Detection of carbapenem-resistance genes in bacteria isolated from wastewater in Ontario
Abstract
Bacterial carbapenem resistance is a major public health concern since these antimicrobials are often the last resort to treat serious human infections. To evaluate methodologies for detection of carbapenem resistance, carbapenem-tolerant bacteria were isolated from wastewater treatment plants in Toronto, Ottawa, and Arnprior, Ontario. A total of 135 carbapenem-tolerant bacteria were recovered. Polymerase chain reaction (PCR) indicated the presence of carbapenem hydrolysing enzymes KPC (n = 10), GES (n = 5), VIM (n = 7), and IMP (n = 1), and β-lactamases TEM (n = 7), PER (n = 1), and OXA-variants (n = 16). A subset of 46 isolates were sequenced and analysed using ResFinder and CARD-RGI. Both programs detected carbapenem resistance genes in 35 sequenced isolates and antimicrobial resistance genes (ARGs) conferring resistance to multiple class of other antibiotics. Where β-lactamase resistance genes were not initially identified, lowering the thresholds for ARG detection enabled identification of closely related β-lactamases. However, no known carbapenem resistance genes were found in seven sequenced Pseudomonas spp. isolates. Also of note was a multi-drug-resistant Klebsiella pneumoniae isolate from Ottawa, which harboured resistance to seven antimicrobial classes including β-lactams. These results highlight the diversity of genes encoding carbapenem resistance in Ontario and the utility of whole genome sequencing over PCR for ARG detection where resistance may result from an assortment of genes.
Graphical Abstract

Introduction
The global increase of antimicrobial resistance (AMR) in clinically relevant microorganisms is becoming critical. AMR genes (ARGs) are of particular concern when encoded by mobile genetic elements such as plasmids and transposons that have been shown to transfer ARGs to pathogenic bacteria (Noble et al. 1992; Davison 1999; von Wintersdorff et al. 2016). The potential for transfer of genetic material within bacterial communities via conjugation and transformation increases the importance of studying and monitoring clinically significant ARGs that may be acquired by pathogenic organisms. Of particular concern is the rise in resistance to last-resort antibiotics, such as carbapenems, in microbial communities (Bush 1999; Mulvey et al. 2003; van Duin and Doi 2017).
Increasing levels of resistance to carbapenem antimicrobials has become a major public health concern because of their importance in human medicine and lack of development of new antimicrobials. Most concerning are infections due to carbapenem-resistant Enterobacteriaceae (CRE) strains that are associated with higher morbidity and mortality rates (Chang et al. 2011; Ben-David et al. 2012; Esterly et al. 2012). Included in this group of bacteria are many species relevant to both the food industry and clinic such as Escherichia coli and Klebsiella spp. CRE have been isolated from healthy human carriers, food-producing animals, and food products around the globe (Nordmann et al. 2011).
Carbapenems belong to the β-lactam class of antimicrobials, a broad-spectrum class of agents that contain a four-membered β-lactam ring. Carbapenems include the antibiotics doripenem, ertapenem, imipenem, and meropenem (Codjoe and Donkor 2018). They are typically reserved for treatment of the most serious infections caused by multi-drug-resistant pathogens as they are not susceptible to common β-lactam resistance mechanisms (Bush 2010; Codjoe and Donkor 2018).
Bacterial resistance to β-lactams typically occurs through the production of β-lactamase enzymes that hydrolyze the β-lactam ring. β-lactamases may be grouped according to protein homology (Ambler molecular classification) and (or) based on hydrolysis and inhibition profiles (Bush–Jacoby–Medeiros functional classification), resulting in derivatives such as penicillinases, cephalosporinases, carbenicillinases, and carbapenemases. Different types of β-lactamases inactivate different β-lactam antimicrobials. The extended spectrum β-lactamases (ESBLs) are a group of enzymes that break down antibiotics of the penicillin and cephalosporin classes (Shaikh et al. 2015). Carbapenemases are a versatile family of the β-lactamases as they are able to hydrolyze almost all hydrolysable β-lactams including penicillins, cephalosporins, and carbapenems (Queenan and Bush 2007). As carbapenemases are β-lactamases, they belong to one of two molecular families distinguished based on the active site. Molecular class A and D molecules contain a serine-based active site, whereas molecular class B, also known as metallo-β-lactamases (MBLs), contain zinc (Queenan and Bush 2007; Bush and Jacoby 2010). Originally carbapenemases were thought to be species-specific chromosomal β-lactamases; however, many of these enzymes including IMP and KPC have subsequently been identified in diverse species on plasmids (Queenan and Bush 2007; Almeida et al. 2013).
