The value of paleolimnology in reconstructing and managing ecosystem vulnerability: a systematic map
Abstract
Vulnerability can measure an ecosystem’s susceptibility to change as a result of pressure or disturbance, but can be difficult to quantify. Reconstructions of past climate using paleolimnological methods can create a baseline to calibrate future projections of vulnerability, which can improve ecosystem management and conservation plans. Here, we conduct a systematic map to analyze the range and extent that paleolimnological published studies incorporated the concept of vulnerability. Additional themes of monitoring, management, conservation, restoration, or ecological integrity were also included. A total of 52 relevant unique articles were found, a majority of which were conducted in Europe or North America since 2011. Common themes identified included management and adaptation, with the latter heavily focussed on climate change or disturbance. From this, we can infer that the use of paleolimnology to discuss the concept of vulnerability is an emerging field. We argue that paleolimnology plays a valid role in the reconstruction of ecosystem vulnerability due to its capacity to broaden the scope of long-term monitoring, as well as its potential to help establish management and restoration plans. The use of paleolimnology in vulnerability analysis will provide a clearer lens of changes over time; therefore, it should be frequently implemented as a tool for vulnerability assessment.
Introduction
Examining climate variability and ecosystem responses of the past can help describe an ecosystem’s capacity for change. Decisions about conservation, restoration, and often remediation, cannot be effective unless a clear understanding of the cause of the issue exists (Vos et al. 2000). Environmental monitoring aims to measure certain variables over time and space, and can be recognized as a tool for early warning and control (Vos et al. 2000). As such, monitoring informs policy makers with the necessary information for designing and implementing effective environmental policy (Lovett et al. 2007), as well as for managing vulnerability and the conditions that cause it (Luers 2005). Here lies an inherent problem, understanding the context of vulnerability and elucidating the factors that make a system susceptible is essential for a decision-makers ability to prepare and adapt to change (Ford and Smit 2004). However, “vulnerability” is not easily quantifiable and is used in a variety of contexts, such as describing conditions in social, economic, or environmental settings.
Vulnerability can be discussed on multiple scales, for example, the fragility of urban water infrastructures facing the impacts of climate change addresses social (those that rely on the infrastructures), economic (potential costs due to damages), and environmental (detrimental impacts to the resource) vulnerabilities (Dong et al. 2020). Thus, vulnerability as a measure can only be considered as a result of a complex set of factors, each requiring multiple parameters to define and quantify (Aven 2010). As a core concept, vulnerability has been defined as the potential for loss (Adger 2006), but the determination of vulnerability in a system contains components of risk and resilience (Aven 2010) as well as exposure, sensitivity, and the capacity to adapt to changes (Ford et al. 2007). While these descriptions are applied to general discussion of vulnerability, they are especially relevant in the context of ecosystems (Weißhuhn et al. 2018).
Defining the state of an ecosystem can partly be done through the lens of monitoring ecological integrity, which evaluates the extent that an ecosystem has comparable diversity and function of other similar local natural ecosystems, and an ability to maintain and support these ecological processes (Karr and Dudley 1981). Ecological integrity demonstrates self-organization of an ecosystem and can include elements of biotic structures and changes in energy, hydrological, and chemical budgets (Müller et al. 2009). The concept of integrity inherently implies an adaptive capacity to support and maintain the balance and trajectory of the system (Karr 1996), and understanding factors that contribute to ecological integrity, such as responses and resilience to change, can help determine the system’s ability to adapt to change or to disturbance (Luers 2005). In an ecological context, adaptation refers to a process or action that allows the ecosystem to adjust or cope with stress (Smit and Wandel 2006), and adaptive capacity is considered the extent of an ecosystem’s functional ability to be able to respond and reduce risk (Ford and Smit 2004). Being capable of adapting can greatly affect resilience to external pressures, such as anthropogenic stressors that could lead to collapse (Perga et al. 2015), making this an important factor in determining ecosystem vulnerability.
Vulnerability assessments help to identify the ecosystem components facing the biggest threat and the extent of that exposure (Luers 2005) and are generally not a one-size-fits-all approach. Though vulnerability assessments are historically most applied to a social context, ecological vulnerability assessments, which categorize risk, have gained more traction in recent decades (De Lange et al. 2010). The determination of risk can vary based on the scope of assessments for ecological vulnerability. For example, in the case of chemical contamination, data on the toxicity of chemicals released to the environment can provide a “yes” or “no” answer to whether risk exists for a general non-site-specific assessment; whereas a site-specific assessment requires characterization of a system’s specific biological and ecological properties (such as structure or sensitivity) for more precise quantification of risk (De Lange et al. 2010). However, these assessments are rarely spatially explicit (Lee et al. 2018) and often require more baseline information for an in-depth understanding of site-specific vulnerability. To improve understanding of specific ecosystems, long-term historical data can offer a well-rounded representation of the environment and demonstrate its natural history, providing target conditions and offering insight into potential effective management practices (Smol 1992). However, many ecosystems are missing long-term data; therefore, they are missing historical context on how the ecosystem has functioned and experienced changes (Smol 1992).
Regular monitoring usually does not occur over a long enough time period to provide reference (predisturbance) conditions (Norberg et al. 2008), meaning that an external method is often required to collect missing long-term monitoring data. When predisturbance data are missing (which is often the case), paleolimnology can fill this gap (Walker et al. 1991). Paleolimnology is a science that joins the study of lacustrine sediments with the historic and prehistoric ecological context (Smol 1992), offering a range of quantitative data over a calibrated time scale that is otherwise difficult to collect (Walker 2001). The collection of aquatic, terrestrial, and atmospheric deposition in lakes preserves the context of surrounding environment in deposited sediments over time (Williamson et al. 2009), meaning lakes can act as indices for climate variability (Saulnier-Talbot 2016). This is particularly true for closed lakes, which have defined boundaries that control the flow of inputs and outputs (Fergusson and Bangerter 2015). These boundaries allow lakes to preserve proxy records, making lake dynamics such as water level and chemistry highly responsive to climatic changes (Sullivan and Charles 1994; Walker 2001). Lakes are also especially vulnerable to climate change because of the limited capacity for species to relocate due to habitat fragmentation, water density dependence on ambient temperature, and high exposure to human activity (Woodward et al. 2010), making them good sites for analyzing impacts. Using this recording of long-term trends preserved in lakes quantifies past ecosystem changes—a valuable insight for projecting future scenarios (Fordham et al. 2020).
To gain a comprehensive understanding of the value of paleolimnological practices in assessing vulnerability in ecosystems, it is prudent to understand the extent and reach of current research. Here, we conduct a literature search using a systematic mapping approach to analyze the extent of peer-reviewed articles where paleolimnology has been applied in relation to vulnerability as well as review the value of paleolimnological practices in vulnerability assessment. This will help to highlight how paleolimnology can be used to reconstruct ecosystem vulnerability and inform decision-makers. By identifying examples of the use of paleolimnology in monitoring, management, and restoration in the literature, we argue that paleolimnological practice has a rightful place in analyzing vulnerability and ecological integrity. Paleolimnology provides an important comprehensive perspective on ecosystem change and what work needs to be done, so that management needs can be fulfilled.
