted mortality in salmon fisheries: non-retention in gillnets and effects on estimates of spawners.Journal of Applied Ecology,46(4):752–761.
Barnett HK,Quinn TP,Bhuthimethee M, and Winton JR.2020.Increased prespawning mortality threatens an integrated natural- and hatchery-origin sockeye salmon population in the Lake Washington Basin.Fisheries Research,227:105527.
Bass A,Hinch SG,Casselman MT,Bett NN,Burnett NJ,Middleton CT,et al. 2018.Visible gill-net injuries predict migration and spawning failure in adult sockeye salmon.Transactions of the American Fisheries Society,147(6):1085–1099.
Beechie T,Buhle E,Ruckelshaus M,Fullerton A, and Holsinger L.2006.Hydrologic regime and the conservation of salmon life history diversity.Biological Conservation,130(4):560–572.
Bradshaw WE, and Holzapfel CM.2008.Genetic response to rapid climate change: it’s seasonal timing that matters.Molecular Ecology,17(1):157–166.
Carlson SM, and Quinn TP.2007.Ten years of varying lake level and selection on size-at-maturity in sockeye salmon.Ecology,88(10):2620–2629.
Carlson SM, and Seamons TR.2008.A review of the quantitative genetic components of fitness in salmonids: implications for adaptation to future change.Evolutionary Applications,1:222–238.
Carlson SM,Cunningham CJ, and Westley PAH.2014.Evolutionary rescue in a changing world.Trends in Ecology & Evolution,29(9):521–530.
Chasco BE,Kaplan IC,Thomas AC,Acevedo-Gutiérrez A,Noren DP,Ford MJ,et al. 2017.Competing tradeoffs between increasing marine mammal predation and fisheries harvest of Chinook salmon.Scientific Reports,7(1):15439.
Chezik KA,Anderson SC, and Moore JW.2017.River networks dampen long-term hydrological signals of climate change.Geophysical Research Letters,44(14):7256–7264.
Cook KV,Hinch SG,Drenner SM,Raby GD,Patternson DA, and Cooke SJ.2019.Dermal injuries caused by purse seine capture result in lasting physiological disturbances in coho salmon.Comparative Biochemistry and Physiology, Part A,227(1):75–83.
Crossin GT,Hinch SG,Cooke SJ,Welch DW,Patterson DA,Jones SRM,et al. 2008.Exposure to high temperature influences the behavior, physiology, and survival of sockeye salmon during spawning migration.Canadian Journal of Zoology,86(2):127–140.
Crozier LG,Scheuerell MD, and Zabel RW.2011.Using time series analysis to characterize evolutionary and plastic responses to environmental change: a case study of a shift toward earlier migration date in Sockeye Salmon.The American Naturalist,178(6):755–773.
Crozier LG,McClure MM,Beechie T,Bograd SJ,Boughton DA,Carr M,et al. 2019.Climate vulnerability assessment for Pacific salmon and steelhead in the California Current Large Marine Ecosystem.PLoS ONE,14(7):e0217711.
Cushing,DH.1990.Plankton production and year-class strength in fish populations: an update of the match/mismatch hypothesis.Advances in Marine Biology,26(1):249–293.
Dingle H.2014.Migration: the biology of life on the move.2nd edition.Oxford University Press,Oxford, UK.340 p.
Eliason EJ,Clark TD,Hague MJ,Hanson LM,Gallagher ZS,Jeffries KM,et al. 2011.Differences in thermal tolerance among sockeye populations.Science,332(6025):109–112.
English KK,Koski WR,Sliwinski C,Blakely A,Cass A, and Woodey JC.2005.Migration timing and river survival of late-run Fraser River sockeye salmon estimated using radio telemetry techniques.Transactions of the American Fisheries Society,134(5):1342–1365.
English KK,Peacock D,Challenger W, and Mochizuki T.2016.North and central coast salmon escapement, catch, run size and exploitation rate estimates for each salmon conservation unit for 1954–2014.Prepared by LGL Limited Environmental Research for Pacific Salmon Foundation, and Fisheries Oceans Canada.98 p.
Farrell AP,Hinch SG,Cooke SJ,Patterson DA,Crossin GT,Lapointe M,et al. 2008.Pacific salmon in hot water: applying aerobic scope models and biotelemetry to predict the success of spawning migrations.Physiological and Biochemical Zoology,81(6):697–709.
Gelman A.2008.Scaling regression inputs by dividing by two standard deviations.Statistics in Medicine,27(15):2865–2873.
Gjerdrum C,Vallée AMJ,St. Clair CC,Bertram DF,Ryder JL, and Blackburn GS.2003.Tufted puffin reproduction reveals ocean climate variability.Proceedings of the National Academy of Sciences of the United States of America,100(16):9377–9382.