A number of β-lactamases and ESBLs belonging to non-carbapenemase classes have also exhibited low-level carbapenem-hydrolyzing activity and can confer carbapenem resistance to some extent (Jacoby 2009; Bonnin et al. 2011). Overall, three major mechanisms resulting in bacterial carbapenem resistance have been described: the production of carbapenemase(s), production of a β-lactamase or ESBL coupled with porin loss or modification, and efflux (Jacoby et al. 2004; Jacoby 2009; Hao et al. 2018).
Phenotypic confirmation of carbapenemase production is based on detection of carbapenemase diffusion or inhibition of carbapenemase activity (Bartolini et al. 2014). Detection of diffusible carbapenemases can be evaluated using the modified Hodge test (MHT); however, interpretation of results is subjective and may include false-positive and false-negative results (Girlich et al. 2012; Pierce et al. 2017; Tamma et al. 2017). Similarly, the Carba NP test suffers from subjective results, poor sensitivity, and the requirement of specialized reagents (Pierce et al. 2017; Tamma et al. 2017). Measuring inhibition of carbapenemase activity is conducted using Ethylenediaminetetraacetic acid (EDTA) and dipicolinic acid against MBLs, boronic acid to inhibit Ambler class A enzymes, and cloxacillin against ampicillinases (Bartolini et al. 2014). Unfortunately most of the available methods to detect carbapenemase production were designed primarily for Enterobacteriaceae, involve time-consuming protocols, are subjective, not very sensitive/specific, and many require specialized reagents (Pierce et al. 2017).
Evidence has shown that human activities facilitate the emergence and dissemination of AMR bacteria in the environment (Li et al. 2015; Freeman et al. 2018). This is of great concern as bacteria, possibly containing ARGs, are capable of persistence and spreading throughout the environment (Anderson et al. 2005). Wastewater treatment plants (WWTPs) are environmental hot spots for ARG dissemination, as these sites typically have extremely high bacterial loads from human sources combined with subinhibitory concentrations of antimicrobials discharged from household, hospital, agricultural, and industrial human sources (Berendonk et al. 2015; Li et al. 2015).
AMR surveillance programs such as the European program DARE (Detecting evolutionary hot spots of Antibiotic Resistance in Europe) have suggested that resistance testing should focus on resistance determinants that are clinically relevant, prevalent in the environment, associated with mobile genetic elements, and have the potential to be acquired by horizontal gene transfer (Berendonk et al. 2015). Candidate genes frequently occurring in various environments impacted by anthropogenic activities include the β-lactamases blaCTX-M, blaTEM, MBL blaNDM-1, and carbapenemases blaKPC and blaVIM (among others) (Jacoby 2009).
Wastewater is increasingly used for surveillance of AMR as it contains an abundance of bacteria derived from anthropogenic sources and elevated levels of ARGs (Li et al. 2015; Hembach et al. 2017; Muurinen et al. 2017; Ng et al. 2017; Freeman et al. 2018; Hutinel et al. 2019; Pärnänen et al. 2019; Aarestrup and Woolhouse 2020; Sims and Kasprzyk-Hordern 2020). Effective surveillance programs require rapid methods capable of processing large numbers of samples. We therefore sought to assess methodologies for detection and enumeration of bacterial carbapenem-resistance genes using sewage influent from different WWTPs, as carbapenems are clinically very important for treatment of difficult infections, and wastewater influent is likely to harbour carbapenem-tolerant bacteria.
Materials and methods
Sampling
Wastewater (influent) samples (1 L) were collected from Arnprior, a small agricultural city (3 March 2017); Ottawa, a mid-sized city with light industry (10 March 2017); and Toronto, a major industrialized city (17 March 2017). Samples were received on ice at the Ottawa Laboratory Carling (Canadian Food Inspection Agency) where they were filtered and plated on MacConkey agar containing meropenem within 24 h of collection (described below).