Methods
To understand the extent of paleolimnological methods used in vulnerability assessments, a systematic map protocol was used to identify, select, and synthesize articles using replicable methods (Supplementary Material 1). Using the ROSES format (Haddaway et al. 2017), the trends in the extent and range of published literature was mapped in broad topic areas to identify gaps in research and clarify the types of approaches that paleolimnological methods are using within a larger context of vulnerability and ecological integrity. Our review included three steps: (i) identifying the broad research realm of paleolimnology and vulnerability assessment through a key word search, (ii) systematically identifying and selecting key research articles that captured the themes identified, and (iii) extracting and summarizing the results to identify key knowledge gaps. For this study, the aim was to include as many relevant articles as possible in our initial key word search to be able to gather a broad scope of studies in the field of paleolimnology including content related to the desired topics.
Search strategy
Based on our areas of interest and main topics, a search was chosen as follows (Boolean logic shown in Table 1, Supplementary Material 2); pal*eolimnolog*, vulnerability, monitor*, manag*, conserve*, restor*, and ecological integrity (* to allow for variations of spelling and use of the root words). Since the use of three databases improves the comprehensiveness of the search, we examined the Boolean logic criteria in combination with each other to search three different online databases; Web of Science, Scopus, and PubMed (the terms paleolimnolog* OR palaeolimnolog* were used for PubMed). Web of Science and Scopus were set to search for these terms in the titles, key words, and abstracts of the articles to avoid results that only mention the words in references or briefly throughout the paper. PubMed does not provide this option, as such all fields were searched and more thoroughly examined for relevant content. The initial search was conducted on 11 July 2020 and updated on 1 August 2020. The searches were restricted to articles published in the present and previous century (1 January 1900, to the date the search took place). A full list of articles found, including those that met our inclusion criteria and those that were excluded from analysis, is included as Supplementary Material 1.
Table 1.
Words (“Paleolimnolog* OR Palaeolimnolog*” used in PubMed searches) | Web of science | Scopus | PubMed |
---|---|---|---|
Pal*eolimnolog* AND vulnerability | 16 | 19 | 15 |
Pal*eolimnolog* AND vulnerability AND monitor* | 3 | 5 | 2 |
Pal*eolimnolog* AND vulnerability AND manag* | 6 | 8 | 5 |
Pal*eolimnolog* AND vulnerability AND conserv* | 0 | 1 | 4 |
Pal*eolimnolog* AND vulnerability AND restor* | 3 | 2 | 1 |
Pal*eolimnolog* AND ecological integrity | 9 | 9 | 6 |
Pal*eolimnolog* AND ecological integrity AND manag* | 2 | 3 | 2 |
Note
A total of 52 unique articles are included.
Inclusion criteria
Inclusion criteria were developed to validate the relevance of the search results. Articles were considered relevant if they were studies using methods in paleolimnology. Studies that discussed similar terms such as paleoclimatology, paleoecology, or paleoenvironmental reconstructions were considered applicable as these terms are often used in similar or applicable contexts. It was important for studies to pertain to vulnerability or ecological integrity in some way by including monitoring, management, conservation, or restoration with a paleolimnological context. Articles that reviewed paleolimnology were noted but not included, and articles that mentioned paleolimnology but did not include it as part of a study were not included. Only articles published in English were included.
Article selection process
To select relevant articles, the titles and abstracts of each article from the results were screened for key words and overall inclusion of paleolimnology and vulnerability/ecological integrity, deciphering if it captured the identified themes as outlined (Table 1). If the inclusion of paleolimnology or vulnerability/ecological integrity was not clear from the title or abstract, then the full article was screened for key terms of interest to determine the context that the search terms were included. Articles that were found to fit the inclusion criteria and contained content relevant to paleolimnology and vulnerability/ecological integrity were included in the data as existing literature on the topics. The initial search and data extraction were done by VW and the consistency of decisions was checked by AM. One paper by one of the authors of this paper was found, but it was excluded as it was not relevant to vulnerability (see Supplementary Material 2).
Analysis
Our analysis focussed on understanding the ways that paleolimnology has been applied to analyzing different aspects of environmental vulnerability as well as mapping the range of published literature and identifying gaps in the research regarding these areas. After articles were narrowed down to those containing relevant information for this research based on the inclusion criteria and selection process, we also examined the inclusion of capacity and adaptation in the literature. While the terms are often used together for the concept of “adaptive capacity”, they are also often seen separately in the literature. Searching them separately allowed us to analyze the different contexts they were used (e.g., resilience capacity vs. carrying capacity), which were relevant to the concept of vulnerability. The inclusion of these terms allowed for an understanding of the extent that capacity and adaptation are discussed in papers regarding paleolimnology and vulnerability, and the proportion in comparison to the total of papers from initial results.
Metadata were extracted from each publication, which included the year of publication, region of the study, key words that each publication identified (if they identified key words), and thematic topics. The papers were read through and organized based on the search terms they were found under—the inclusion of vulnerability, monitoring, management, conservation, or restoration in reference to applying paleolimnology. Some papers were relevant in more than one topic and were placed in multiple categories with appropriate notes. Organizing articles based on content helped determine the amount of literature that is available on these topics. Although this is not a fully complete collection of papers that discuss these areas of research, it can help determine the reach of each thematic area in the literature. The articles were organized based on the systematic search process, as well as additional relevant background, and they are discussed based on the role of paleolimnology in vulnerability assessment, as well as four subtopics (ecological integrity, reference conditions and ecosystem monitoring, establishing management goals, and creating targets for ecosystem restoration) that contribute to the discussion of paleolimnological application.
Results
A total of 52 unique peer-reviewed primary research articles were found (Table 1). Among all search criteria, 25 articles were selected from Web of Science (4 unique), 28 from Scopus (7 unique), and 21 from PubMed (20 unique). One article overlapped all three databases, and 20 articles overlapped Web of Science and Scopus. Some articles also resulted in more than one combination of key words and were identified in more than one Boolean search process (e.g., counted as results for Pal*eolimnolog AND vulnerability AND monitor* as well as Pal*eolimnolog* AND vulnerability AND manag*) but were only counted once in the total of unique papers. The 5 most popular key words used throughout the 52 articles were “paleolimnology”, “lakes”, “diatoms”, “climate change”, and “Holocene” (Fig. 1). Forty-four of the 52 articles, just over 84%, were published in the last decade (between 2011 and 2020).
Fig. 1.

To what extent is capacity covered in the paleolimnology and vulnerability literature?