Gronsdahl S,Moore RD,Rosenfeld J,McCleary R, and Winkler R.2019.Effects of forestry on summertime low flows and physical fish habitat in snowmelt-dominant headwater catchments of the Pacific Northwest.Hydrological Processes,33(25):3152–3168.
Hague MJ, and Patterson DA.2007.Quantifying the sensitivity of Fraser River sockeye salmon (Oncorhynchus nerka) management adjustment models to uncertainties in run timing, run shape, and run profile. Canadian Technical Reports in Fisheries and Aquatic Science 2776.
Hague MJ,Ferrari MR,Miller JR,Patterson DA,Russell GL,Farrell AP,et al. 2011.Modeling the future hydroclimatology of the lower Fraser River and its impacts on the spawning migration survival of sockeye salmon.Global Change Biology,17(1):87–98.
Hinch SG,Cooke SJ,Farrell AP,Miller KM,Lapointe M, and Patterson DA.2012.Dead fish swimming: a review of research on the early migration and high premature mortality in adult Fraser River sockeye salmon Oncorhynchus nerka.Journal of Fish Biology,81:576–599.
Hodgson S, and Quinn TP.2002.The timing of adult sockeye salmon migrations into fresh water: adaptations by populations to prevailing thermal regimes.Canadian Journal of Zoology,80(3):542–555.
Holtby LB, and Ciruna KA.2007.Conservation units for Pacific salmon under the wild salmon policy. Canadian Science Advisory Secretariat Research Document 2007/070.
IPCC.2013.Climate Change 2013: the physical science basis. Contribution of Working Group I to the Fifth Assessment Report of the Intergovernmental Panel on Climate Change.Edited by TF Stocker,D Qin,GK Plattner,M Tignor,SK Allen,J Boschung,et al. Cambridge University Press,Cambridge, UK and New York, New York.1535 p.
Isaak DJ,Luce CH,Rieman BE,Nagel DE,Peterson EE,Horan DL,et al. 2010.Effects of climate change and wildfire on stream temperatures and salmonid thermal habitat in a mountain river network.Ecological Applications,20(5):1350–1371.
Isaak DJ,Luce CH,Horan DL,Chandler GL,Wollrab SP, and Nagel DE.2018.Global warming of salmon and trout rivers in the Northwestern U.S.: road to ruin or path through purgatory? Transactions of the American Fisheries Society,147(3):566–587.
Katinić PJ,Patterson DA, and Ydenberg RC.2015.Thermal regime, predation danger and the early marine exit of sockeye salmon Oncorhynchus nerka.Journal of Fish Biology,86(1):276–287.
Katinić PJ,Patterson DA, and Ydenberg RC.2017.Condition dependence in the marine exit timing of sockeye salmon (Oncorhynchus nerka) returning to Copper Creek, Haida Gwaii.Canadian Journal of Fisheries and Aquatic Sciences,74(1):15–22.
Kieffer JD.2000.Limits to exhaustive exercise in fish.Comparative Biochemistry and Physiology,126A(2):161–179.
Kovach RP,Joyce JE,Echave JD,Lindberg MS, and Tallmon DA.2013.Earlier migration timing, decreasing phenotypic variation, and biocomplexity in multiple salmon species.PLoS ONE,8(1):e53807.
Lisi PJ,Schindler DE,Cline TJ,Scheuerell MD, and Walsh PB.2015.Watershed geomorphology and snowmelt control stream thermal sensitivity to air temperature.Geophysical Research Letters,42(9):3380–3388.
Ludwig GX,Alatalo RV,Helle P,Lindén H,Lindström J, and Siitari H.2006.Short- and long-term population dynamical consequences of asymmetric climate change in black grouse.Proceedings of the Royal Society B: Biological Sciences,273(1597):2009–2016.
MacDonald JS,Patterson DA,Hague MJ, and Guthrie IC.2010.Modeling the influence of environmental factors on spawning migration mortality for sockeye salmon fisheries management in the Fraser River, British Columbia.Transactions of the American Fisheries Society,139(3):768–782.
Mantua N,Tohver I, and Hamlet A.2010.Climate change impacts on streamflow extremes in summertime stream temperature and their possible consequences for freshwater salmon habitat in Washington State.Climatic Change,102(1):187–223.
Martins EG,Hinch SG,Patterson DA,Hague MJ,Cooke SJ,Miller KM,et al. 2011.Effects of river temperature and climate warming on stock-specific survival of adult migrating Fraser River sockeye salmon (Oncorhychus nerka).Global Change Biology,17(1):99–114.
Martins EG,Hinch SG,Cooke SJ, and Patterson DA.2012a.Climate effects on growth, phenology, and survival of sockeye salmon (Oncorhynchus nerka): a synthesis of the current state of knowledge and future research directions.Reviews in Fish Biology and Fisheries,22(2012):887–914.