Microbiological analysis
Carbapenem-resistance in this study was defined as growth on agar containing ≥4 μg/mL meropenem for Enterobacteriaceae, ≥8 μg/mL for Stenotrophomonas maltophilia, and ≥16 μg/mL for other non-Enterobacterales including Pseudomonas spp. (but not P. aeruginosa), as recommended by Clinical and Laboratory Standards Institute (CLSI; 2020) guidelines. Carbapenem-tolerance was defined as growth on media containing 4 μg/mL for S. maltophilia and Pseudomonas spp. (CLSI 2020).
Water analyses were conducted as per the method of Zurfluh et al. (2013). Briefly, 250 mL of each water sample was filtered through a series of 0.80 μm and 0.22 μm sterile membrane filters (S-Pak Membrane Filters, MilliporeSigma, Ottawa, Ontario, Canada). For each sample all filters were aseptically transferred to a single sterile stomacher bag containing 25 mL modified tryptone soya broth (mTSB) (Oxoid, Ottawa, Ontario, Canada). Filters were rinsed by manually massaging stomacher bags for 5 min to release the collected bacterial cells. An aliquot from each filter rinse was serially diluted in sterile phosphate buffered saline, and dilutions were plated in triplicate on MacConkey agar (MAC) (Sigma, Ottawa, Ontario, Canada) containing 4 μg/mL meropenem (USP, Rockville, Maryland, USA) to select for carbapenem-tolerant gram-negative bacteria. Plates were incubated at 37 °C for 24 h followed by storage at 4 °C until further analysis.
Fifty (Toronto), 51 (Ottawa), and 34 (Arnprior) colony forming units were randomly selected from spread plates for purification via restreaking on MAC containing 4 μg/mL meropenem using a sterile loop. Purified isolates were used for further AMR characterization and β-lactamase ARG detection as described below.
Carbapenemase production confirmation
All isolates were tested for carbapenemase-production using the MHT according to CLSI guidelines (CLSI 2011). A 0.5 MacFarland suspension of ATCC 25922 Escherichia coli was diluted 1:10 in saline, and a lawn was spread on Mueller Hinton agar (Thermo Scientific, Oxoid, Ottawa, Ontario, Canada) and left to dry for 3 min. A 10 μg meropenem disc (Thermo Scientific Oxoid, Ottawa, Ontario, Canada) was placed in the centre of the agar plate. Following disc placement, a sterile swab was used to pick three colonies of the purified test organism and was streaked from the edge of the disc to the edge of the agar plate in a straight line. Plates were incubated at 37 °C overnight. If the test organism allowed enhanced growth of the ATCC 25922 lawn towards the disc (into the inhibition zone producing a clover-leaf shape) it was considered to be a carbapenemase producer.
DNA extraction
For each purified isolate, one colony was transferred to 1.2 mL of Mueller-Hinton broth (Thermo Scientific Oxoid, Hants, UK) containing 4 μg/mL meropenem using a sterile loop and incubated at 36 °C overnight. Genomic DNA was isolated from overnight broth cultures using the Promega Maxwell 16 Cell DNA purification kit (Promega, Wisconsin, USA). For each sample, 400 μL of broth culture was added to a Maxwell standard elution volume cartridge and the remaining 800 μL of culture was mixed with an equal volume of 30% glycerol and frozen at −80 °C for long-term storage. Extracted DNA was stored at −20 °C.