Out of the 52 unique articles that meet our inclusion criteria, only three articles utilized the term “capacity” within the context of vulnerability; the articles were found to specifically discuss an ecosystem’s capacity for change (Buckley et al. 2010; Mallen-Cooper and Zampatti 2018), and (or) capacity for disturbance (Buckley et al. 2010; Harvey et al. 2019), which are all elements of ecosystem sensitivity and vulnerability. These three papers also include the key word “management” (Fig. 1).
To what extent is adaptation covered in the paleolimnology and vulnerability literature?
Out of the 52 articles, 15 were found that consider adaptation in a vulnerability context, all of which also discuss disturbance or climate change (Table 2). Out of these 15, just over 73%, were published in 2015 or later (Fig. 2A). Sixteen of the 52 studies took place in North America (approx. 31%), and five of these consider adaptation. Eighteen of the 52 studies took place in Europe (approx. 35%), and four of these consider adaptation. There are no visible trends between the studies that consider adaptation and the location of the study (Fig. 2B).
Table 2.
Articles that consider adaptation | Mentions observed adaptation | Mentions disturbance or climate change | Mentions anthropogenic impact | Mentions ecosystem resilience | Mentions adaptive management |
---|---|---|---|---|---|
Lipid biomarkers in lacustrine sediments of subtropical northeastern Mexico and inferred ecosystem changes during the late Pleistocene and Holocene (Chávez-Lara et al. 2019) | ✓ | ✓ | |||
The Apparent Resilience of the Dry Tropical Forests of the Nicaraguan Region of the Central American Dry Corridor to Variations in Climate Over the Last C. 1200 Years (Harvey et al. 2019) | ✓ | ✓ | ✓ | ||
Management pathways for the floodplain wetlands of the southern Murray–Darling Basin: Lessons from history (Gell et al. 2019) | ✓ | ✓ | ✓ | ||
High-resolution paleolimnology opens new management perspectives for lakes adaptation to climate warming (Perga et al. 2015) | ✓ | ✓ | ✓ | ||
Assessment of drought over the past two millennia using near-shore sediment cores from a Canadian boreal lake (Haig et al. 2013) | ✓ | ✓ | |||
Predictability of the impact of multiple stressors on the keystone species Daphnia (Cambronero et al. 2018) | ✓ | ✓ | |||
Cultural implications of late Holocene climate change in the Cuenca Oriental, Mexico (Bhattacharya et al. 2015) | ✓ | ✓ | ✓ | ||
The structure and diversity of freshwater diatom assemblages from Franz Josef Land Archipelago: a northern outpost for freshwater diatoms (Pla-Rabés et al. 2016) | ✓ | ✓ | ✓ | ✓ | |
Timing and causes of mid-Holocene mammoth extinction on St. Paul Island, Alaska (Graham et al. 2016) | ✓ | ✓ | |||
Climate as a contributing factor in the demise of Angkor, Cambodia (Buckley et al. 2010) | ✓ | ||||
Back to the future in a petri dish: Origin and impact of resurrected microbes in natural populations (Houwenhuyse et al. 2017) | ✓ | ✓ | ✓ | ||
History, hydrology and hydraulics: Rethinking the ecological management of large rivers (Mallen-Cooper and Zampatti 2018) | ✓ | ✓ | ✓ | ✓ | |
Springs of the Alps—Sensitive Ecosystems to Environmental Change: From Biodiversity Assessments to Long-term Studies (Cantonati et al. 2006) | ✓ | ✓ | ✓ | ||
Sedimentary DNA Reveals Cyanobacterial Community Diversity over 200 Years in Two Perialpine Lakes (Monchamp et al. 2016) | ✓ | ✓ | |||
Taxonomy and diversity of Afroalpine Chironomidae (Insecta: Diptera) on Mount Kenya and the Rwenzori mountains, East Africa (Eggermont and Verschuren 2007) | ✓ | ✓ |
Fig. 2.

Discussion
The terms adaptation and capacity are both discussed when defining the concept of vulnerability, and are often used to describe its magnitude in interchangeable ways (Adger 2006; Plummer et al. 2012). Capacity can define the ability of an ecosystem to cope with or adapt to circumstances that make it vulnerable (Füssel and Klein 2006), and absence of the capacity to adapt in an ecosystem can also lead to vulnerability, making adaptation vital to achieve ecosystem recovery whether that is natural or managed (Adger 2006). In our systematic mapping process, adaptation was found to be used much more frequently than capacity. All of the articles identified in our search that contained a discussion of the concept of adaptation referenced it in the context of disturbance(s) or climate change (Table 2). This is intuitive as methods in paleolimnology are often used to analyze environmental change and (or) environmental disturbance (Burge et al. 2017). Only 18 articles considered either adaptation or capacity in a vulnerability context. Although the low number of papers published that consider these could be due to a variation in key words, it is more likely that these contexts are only recently being investigated with approaches that use paleolimnology. There is a significant increase in papers that use the terms “paleolimnology” and “vulnerability” after 2010, and the majority of the studies took place in either North America or Europe. We can infer that the use of paleolimnology in the context of discussions of vulnerability is an emerging field, with over 30% of articles in this search having been published in the last 3 years (2018 to July 2020). The terms used for this literature search offered insight into some of the different ways paleolimnology can be applied to assess vulnerability. Here, we discuss the role of paleolimnology in vulnerability assessment as well as four subtopics (ecological integrity, reference conditions and ecosystem monitoring, establishing management goals, and creating targets for ecosystem restoration) that display the validity of paleolimnological application. To keep discussion concise and to avoid repetition, only select articles from the review are integrated.
The role of paleolimnology in vulnerability assessment
The concept of vulnerability is a highly important factor to consider in regards to ecosystem health. Environmental vulnerability specifically analyzes the susceptibility to harm, or the adaptive capacity of, an ecosystem (Adger 2006) and the threshold for change (Luers 2005). Climate change represents a multi-scalar environmental threat, and escalating impacts are heterogeneous across different biomes (NASA 2019). Therefore, ecosystems cannot always be generalized with the use of large-scale assessments and often need to be understood on an individual level. This makes site-specific vulnerability assessments crucial to understanding ecosystem responses and vulnerability on a case-by-case basis. For example, lakes can be treated for the causes of eutrophication (e.g., nutrients), but some eutrophic lakes experience more severe impacts than others (Perga et al. 2015) and must be treated accordingly. Understanding site-specific vulnerability will address the particular needs of the ecosystem such as nutrient control or hydrological regulation, which can lead to defining the best management practices to implement.