Martins EG,Hinch SG,Patterson DA,Hague MJ,Cooke SJ,Miller KM,et al. 2012b.High river temperature reduces survival of sockeye salmon approaching spawning grounds and exacerbates female mortality.Canadian Journal of Fisheries and Aquatic Sciences,69(2):330–342.
Minke-Martin V,Hinch SG,Braun DC,Burnett NJ,Casselman MT,Eliason EJ,et al. 2018.Physiological condition and migratory experience affect fitness-related outcomes in adult female sockeye salmon.Ecology of Freshwater Fish,27(1):296–309.
Moore JW,Beakes MP,Nesbitt HK,Yeakel JD,Patterson DA,Thompson LA,et al. 2015.Emergent stability in a large, free-flowing watershed.Ecology,96(2):340–347.
Morita K.2019.Earlier migration timing of salmonids: an adaptation to climate change or maladaptation to the fishery? Canadian Journal of Fisheries and Aquatic Sciences,76(3):475–479.
Mote PW,Parson EA,Hamlet AF,Keeton WS,Lettenmaier D,Mantua N,et al. 2003.Preparing for climatic change: the water, salmon, and forests of the Pacific Northwest.Climatic Change,61:45–88.
Murdock TQ, and Spittlehouse DL.2011.Selecting and using climate change scenarios for British Columbia.Pacific Climate Impacts Consortium, University of Victoria,Victoria, British Columbia.39 p.
Nelson BW,Walters CJ,Trites AW, and McAllister MK.2019.Wild Chinook salmon productivity is negatively related to seal density and not related to hatchery releases in the Pacific Northwest.Canadian Journal of Fisheries and Aquatic Sciences,76(3):447–462.
Newell JC, and Quinn TP.2005.Behavioral thermoregulation by maturing adult sockeye salmon (Oncorhynchus nerka) in a stratified lake prior to spawning.Canadian Journal of Zoology,83(9):1232–1239.
Olesiuk PF.2010.An assessment of population trends and abundance of harbour seals (Phoca vitulina) in British Columbia. DFO Canadian Science Advisory Secretariat Research Document 2009/105.
Parmesan C, and Yohe G.2003.A globally coherent fingerprint of climate change impacts across natural systems.Nature,421(6918):37–42.
Patterson DA,Macdonald JS,Skibo KM,Barnes DP,Gurthrie I, and Hills J.2007.Reconstructing the summer thermal history for the lower Fraser River, 1941 to 2006, and implications for adult sockeye salmon (Oncorhynchus nerka) spawning migration. Canadian Technical Report of Fisheries and Aquatic Sciences 2724.
Perry AL,Low PJ,Ellis JR, and Reynolds JD.2005.Climate change and distribution shifts in marine fishes.Science,308(5730):1912–1915.
Plummer M.2019.R-package ‘rjags’. Version 4-10.
Quinn TP, and Adams DJ.1996.Environmental changes affecting the migratory timing of American shad and sockeye salmon.Ecology,77(4):1151–1162.
Quinn TP,McDinnity P, and Reed TE.2015.The paradox of “premature migration” by adult anadromous salmonid fishes: patterns and hypotheses.Canadian Journal of Fisheries and Aquatic Sciences,73(7):1016–1030.
R Core Team.2017.R 3.4.0: a language and environment for statistical computing.R Foundation for Statistical Computing,Vienna, Austria [online]: Available from R-project.org/.
Rand PS,Hinch SG,Morrison J,Foreman MGG,MacNutt MJ,Macdonald JS,et al. 2006.Effects of river discharge, temperature, and future climates on energetics and mortality of adult migrating Fraser River sockeye salmon.Transactions of the American Fisheries Society,135(3):655–667.
Reed TE,Schindler DE,Hague MJ,Patterson DA,Meir E,Waples RS,et al. 2011.Time to evolve? Potential evolutionary responses of Fraser River sockeye salmon to climate change and effects on persistence.PLoS ONE,6(6):e20380.
Rice KJ, and Emery NC.2003.Managing microevolution: restoration in the face of global change.Frontiers in Ecology and the Environment,1(9):469–478.
Robinson RA,Crick HQP,Learmonth JA,Maclean IMD,Thomas CD,Bairlein F,et al. 2009.Travelling through a warming world: climate change and migratory species.Endangered Species Research,7(1):87–99.
Roscoe DW,Hinch SG,Cooke SJ, and Patterson DA.2010.Behavior and thermal experience of adult sockeye salmon migrating through stratified lakes near spawning grounds: roles of reproductive and energetic states.Ecology of Freshwater Fish,19(1):51–62.
Sing T,Sander O,Beerenwinkel N, and Lengauer T.2015.R-package ‘ROCR’: visualizing the performance of scoring classifiers. Version 1.0-7.