Characterization of β-lactamases
Genomic DNA was diluted 1:10 (to a concentration of approximately 1–4 ng/μL) and utilized in PCR reactions as described previously (Bogaerts et al. 2013). Twenty-one primer sets (Integrated DNA Technologies, Illinois, USA) were used to screen for select β-lactamase, ESBL, and carbapenemase resistance genes (Table 1). Primers for the OXA PCR were designed in house as follows: (i) nucleotide sequences for multiple alleles of each gene were downloaded from the Center for Genomic Epidemiology (CGE) ResFinder database (accessible at: bitbucket.org/genomicepidemiology/resfinder_db), (ii) alleles for each gene were aligned and analysed for conserved sequence regions, and (iii) conserved regions were input to National Center for Biotechnology Information (NCBI) Primer-BLAST (Basic Local Alignment Search Tool) to design target-specific primers for use in PCR (Ye et al. 2012). The 25 μL reaction mixtures contained 2 μL of diluted DNA extract, 12.5 μL of 2X TopTaq Mastermix (Qiagen, USA), and 0.2 μM of each primer (with the exception of the CARBA multiplex PCR that contained 0.6 μM of blaIMP primers and 0.2 μM of other primers, Table 1). PCR was performed using a BioRad C1000 Touch Thermal Cycler (BioRad, California, USA) with the following conditions: 15 min at 95 °C, 30 cycles of 30 s denaturation at 94 °C, 90 s annealing at 57 °C, and 90 s elongation at 72 °C, followed by a final elongation at 72 °C for 10 min. PCR amplicons were analysed using capillary electrophoresis on the Qiaxcel DNA Screening system (Qiagen, USA) with QX alignment markers 15/3000 bp, and QX DNA size marker 100–2500 bp.
Table 1.
PCR name | Targeted gene | Primer sequence (5′–3′)a | Amplicon size (bp) | Reference | |
---|---|---|---|---|---|
BactQuantb | 16S | F | CCTACGGGDGGCWGCA | 466 | Liu et al. (2012) |
R | GGACTACHVGGGTMTCTAATC | ||||
CARBA | blaNDM | F | ACTTGGCCTTGCTGTCCTT | 603 | Bogaerts et al. (2013) |
R | CATTAGCCGCTGCATTGAT | ||||
blaVIM | F | TGTCCGTGATGGTGATGAGT | 437 | Bogaerts et al. (2013) | |
R | ATTCAGCCAGATCGGCATC | ||||
blaKPC | F | TCGCCGTCTAGTTCTGCTGTCTTG | 353 | Bogaerts et al. (2013) | |
R | ACAGCTCCGCCACCGTCAT | ||||
blaOXA-48 | F | ATGCGTGTATTAGCCTTATCG | 265 | Bogaerts et al. (2013) | |
R | CATCCTTAACCACGCCCAAATC | ||||
blaIMP | F | ACAYGGYTTRGTDGTKCTTG | 387 | Bogaerts et al. (2013) | |
R | GGTTTAAYAAARCAACCACC | ||||
MINESBL | blaPER | F | AGTGTGGGGGCCTGACGAT | 725 | Bogaerts et al. (2013) |
R | GCAACCTGCGCAATRATAGCTT | ||||
blaGES | F | CTGGCAGGGATCGCTCACTC | 600 | Bogaerts et al. (2013) | |
R | TTCCGATCAGCCACCTCTCA | ||||
OXAc | blaOXA-51 | F | GACTGCACGCCGGAACTC | 156 | This studyb |
R | CAGTATCCCGAGAGCCTTGA | ||||
blaOXA-2 | F | TTTCTCTTGCCACTTTCGCG | 409 | This studyb | |
R | GCGTTGCCATAGTCGATTTTC | ||||
blaOXA-10 | F | GCCGTCAATGGTGTCTTCGT | 544 | This studyb | |
R | TGACTCAGTTCCCACACCAGA | ||||
POM | blaPOM-1 | F | GACAGCCAGGACATCCACTT | 210 | This studyb |
R | GCTCAGGCTGTCGCTGTAG | ||||
TEM-SHV | blaTEM | F | TCGCCGCATACACTATTCTCAGAATGA | 445 | Monstein et al. (2007) |
R | ACGCTCACCGGCTCCAGATTTAT | ||||
blaSHV | F | ATGCGTTATATTCGCCTGTG | 747 | Paterson et al. (2003) | |
R | TGCTTTGTTATTCGGGCCAA | ||||
CTX-M-U | blaCTX-M | F | ATGTGCAGYACCAGTAARGTKATGGC | 593 | Mulvey et al. (2003) |
R | TGGGTRAARTARGTSACCAGAAYCAGCGG |
a
Degenerate bases: Y, is a pyrimidine; R, is a purine; K, is a G or T; S, is a G or C.
b
The BactQuant 16S primers were used as a positive control to ensure bacterial DNA was present in diluted gDNA samples.
c
Alleles for each gene downloaded from Center for Genomic Epidemiology ResFinder database. Nucleotide sequences of alleles were aligned, and NCBI Primer Blast was used to design primers for conserved regions.