Paleolimnology can unlock details of prior ecosystem state and function (Walker et al. 1991), as well as the stressor itself (Korosi et al. 2013), by establishing baseline and trends that can date back hundreds or thousands of years (Smol 1992). As such, paleolimnology can expand the available timeframe and establish the basis for monitoring whereby the scope of current conditions can be compared with historic patterns (Reavie et al. 2006). Sedimentary profiles can provide water quality data as well as harbour physical, chemical, and biological indicators preserved in the sediment that allow for the interpretation of environmental changes (Walker et al. 1991). Some of these include subfossil remains of dipteran aquatic larvae (e.g., chironomids) and siliceous algae (diatoms), as these remains can signify climatic factors that drove their abundance in their specific timeframe (Smol 1992). Observing changes in these compositions across a lake sediment profile can delineate environmental changes such as temperature (e.g., Barley et al. 2006; Langdon et al. 2008; Korosi et al. 2013; McKeown and Potito 2016), nutrient availability (e.g., Reavie et al. 2006; Logan et al. 2011), and disturbances (e.g., Brenner et al. 1993; Quinlan et al. 2008) over time. The analysis of these variables can generate quantitative reconstructions of past climate patterns, define reference conditions of the natural state prior to a disturbance (Norberg et al. 2008), and distinguish the differences between natural variability in the ecosystem’s trajectory from forced changes influenced by external factors (Sullivan and Charles 1994). Policy development concerning the impacts of climate change could greatly benefit from ecosystem vulnerability assessments that include paleolimnology (Füssel and Klein 2006). Indeed, implementing effective management protocols for monitoring can be based on a baseline assessment using paleolimnological methods (Fig. 3). Paleolimnology’s ability to conceptualize the historical impacts of environmental change on independent proxies of an ecosystem as well as project patterns of future change helps create an understanding of an ecosystem’s susceptibility and ability to withstand change and, as a result, its vulnerability. This also means that the concepts that contribute to and help understand vulnerability are fundamentally connected to the concepts that play a role in lake health (Fig. 4).
Fig. 3.

Fig. 4.

Ecological integrity
The components of an ecosystem that are impacted by vulnerability include adaptive capacity, the ability to maintain ecological function, and diversity (Smit and Wandel 2006), which are all elements that make up the concept of ecological integrity (Karr and Dudley 1981). Paleolimnology has historically been used to offer insights into the trajectory of an ecosystem (Smol 1992), helping outline historical changes in community and maintenance of ecosystem function (Gregory-Eaves and Beisner 2011), integral to determining ecological integrity. Although integrity is considered a subjective concept (Wicklum and Davies 1995), it is helpful for determining susceptibility to environmental change (Luers 2005). Eggermont and Verschuren (2007) used an approach that considered ecological integrity to reconstruct changes in productivity due to glacial retreat. They concluded that paleolimnology could be useful for evaluating changes in ecological integrity and anthropogenic climate change (Eggermont and Verschuren 2007). Mallen-Cooper and Zampatti (2018) evaluated the ecological integrity of the Murray River in Australia using paleolimnological evidence to understand changes in hydrology on the site. Knowledge of historical conditions reconstructed through paleolimnological evidence was combined with models that incorporated spatial, temporal, and hydrodynamic information to project future changes and improve management plans for the site (Mallen-Cooper and Zampatti 2018), outlining the value of defining ecological integrity.
Ecological vulnerability assessment can help better understand the various factors that impact ecological integrity, and therefore understanding vulnerability can help decision-makers make informed choices regarding environmental management (He et al. 2018). Identifying areas of vulnerability and disturbance can point towards the stressors themselves, which can impact lake management decisions and goals to mitigate problems effectually (Brenner et al. 1993). For example, excess phosphorus in an aquatic ecosystem acts as the disturbance agent and agricultural runoff can act as the source, resulting in decisions to reduce runoff. The detailed reconstructions offered through monitoring and supplementary paleolimnological data can clearly define ecosystem vulnerability (Smol 1992), providing the stepping stone towards establishing environmental management goals (Luers 2005). A broad scope of natural variability over time is the best way to understand the natural trajectory of change and state at which the ecosystem functions best, which will undoubtedly determine the improvements needed and enhance ecological integrity. This can help avoid uninformed, short-sighted decisions that can result in irreversible mistakes with unintended consequences (Frissell and Bayles 1996).
Reference conditions and ecosystem monitoring
Monitoring often precedes decisions about conservation, restoration, remediation, or other management implementation because it can lead to a clear understanding of change over time (Vos et al. 2000). Regular monitoring does not usually occur over a long enough time period to provide reference (predisturbance) conditions so paleolimnological practices are utilized to supplement gaps in long-term monitoring data (Norberg et al. 2008). Logan et al. (2011) used paleolimnology to assess nutrient statuses of rivers in Australia to compensate for the lack of long-term data. This study analyzed diatoms and stable isotopes from cores and was able to show fluctuations in normally oligotrophic estuaries that are now becoming increasingly eutrophic likely as a result of changing climate. Lami et al. (2010) compared paleolimnological data with monitoring data available from annual limnological surveys. The paleolimnological data were able to demonstrate ecosystem responses to changing climate over time through varying algal assemblages and productivity over the last 3500 years as well as complement the known variations in the Himalayas from the available long-term data. Paleolimnological data can also help differentiate driving forces in aquatic ecosystem changes, such as where Summers et al. (2016) determined if the observed changes in lake productivity were climate change driven or industry driven in lakes near the Athabasca oil sands region. The study found that these specific changes were driven by climate warming, showing that paleolimnology can be used to differentiate impacts from simultaneous disturbances and fill gaps in monitoring information. These studies validate the efficacy of paleolimnological-based data as supplemental monitoring.
Human activity plays a unique role in ecosystem function, driving changes outside the natural range of variability (Battarbee and Bennion 2011), which can cause distortion and vulnerability in an ecosystem (Norberg et al. 2008; McKeown and Potito 2016). While paleolimnological records can often detect these changes to ecosystems, it is not always easy to distinguish anthropogenic-driven disturbances from natural or random variability (Bennion et al. 2011). Langdon et al. (2004) found that chironomid-based paleolimnology has proven useful for detecting land-use changes and other anthropogenic-driven ecosystem alterations that can affect sediments and chemistry of the aquatic system. Humans were found to have interacted with the site through land-use disturbances, such as deforestation, inducing vulnerability. Langdon et al. (2004) also found that Holocene-era temperatures can be reconstructed in the absence of anthropogenic disturbance interfering with the record.
An important part of quantifying vulnerability is having reliable variables to measure and compare conditions. Change over time could not be detected as easily without dependable indicators (Gorham et al. 2001), making indicators key for reliable monitoring. Paleoecological indicators include preserved physical, chemical, and biological information in aquatic sediment such as pollen (e.g., Langdon et al. 2004), diatoms (e.g., Pienitz et al. 1999; Quinlan et al. 2008; Chraïbi and Fritz 2020), Daphnia (e.g., Azan and Arnott 2017), and Chironomidae (e.g., Massaferro and Corley 1998; Langdon et al. 2008; McKeown and Potito 2016), that have all been used as reliable monitoring proxies (Gorham et al. 2001). For example, food web structural variations can be indicated by diatoms, since their different environmental tolerances allow them to be responsive to change (Sculley et al. 2017). Diatom assemblages have also proven useful in Pienitz et al. (1999) as quantitative indicators of environmental change in northern tree-line lakes. Pienitz et al. (1999) determined that the historic limnologic changes do correlate with the regional tree line advancement and retreat, as well as add further evidence that climate warming impacts are exacerbated in high latitude aquatic ecosystems. This was also stressed by Smol (2005), as the lack of monitoring data available as well as the intensified climate change impacts in the Arctic further stress the need for reference conditions and importance of paleolimnological studies in these regions. While we know that anthropogenic stressors exist and are placing high stress on ecosystems, it is impossible to understand the extent and urgency for action without a full scope of the historical changes and the time scale on which they occur (Quinlan et al. 2008). With an expanded timeframe available to create climate reconstructions, paleolimnology can project future changes and determine ecosystem vulnerability, setting up for successful management planning.