Stockner JG, and MacIsaac EA.1996.British Columbia lake enrichment programme: two decades of habitat enhancement for sockeye salmon.Regulated Rivers: Research and Management,12(5):547–561.
Teffer AK,Bass AL,Miller KM,Patterson DA,Juanes F, and Hinch SG.2018.Infections, fisheries capture, temperature, and host responses: multistressor influences on survival and behavior of adult Chinook salmon.Canadian Journal of Fisheries and Aquatic Sciences,75(11):2069–2083.
Tschaplinski PJ, and Pike RG.2017.Carnation creek watershed experiment—long-term responses of coho salmon populations to historic forest practices.Ecohydrology,10(2):e1812.
Vehtari A,Gelman A,Gabry J,Yao Y,Piironen J, and Goodrich B.2018.R-package ‘loo’: efficient leave-one-out cross-validation and WAIC for Bayesian models. Version 2.0.0.
Whitney CK,Hinch SG, and Patterson DA.2013.Provenance matters: thermal reaction norms for embryo survival among sockeye salmon Oncorhynchus nerka population.Journal of Fish Biology,82(4):1159–1176.
Supplementary materials
Supplementary Material 1 (DOCX / 14.1 KB)
- Download
- 14.13 KB
Supplementary Material 2 (XLSX / 12.8 KB)
- Download
- 12.80 KB
Supplementary Material 3 (XLSX / 11 KB)
- Download
- 11.08 KB
Information & Authors
Information
Published In
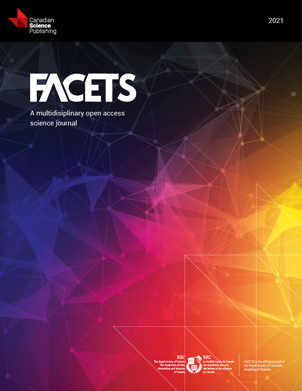
FACETS
Volume 6 • Number 1 • January 2021
Pages: 71 - 89
Editor: Daniel E. Schindler
History
Received: 18 April 2020
Accepted: 3 September 2020
Version of record online: 28 January 2021
Copyright
© 2021 Atlas et al. This work is licensed under a Creative Commons Attribution 4.0 International License (CC BY 4.0), which permits unrestricted use, distribution, and reproduction in any medium, provided the original author(s) and source are credited.
Data Availability Statement
All relevant data are within the paper and Supplementary Material.
Key Words
Sections
Subjects
Plain Language Summary
Climate change risks for migrating salmon are shaped by warming and drought in coastal rivers
Authors
Author Contributions
WIA, DR-E, NJB, and JWM conceived and designed the study.
WIA, KMS, JWNJ, BM-M, and WGH performed the experiments/collected the data.
WIA, WGH, and MR analyzed and interpreted the data.
MR and JWM contributed resources.
WIA, KMS, WGH, NJB, and JWM drafted or revised the manuscript.
Competing Interests
The authors have declared that no competing interests exist.
Metrics & Citations
Metrics
Other Metrics
Citations
Cite As
William I. Atlas, Karl M. Seitz, Jeremy W.N. Jorgenson, Ben Millard-Martin, William G. Housty, Daniel Ramos-Espinoza, Nicholas J. Burnett, Mike Reid, and Jonathan W. Moore. 2021. Thermal sensitivity and flow-mediated migratory delays drive climate risk for coastal sockeye salmon. FACETS.
6(): 71-89. https://doi.org/10.1139/facets-2020-0027
Export Citations
If you have the appropriate software installed, you can download article citation data to the citation manager of your choice. Simply select your manager software from the list below and click Download.
Cited by
1. Climate impacts to inland fishes: Shifting research topics over time
2. Using repeat injury assessments in adult sockeye salmon (Oncorhynchus nerka) to predict spawning success and describe severity of migration conditions
3. Changes in infectious agent profiles and host gene expression during spawning migrations of adult sockeye salmon (Oncorhynchus nerka)
4. The social-ecological system of the Kenai River Fishery (Alaska, USA)
5. Life Cycle Model Reveals Sensitive Life Stages and Evaluates Recovery Options for a Dwindling Pacific Salmon Population
6. Quantifying regional patterns of collapse in British Columbia Central Coast chum salmon (Oncorhynchus keta) populations since 1960
7. Contribution of Glacier Runoff during Heat Waves in the Nooksack River Basin USA
8. Watershed Classification Predicts Streamflow Regime and Organic Carbon Dynamics in the Northeast Pacific Coastal Temperate Rainforest
9. The role of large, glaciated tributaries in cooling an important Pacific salmon migration corridor: a study of the Babine River
10. Combined Effects of Summer Water Temperature and Current Velocity on the Distribution of a Cold-Water-Adapted Sculpin (Cottus nozawae)