Species identification by MALDI-TOF
The species identity of each isolate was determined by matrix-assisted laser desorption/ionization time of flight mass spectrometry (MALDI-TOF). Briefly, colonies were streaked onto tryptic soy agar and incubated overnight at 37 °C. One bacterial colony was applied on duplicate spots of a Biotarget plate, air dried, and overlaid with freshly prepared α-cyano-4-hydroxy-cinnamic acid matrix. Mass spectra were obtained using a MALDI Biotyper mass spectrometer (Bruker Daltonic, Bremen, Germany) and compared with the Biotyper taxonomy database using the MALDI Biotyper 3.1 software. Isolates that received score values of 2.3–3 were identified to the species level. Scores between 2 and 2.299 corresponded to isolates identified to the genus level.
Whole genome sequencing (WGS) and in silico ARG detection
Isolates from a variety of genera with at least one β-lactam resistance gene as determined by PCR were selected for sequencing. A number of PCR-negative isolates were also selected to investigate additional ARGs that may be conferring resistance. From Toronto, Ottawa, and Arnprior 15, 17, and 14, isolates (respectively) were selected for sequencing. Of the selected isolates eight, eight, and one isolate(s) were selected based on PCR-positive results (Toronto, Ottawa, and Arnprior, respectively). The remaining isolates sequenced from each WWTP were PCR-negative. Sequencing libraries were constructed using the Nextera XT DNA sample preparation kit (Illumina, Inc., San Diego, California, USA) and paired-end sequencing was performed on the Illumina MiSeq platform (Illumina Inc.) using 600 cycle MiSeq reagent kits (v3) with 6% PhiX control.
Paired-end Illumina MiSeq sequencing reads were processed with the CFIA-OLC Workflow for Bacterial Assembly and Typing (COWBAT). Source code for COWBAT can be found online: github.com/OLC-Bioinformatics/COWBAT. Quality of raw sequencing reads was assessed with FastQC version 0.11.8 (Andrews 2010). Quality trimming was performed with BBDuk from BBTools version 38.22 (Bushnell 2014) with the following parameters: trim quality of 10 and removal of reads below 50 base pair (bp) long. Error correction was performed using tadpole version 8.22 (Bushnell 2014) in “correct” mode with default parameters. Sequences were checked for contamination using ConFindr 0.5.0 with default parameters (Low et al. 2019). Contigs were assembled from the trimmed and error-corrected reads using SKESA version 2.3.0 with the vector percent argument disabled (Souvorov et al. 2018). Pilon version 1.22 (Walker et al. 2014) was used to perform one round of automatic assembly improvement. Quality was assessed with Qualimap version 2.2.2 (García-Alcalde et al. 2012; Okonechnikov et al. 2016). Multilocus sequence typing (MLST) was conducted using the Center for Genomic Epidemiology MLST-2.0 Server (Larsen et al. 2012), which utilizes MLST allele sequence and profile data from PubMLST.org.
ARG detection in isolate sequences was conducted using both the Comprehensive Antibiotic Resistance Database Resistance Gene Identifier (CARD-RGI) tool version 5.1.0 with default settings (McArthur et al. 2013) and the CGE Bacterial Analysis Pipeline webtool ResFinder version 2.1 (cge.cbs.dtu.dk/services/ResFinder-2.1/) (Zankari et al. 2012) with the default settings for gene identity threshold (90%) and minimum length (60%) unless stated otherwise. Plasmid detection was conducted using the MOB-typer and MOB-recon tools from MOB-suite version 3.0.0 (Robertson and Nash 2018). ARG location determination was conducted by matching the contig encoding the ARG to the matching contig output as plasmid or chromosome by MOB-recon tool.
Nucleotide sequence accession numbers
Whole-genome sequences have been deposited at DDBJ/EMBL/GenBank under bioproject PRJNA489399. Sequence read archive accession numbers, MHT, PCR, WGS-ARG (ResFinder and CARD-RGI), and MALDI-TOF identification data can be found in Table S1. MOB-typer plasmid contents for each sequence can be found in Table S2.