Establishing management goals
Land management strategies can be necessary to avoid ecosystem destruction or loss, and thorough understanding of the ecosystem and the components facing the biggest risk are needed to inform these decisions (Lee et al. 2018). Natural resources have irreplaceable value, and management of these resources can help maintain their health. In addition to environmental significance, reduced quality of resources such as freshwater can have many economic and social consequences. For example, pressure on fisheries could impact the economy and the communities that rely on it as well as the general balance of the ecosystem itself (Korosi et al. 2013), providing more incentive for management strategies to be implemented. Frissell and Bayles (1996) emphasized that every person would benefit from resource management and conservation, and that it is not an ecosystem problem that we are facing, but a management problem. Paleolimnology is one tool that can help improve the establishment of management needs and eventual implementation and practice.
Perga et al. (2015) concluded that vulnerability to climate warming varied among different lakes, and these impacts may be minimized with local management strategies to address the lake’s specific needs such as nutrient input, fishery management, or hydrological regulations, that will help promote ecosystem services at each site. Smeltzer and Swain (1985) determined that paleolimnology provided valuable help developing management plans for two Vermont lakes, determining the phytoplankton dominance to be anthropogenically induced, therefore needing restoration. Additionally, filling gaps in the knowledge of long-term data can prevent making assumptions that lake conditions are caused by human interference. Reavie et al. (2006) studied cultural eutrophication in three Southern Ontario lakes using paleolimnology to understand the extent of degradation that has occurred, and they found that one of the lakes was historically more eutrophic and demonstrated naturally higher nutrients and hypolimnetic anoxic conditions prior to human impact. Therefore, the current state of the lake is not a big concern because the conditions are not very different from its natural past, removing the need for management of these conditions. Vermaire et al. (2013) emphasized the necessity of proper management to protect northern communities and the ecosystems they rely on from storm activity increasing due to climate change.
Paleolimnology can also offer information regarding species and biodiversity changes, offering unique perspectives of drivers of change over time leading to management propositions (Gregory-Eaves and Beisner 2011). Recovering historical data can answer questions about species ranges and habitats, such as in the case of the yellow perch (Perca flavescens) by Stager et al. (2015). Although it was believed to have been an invasive species in lakes of northern New York state, DNA preserved in paleolimnological record showed that the yellow perch is actually a native species, appearing in the sedimentary record of the region for over the past two millennia, which changed the conservation strategies for these lakes. Similarly, nitrogen isotopes were used as indicators by Finney et al. (2000), to represent changes in sockeye salmon abundance over the last 300 years, showing that changes in climate have impacted salmon returns and, therefore, lake productivity from salmon carcass nutrient input. This highlights the importance for salmon stock management for this multi-value species. Thus, productive management practices can be put in place with the help of the enhanced understanding of ecosystem needs provided by paleolimnological methods and quantified vulnerability.
Creating targets for ecosystem restoration
The current linkages between paleo-based science and conservation are weak in both literature and practice (Birks 2012). Restoration ecology aims to return the ecosystem to the state in which it operates best, which is often considered “predisturbance” or as the state prior to cultural interference (Bennion et al. 2011). To restore to a previous state, the structure and function of the predisturbance state must be understood to make sure the targets are both desirable and achievable in the context of an ecosystem’s natural trajectory (Roberts et al. 2019). When long-term data are missing, paleolimnological data can help define the predisturbance reference conditions that can act as targets for restoration attempts (Brenner et al. 1993), which can help lead to successful aquatic ecosystem management and restoration (Roberts et al. 2019). Reference conditions provide the standard of the ecosystem state without human-driven changes, as well as offer knowledge of past ecosystem functions. This helps create evidence-based goals for restoration as well as an idea of potential responses to management (Roberts et al. 2019), making paleolimnology a valuable asset for restoration plans.
Paleolimnology can be utilized for establishing restoration targets that revolve around the concept of aiming for desirable, predisturbance conditions. Brenner et al. (1993) used paleolimnological methods to define predisturbance conditions for lakes in Florida to set restoration targets for nutrient and Chl a levels, and apply these targets towards successful restoration of the sites. Norberg et al. (2008) required the use of paleolimnological monitoring data to explain the cause and extent of lake acidification, and whether it is natural or human-induced, as monitoring data are needed to implement appropriate liming restoration efforts. This supplemented missing long-term monitoring data and provided evidence of pre-acidic conditions that acted as targets for restoring the lakes (Norberg et al. 2008). Gell et al. (2019) also used paleolimnology to determine how resilient a floodplain was prior to human disturbance for restoration goals the Murray–Darling Basin (Southeastern Australia). However, many factors were found that also contributed to the initial degradation of the ecosystem, including natural changes. This highlights the importance of not only establishing restoration goals, but understanding the multi-faceted mechanism of change through inferences of multiple responsive parameters.
One of the major obstacles with restoration is some environmental changes are difficult to reverse. Bennion et al. (2011) concluded that when defining restoration targets through reference conditions, it is important to consider the other ecosystem changes that may have occurred between the time of the desired ecosystem state and the current state. Therefore, reference conditions defined by paleolimnology should be considered a “benchmark” rather than a target to leave room for improved conclusion of ecosystem structure (Bennion et al. 2011). Yasuhara et al. (2012) completed a meta-analysis to demonstrate that few sites return to the predegraded state from the degraded state, and even fewer experience full recovery. Yasuhara et al. (2012) also noted the challenge that the predisturbance or pre-industrial trajectories have higher diversity, making returning to the predisturbance state more difficult. For the cases that do experience recovery, it always occurs after initiation of restoration efforts and environmental regulations, proving the value of implementing these actions (Yasuhara et al. 2012). Though full ecosystem restoration is difficult to achieve, paleolimnology can still help work towards ecosystem recovery and reduce overall vulnerability.
Conclusion
Paleolimnological methods can be a useful tool for reconstructing environmental vulnerability. Our systematic mapping process established that 84% of articles included were published in the last decade, and just over 30% of articles in this search were published in the last 3 years at the time of our search. This seems to indicate that including paleolimnology in the discussion of vulnerability is an emerging topic, and it is proven in the literature to have a valid role in the reconstruction of ecosystem vulnerability because of its capacity to broaden the scope of long-term monitoring, as well as its potential for helping establish management and restoration goals.