Results and discussion
Characterization of carbapenem-tolerant strains recovered from WWTP influents
A total of 135 meropenem-tolerant strains (growth on ≥4 μg/mL meropenem) were recovered from WWTP influent samples from Toronto (50), Ottawa (51), and Arnprior (34). Genera identified included Aeromonas, Enterobacter, Klebsiella, Pandoraea, Providencia, Pseudomonas and Stenotrophomonas as determined using MALDI-TOF (Fig. 1b, Table S1).
Fig. 1.

All strains were tested for carbapenemase-production using the MHT which indicated 12, 7, and 0 carbapenemase-producing strains from Toronto, Ottawa, and Arnprior, respectively. Predicted carbapenemase producing strains included Aeromonas, Klebsiella, Pseudomonas, or Providencia genera (Table 2).
Table 2.
Genus | Total | No. of carbapenemase producersa |
---|---|---|
Aeromonas | 10 | 5 |
Enterobacter | 1 | 0 |
Klebsiella | 9 | 9 |
Pandoraea | 2 | 0 |
Providencia | 1 | 1 |
Pseudomonas | 95 | 4 |
Stenotrophomonas | 9 | 0 |
a
Number of carbapenemase producers determined using the modified Hodge test.
Identification of ARGs in isolates found in WWTP influents
Strains were screened for ARGs using a panel of 21 PCR assays to identify select β-lactamase, ESBL, and carbapenemase resistance genes (Table 1). PCR analysis indicated the presence of β-lactamase genes (bla) with the potential to hydrolyze carbapenems in 35 strains (Fig. 1a, Table S1). The majority of β-lactam-related ARGs were identified in isolates from the Toronto WWTP site, followed by Ottawa (Fig. 1a). Isolates from Arnprior exhibited the lowest number of ARGs, and the identified isolates mostly belonged to species not typically considered to be AMR indicators (e.g., P. fluorescens and P. putida) (Berendonk et al. 2015) (Fig. 1).
The PCR analyses focused on β-lactamase genes reported to confer carbapenem-resistance (Table 3) (Paterson et al. 2003; Monstein et al. 2007; Jacoby 2009; Bush 2010; Bonnin et al. 2011; Nordmann et al. 2011; Bogaerts et al. 2013; van Duin and Doi 2017). Genes for class A carbapenemases are typically found on transferrable plasmids and are highly prevalent among Enterobacteriaceae, with KPC being the most prevalent (van Duin and Doi 2017). The blaKPC gene was one of the most common ARGs detected by PCR among the isolates with one Klebsiella testing positive from the Ottawa site, as well as eight Klebsiella and a single Providencia from the Toronto site (Fig. 1a). The class A carbapenemase GES enzyme was detected in two Toronto and three Ottawa Aeromonas isolates (Figs. 1a and 2).
Fig. 2.

Table 3.
Active site | Molecular | Functional | bla genea | Locationb | Common name | Resistance | References |
---|---|---|---|---|---|---|---|
Serine | C | 1 | AmpC | C | Cephalosporinase | Cephalosporins, cephamycins | |
Ampa | C, P | ||||||
ACC | P | ||||||
ACT-1 | P | ||||||
CepH | C | ||||||
FOX-1 | P | ||||||
MIR-1 | P | ||||||
ACT-28c | C | ||||||
1, 1e | CMY-2, CMY-37 | C, P | Cephalosporinase, ESAC β-lactamase | Cephalosporins, cephamycins | |||
A | 2a | LEN-1 | C | Penicillinase | Penicillins | ||
2b | SHV-1 | C, P | Penicillinase | Penicillins, cephalosporins | |||
TEM-1, 2 | C, P | ||||||
2be | BEL-1 | P | ESBL | Extended-spectrum cephalosporins (cefepime), monobactams | |||
CTX-M | C, P | ||||||
OXY | C, P | ||||||
PER | P | ||||||
SHV-a | C, P | ||||||
TEM-a | C, P | ||||||
VEB | P | ||||||
2br, 2ber | SHV-a | C, P | ESBL | Penicillins (2br), extended-spectrum cephalosporins (cefepime), monobactams | |||
TEM-a | C, P | ||||||
2c | CARB-3 | C, P | Carbenicillinase | Penicillins, carbenicillin | |||
PSE | P | ||||||
2f | GES | C, P | Carbapenemase | Carbapenems | |||