The multi-faceted science is an integral priming step for establishing reference conditions so that decision-makers can move forward with management plans and conservation goals. However, Gillson and Marchant (2014) showed that paleolimnological tools and data lack a transparency and are not always applicable or user-friendly for stakeholders or policy-makers, preventing it from being used more commonly in conservation sciences. Even when considering conservation or restoration practices, it is not stable or realistic to plan to prevent disturbance from occurring in a dynamic system. Therefore, full understanding of a system requires integrating available facets of information in a multi-scalar perspective such as historical ecology, long-term monitoring, etc., in combination with paleolimnology (Gillson and Marchant 2014). Steps to manage vulnerability need to be well informed, and paleolimnology should be applied often in studies due to the range of perspective it can offer. Full knowledge of the ecosystem gained through paleolimnology can be a key factor in establishing management, but it can only be effective if it is implemented successfully and maintained over time (Frissell and Bayles 1996).
Ecosystem monitoring can also be improved by conceptualizing environmental change that has occurred over time as well as creating benchmarks that help with implementing ecosystem management and restoration efforts. Paleolimnology can define susceptibility and thresholds for change and, as a result, its vulnerability. Understanding the insight that paleolimnology can provide for ecosystem vulnerability can promote its value and place in ecosystem decision-making. Based on our research and the literature we have reviewed, we believe that paleolimnological practice has a rightful place in analyzing vulnerability and ecological integrity.
Acknowledgements
This project was funded by the Fathom Fund of the Marine Environmental Observation Prediction and Response Network (MEOPAR), and by direct donations by the public through projectDAL (Dalhousie University). We would like to thank Sonia Wesche for discussions on the context of vulnerability in environmental assessment. We thank the editor and reviewers for their comments that improved the format and delivery of this manuscript.
References
Adger WN. 2006. Vulnerability. Global Environmental Change, 16: 268–281.
Aven T. 2010. On some recent definitions and analysis frameworks for risk, vulnerability, and resilience. Risk Analysis, 31: 515–522.
Azan S, and Arnott SE. 2017. The impact of calcium decline on population growth rates of crustacea zooplankton in Canadian Shield lakes. Limnology and Oceanography, 63: 602–616.
Barley EM, Walker IR, Kurek J, Cwynar LC, Mathewes RW, Gajewski K, et al. 2006. A northwest North American training set: distribution of freshwater midges in relation to air temperature and lake depth. Journal of Paleolimnology, 36: 295–314.
Battarbee RW, and Bennion H. 2011. Paleolimnology and its developing role in assessing the history and extent of human impact on lake ecosystems. Journal of Paleolimnology, 45: 399–404.
Bennion H, Battarbee RW, Sayer CD, Simpson GL, and Davidson TA. 2011. Defining reference conditions and restoration targets for lake ecosystems using palaeolimnology: a synthesis. Journal of Paleolimnology, 45: 533–544.
Bhattacharya T, Byrne R, Böhnel H, Wogau K, Kienel U, Ingram BL, et al. 2015. Cultural implications of late Holocene climate change in the Cuenca Oriental, Mexico. Proceedings of the National Academy of Sciences of the United States of America, 112: 1693–1698.
Birks HJB. 2012. Ecological palaeoecology and conservation biology: controversies, challenges, and compromises. International Journal of Biodiversity Science, Ecosystem Services & Management, 8: 292–304.
Brenner M, Whitmore TJ, Flannery MS, and Binford MW. 1993. Paleolimnological methods for defining target conditions in lake restoration: Florida case studies. Lake and Reservoir Management, 7: 209–217.
Buckley BM, Anchukaitis KJ, Penny D, Fletcher R, Cook ER, Sano M, et al. 2010. Climate as a contributing factor in the demise of Angkor, Cambodia. Proceedings of the National Academy of Sciences of the United States of America, 107: 6748–6752.
Burge DRL, Edlund MB, and Frisch D. 2017. Paleolimnology and resurrection ecology: the future of reconstructing the past. Evolutionary Applications, 11: 42–59.
Cambronero MC, Marshall H, Meester LD, Davidson TA, Beckerman AP, and Orsini L. 2018. Predictability of the impact of multiple stressors on the keystone species Daphnia. Scientific Reports, 8: 17572.
Cantonati M, Gerecke R, and Bertuzzi E. 2006. Springs of the Alps—sensitive ecosystems to environmental change: from biodiversity assessments to long-term studies. Hydrobiologia, 562: 59–96.
Chávez-Lara C, Holtvoeth J, Roy P, and Pancost R. 2019. Lipid biomarkers in lacustrine sediments of subtropical northeastern Mexico and inferred ecosystem changes during the late Pleistocene and Holocene. Palaeogeography, Palaeoclimatology, Palaeoecology, 535: 109343.
Chraïbi VLS, and Fritz SC. 2020. Assessing the hierarchy of long-term environmental controls on diatom communities of Yellowstone National Park using lacustrine sediment records. Lake and Reservoir Management, 36: 278–296.
De Lange HJ, Sala S, Vighi M, and Faber JH. 2010. Ecological vulnerability in risk assessment—a review and perspectives. Science of the Total Environment, 408: 3871–879.
Dong X, Jiang L, Zeng S, Guo R, and Zeng Y. 2020. Vulnerability of urban water infrastructures to climate change at city level. Resources, Conservation and Recycling, 161: 104918.
Eggermont H, and Verschuren D. 2007. Taxonomy and diversity of Afroalpine Chironomidae (Insecta: Diptera) on Mount Kenya and the Rwenzori Mountains, East Africa. Journal of Biogeography, 34: 69–89.
Fergusson L, and Bangerter PJ. 2015. Principles of environmental remediation in open and closed systems: a case study of the Lake Dianchi drainage basin. International Journal of Engineering Sciences & Research Technology, 4: 1–16.
Finney BP. 2000. Impacts of climatic change and fishing on Pacific salmon abundance over the past 300 years. Science, 290: 795–799.
Ford JD, and Smit B. 2004. A framework for the vulnerability of communities Arctic assessing to risks associated with climate change. Arctic, 57: 389–400.
Ford JD, Pearce T, Smit B, Wandel J, Allurut M, Ittasarjuat H, et al. 2007. Reducing vulnerability to climate change in the Arctic: the case of Nunavut, Canada. Arctic, 60: 150–166.
Fordham DA, Jackson ST, Brown SC, Huntley B, Brook BW, Dahl-Jensen D, et al. 2020. Using paleo-archives to safeguard biodiversity under climate change. Science, 369: eabc5654.
Frissell CA, and Bayles D. 1996. Ecosystem management and the conservation of aquatic biodiversity and ecological integrity. Journal of the American Water Resources Association, 32: 229–240.
Füssel HM, and Klein RJT. 2006. Climate change vulnerability assessments: an evolution of conceptual thinking. Climatic Change, 75: 301–329.
Gell PA, Reid MA, and Wilby RL. 2019. Management pathways for the floodplain wetlands of the southern Murray–Darling Basin: lessons from history. River Research and Applications, 35: 1291–1301.
Gillson L, and Marchant R. 2014. From myopia to clarity: sharpening the focus of ecosystem management through the lens of palaeoecology. Trends in Ecology & Evolution, 29: 317–325.
Gorham E, Brush GS, Graumlich LJ, Rosenzweig ML, and Johnson AH. 2001. The value of paleoecology as an aid to monitoring ecosystems and landscapes, chiefly with reference to North America. Environmental Reviews, 9: 99–126.
Graham RW, Belmecheri S, Choy K, Culleton BJ, Davies LJ, Froese D, et al. 2016. Timing and causes of mid-Holocene mammoth extinction on St. Paul Island, Alaska. Proceedings of the National Academy of Sciences of the United States of America, 113: 9310–9314.
Gregory-Eaves I, and Beisner BE. 2011. Palaeolimnological insights for biodiversity science: an emerging field. Freshwater Biology, 56: 2653–2661.
Haddaway NR, Macura B, Whaley P, and Pullin AS. 2017. ROSES for systematic map protocols. Version 1.0.
Haig HA, Kingsbury MV, Laird KR, Leavitt PR, Laing R, and Cumming BF. 2013. Assessment of drought over the past two millennia using near-shore sediment cores from a Canadian boreal lake. Journal of Paleolimnology, 50: 175–190.
Harvey WJ, Stansell N, Nogué S, and Willis KJ. 2019. The apparent resilience of the dry tropical forests of the Nicaraguan region of the Central American dry corridor to variations in climate over the last c. 1200 years. Quaternary, 2: 25.
He L, Shen J, and Zhang Y. 2018. Ecological vulnerability assessment for ecological conservation and environmental management. Journal of Environmental Management, 206: 1115–1125.
Houwenhuyse S, Macke E, Reyserhove L, Bulteel L, and Decaestecker E. 2017. Back to the future in a petri dish: origin and impact of resurrected microbes in natural populations. Evolutionary Applications, 11: 29–41.
Karr JR. 1996. Ecological integrity and ecological health are not the same. In Engineering within ecological constraints. Edited by P Schuze. National Academy of Sciences. pp. 97–109.
Karr JR, and Dudley DR. 1981. Ecological perspective on water quality goals. Environmental Management, 5: 55–68.
Korosi JB, Ginn BK, Cumming BF, and Smol JP. 2013. Establishing past environmental conditions and tracking long-term environmental change in the Canadian Maritime provinces using lake sediments. Environmental Reviews, 21: 15–27.
Lami A, Marchetto A, Musazzi S, Salerno F, Tartari G, Guilizzoni P, et al. 2010. Chemical and biological response of two small lakes in the Khumbu Valley, Himalayas (Nepal) to short-term variability and climatic change as detected by long-term monitoring and paleolimnological methods. Hydrobiologia, 648: 189–205.
Langdon PG, Barber K, and Morriss SL. 2004. Reconstructing climate and environmental change in northern England through chironomid and pollen analyses: evidence from Talkin Tarn, Cumbria. Journal of Paleolimnology, 32: 197–213.
Langdon PG, Holmes N, and Caseldine CJ. 2008. Environmental controls on modern chironomid faunas from NW Iceland and implications for reconstructing climate change. Journal of Paleolimnology, 40: 273–293.
Lee C, Duncan C, Owen H, and Pettorelli N. 2018. A new framework to assess relative ecosystem vulnerability to climate change. Conservation Letters, 11: e12372.
Logan B, Taffs KH, Eyre BD, and Zawadski A. 2011. Assessing changes in nutrient status in the Richmond River estuary, Australia, using paleolimnological methods. Journal of Paleolimnology, 46: 597–611.
Lovett G, Burns D, Driscoll C, Jenkins J, Mitchell M, Rustad L, et al. 2007. Who needs environmental monitoring? Frontiers in Ecology and the Environment, 5: 253–260.
Luers AL. 2005. The surface of vulnerability: an analytical framework for examining environmental change. Global Environmental Change, 15: 214–223.
Mallen-Cooper M, and Zampatti BP. 2018. History, hydrology and hydraulics: rethinking the ecological management of large rivers. Ecohydrology, 11: e1965.
Massaferro J, and Corley J. 1998. Environmental disturbance and chironomid palaeodiversity: 15 kyr BP of history at Lake Mascardi, Patagonia, Argentina. Aquatic Conservation: Marine and Freshwater Ecosystems, 8: 315–323.
McKeown M, and Potito AP. 2016. Assessing recent climatic and human influences on chironomid communities from two moderately impacted lakes in western Ireland. Hydrobiologia, 765: 245–263.
Monchamp ME, Walser JC, Pomati F, and Spaak P. 2016. Sedimentary DNA reveals cyanobacterial community diversity over 200 years in two perialpine lakes. Applied and Environmental Microbiology, 82: 6472–6482.
Müller F, Burkhard B, and Kroll F. 2009. Resilience, integrity and ecosystem dynamics: bridging ecosystem theory and management. In Landform—structure, evolution, process control. Lecture Notes in Earth Sciences. Vol. 115. Edited by JC Otto and R Dikau. Springer. pp. 221–242.
NASA. 2019. The effects of climate change [online]: Available from climate.nasa.gov/effects.
Norberg M, Bigler C, and Renberg I. 2008. Monitoring compared with paleolimnology: implications for the definition of reference condition in limed lakes in Sweden. Environmental Monitoring and Assessment, 146: 295–308.
Perga M, Frossard V, Jenny J, Alric B, Arnaud F, Berthon V, et al. 2015. High-resolution paleolimnology opens new management perspectives for lakes adaptation to climate warming. Frontiers in Ecology and Evolution, 3: 72.
Pienitz R, Smol JP, and Macdonaldt GM. 1999. Paleolimnological reconstruction of Holocene climatic trends from two boreal treeline lakes, Northwest Territories, Canada. Arctic, Antarctic, and Alpine Research, 31: 82–93.
Pla-Rabés S, Hamilton PB, Ballesteros E, Gavrilo M, Friedlander AM, and Sala E. 2016. The structure and diversity of freshwater diatom assemblages from Franz Josef Land Archipelago: a northern outpost for freshwater diatoms. PeerJ, 4: e1705.
Plummer R, de Loë R, and Armitage D. 2012. A systematic review of water vulnerability assessment tools. Water Resources Management, 26: 4327–4346.
Quinlan R, Hall RI, Paterson AM, Cumming BF, and Smol JP. 2008. Long-term assessments of ecological effects of anthropogenic stressors on aquatic ecosystems from paleoecological analyses: challenges to perspectives of lake management. Canadian Journal of Fisheries and Aquatic Sciences, 65: 933–944.
Reavie ED, Neill KE, Little JL, and Smol JP. 2006. Cultural eutrophication trends in three southeastern Ontario lakes: a paleolimnological perspective. Lake and Reservoir Management, 22: 44–58.
Roberts LR, Sayer CD, Hoare D, Tomlinson M, Holmes JA, Horne DJ, et al. 2019. The role of monitoring, documentary and archival records for coastal shallow lake management. Geo: Geography and Environment, 6: e00083.
Saulnier-Talbot É. 2016. Paleolimnology as a tool to achieve environmental sustainability in the Anthropocene: an overview. Geosciences, 6: 26.
Sculley JB, Lowe RL, Nittrouer CA, Drexler TM, and Power ME. 2017. Eighty years of food-web response to interannual variation in discharge recorded in river diatom frustules from an ocean sediment core. Proceedings of the National Academy of Sciences of the United States of America, 114: 10155–10159.
Smeltzer E, and Swain EB. 1985. Answering lake management questions with paleolimnology. Lake and Reservoir Management, 1: 268–274.
Smit B, and Wandel J. 2006. Adaptation, adaptive capacity and vulnerability. Global Environmental Change, 16: 282–292.
Smol JP. 1992. Paleolimnology: an important tool for effective ecosystem management. Journal of Aquatic Ecosystem Health, 1: 49–58.
Smol JP. 2005. Tracking long-term environmental changes in Arctic lakes and ponds: a paleolimnological perspective. Arctic Institute of North America, 58: 227–229.
Stager JC, Sporn LA, Johnson M, and Regalado S. 2015. Of paleo-genes and perch: what if an “alien” is actually a native? PLoS ONE, 10: e0119071.
Sullivan TJ, and Charles DF. 1994. The feasibility and utility of a paleolimnology/paleoclimate data cooperative for North America—report on a NOAA paleoclimate Workshop. Journal of Paleolimnology, 10: 265–273.
Summers JC, Kurek J, Kirk JL, Muir DCG, Wang X, Wiklund JA, et al. 2016. Recent warming, rather than industrial emissions of bioavailable nutrients, is the dominant driver of lake primary production shifts across the Athabasca oil sands region. PLoS ONE, 11: e0153987.
Vermaire JC, Pisaric MF, Thienpont JR, Mustaphi CJ, Kokelj SV, and Smol JP. 2013. Arctic climate warming and sea ice declines lead to increased storm surge activity. Geophysical Research Letters, 40: 1386–1390.
Vos P, Meelis E, and Ter Keurs WJ. 2000. A framework for the design of ecological monitoring programs as a tool for environmental and nature management. Environmental Monitoring and Assessment, 61: 317–344.
Walker IR. 2001. Midges: Chironomidae and related Diptera. In Tracking environmental change using lake sediments. Developments in Paleoenvironmental Research. Vol. 4. Edited by JP Smol, HJB Birks, and WM Last. Springer. pp. 43–66.
Walker IR, Mott RJ, and Smol JP. 1991. Allerød–Younger Dryas lake temperatures from midge fossils in Atlantic Canada. Science, 253: 1010–1012.
Weißhuhn P, Müller F, and Wiggering H. 2018. Ecosystem vulnerability review: proposal of an interdisciplinary ecosystem assessment approach. Environmental Management, 61: 904–915.
White PS, and Pickett STA. 1985. Natural disturbance and patch dynamics: an introduction. In The ecology of natural disturbance and patch dynamics. Edited by STA Pickett and PS White. Academic Press, New York, New York. pp. 3–13.
Wicklum D, and Davies RW. 1995. Ecosystem health and integrity? Canadian Journal of Botany, 73: 997–1000.
Williamson CE, Saros JE, Vincent WF, and Smol JP. 2009. Lakes and reservoirs as sentinels, integrators, and regulators of climate change. Limnology and Oceanography, 54: 2273–2282.
Woodward G, Perkins DM, and Brown LE. 2010. Climate change and freshwater ecosystems: impacts across multiple levels of organization. Philosophical Transactions of the Royal Society, B: Biological Sciences, 365: 2093–2106.
Yasuhara M, Hunt G, Breitburg D, Tsujimoto A, and Katsuki K. 2012. Human-induced marine ecological degradation: micropaleontological perspectives. Ecology and Evolution, 2: 3242–3268.
Supplementary materials
Supplementary Material 1 (XLSX / 118 KB)
- Download
- 118.11 KB
Supplementary Material 2 (PPTX / 41.7 KB)
- Download
- 41.78 KB
Information & Authors
Information
Published In
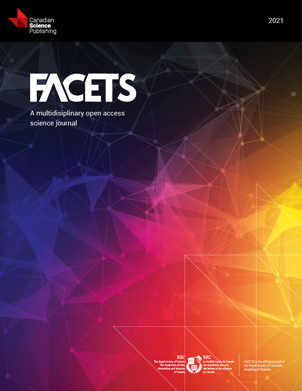
FACETS
Volume 6 • Number 1 • January 2021
Pages: 517 - 536
Editor: Irene Gregory-Eaves
History
Received: 25 August 2020
Accepted: 1 January 2021
Version of record online: 8 April 2021
Copyright
© 2021 Watson and Medeiros. This work is licensed under a Creative Commons Attribution 4.0 International License (CC BY 4.0), which permits unrestricted use, distribution, and reproduction in any medium, provided the original author(s) and source are credited.
Data Availability Statement
All relevant data are within the paper and in the Supplementary Material.
Key Words
Sections
Subjects
Authors
Author Contributions
ASM conceived and designed the study.
VTW performed the experiments/collected the data.
VTW analyzed and interpreted the data.
All contributed resources.
All drafted and revised the manuscript.
Competing Interests
The authors have declared that no competing interests exist.
Metrics & Citations
Metrics
Other Metrics
Citations
Cite As
Victoria Theresa Watson and Andrew Scott Medeiros. 2021. The value of paleolimnology in reconstructing and managing ecosystem vulnerability: a systematic map. FACETS.
6: 517-536.
https://doi.org/10.1139/facets-2020-0067
Export Citations
If you have the appropriate software installed, you can download article citation data to the citation manager of your choice. Simply select your manager software from the list below and click Download.
Cited by
1. Conservation-led palaeolimnology: a review of applied palaeolimnology and lessons to improve accessibility and value to conservation practice
2. Subfossil Cladocera as indicators of pH, trophic state and conductivity: Separate and combined effects in hemi boreal freshwater lakes
3. Conservation-led palaeolimnology – A review of applied palaeolimnology and lessons to improve accessibility and value to conservation practice
4. Revegetation through seeding or planting: A worldwide systematic map
5. Progress in understanding the vulnerability of freshwater ecosystems
6. A paleolimnological context of ecological vulnerability for the freshwater ecosystems of Sable Island National Park Reserve, Canada