IMI | C, P | ||||||
KPC | C, P | ||||||
D | 2d | OXA-1, OXA-10 | C, P | Cloxacillinase | Penicillins, cloxacillin | ||
2de | OXA-11, OXA-15 | C, P | Oxacillinase, Extended spectrum cephalosporinase | Extended-spectrum cephalosporins | |||
2df | OXA-23, OXA-48 | C, P | Carbapenemase | Carbapenems | |||
Zinc | B1 | 3a | IMP-1 | C, P | Metallo-β-lactamase | Most β-lactams including carbapenems | |
NDM | C, P | ||||||
VIM | C, P | ||||||
B2 | 3b | cphA | C, P | Metallo-β-lactamase | More strictly carbapenems | ||
Sfh-1 | C | ||||||
B3 | 3a | L-1, -2 | C, P | Metallo-β-lactamase | Most β-lactams including carbapenems | ||
PAM | C | ||||||
POM | C |
Note
Molecular, molecular classification; Functional, functional classification; ESAC, extended spectrum AmpC; ESBL, extended spectrum β-lactamase.
a
Some gene variants exhibit broader-spectrum resistance to additional antimicrobials, therefore are in separate functional categories. ESBLs are also able to hydrolise the 4th generation cephalosporin cefepime, whereas AmpCs can hydrolyse cephamycins.
b
Gene location(s) refer to those commonly cited in literature; however, these may vary by isolate (transposition and insertion may occur).
c
Enzyme ACT-28 associated with decreased membrane permeability has been found to result in decreased carbapenem sensitivity (Jousset et al. 2019).
The metallo-β-lactamases VIM and IMP were detected in Pseudomonas spp. isolates recovered from both Toronto and Ottawa (Figs. 1 and 2). VIM and IMP are both Class B, metallo-β-lactamases which generally have weak β-lactamase activity against all β-lactams except monobactams but are able to hydrolyze carbapenems (Table 3) (Bush 2010). They are frequently associated with multiple AMR determinants and have spread globally to many of the Enterobacteriaceae (Nordmann et al. 2011; Codjoe and Donkor 2018).
PCR analysis was also conducted to investigate non-carbapenemase class A ESBL genes (Table 3), of which nine isolates were predicted to harbour blaPER (n = 1) and blaCTX-M (n = 8) based on PCR results (Fig. 1a). However, WGS analysis of four CTX-M PCR-positive isolates did not detect the blaCTX-M gene but instead detected the class A ESBL blaOXY-6. The chromosomal class A enzymes of Klebsiella oxytoca (blaOXY) have been documented as one of the β-lactamases most closely related to the CTX-M family, sharing 70%–75% amino acid identity (Tzouvelekis et al. 2000; Bonnet 2004). Analysis of the universal CTX-M-U primers used in this study revealed that the primers used annealed to regions of conserved sequence in both the blaCTX-M and blaOXY genes (Fig. S1). To our knowledge, this is the first report of false positive binding of CTX-M universal primers with OXY-type enzymes.
The blaOXY encoded enzyme is a chromosomal class A β-lactamase discovered and reported in K. oxytoca isolates, conferring amino- and carboxy-penicillin resistance (Fevre et al. 2005). K. oxytoca is an opportunistic pathogen and documented causative agent of antibiotic-associated hemorrhagic colitis (Högenauer et al. 2006). Eight K. oxytoca were isolated from the Toronto WWTP during this study. Each of the K. oxytoca isolates that were sequenced encoded both KPC-2 and OXY-6-2 enzymes (Fig. 2). Reports from Brazil and the United States have also characterized clinical K. oxytoca isolates producing the K. pneumoniae carbapenemase enzyme KPC-2 (Yigit et al. 2003; Almeida et al. 2013). Shared environments between K. pneumoniae and K. oxytoca may facilitate horizontal gene transfer and permit adaptation to new environments across species (Moradigaravand et al. 2017). This suggests K. oxytoca may be an equally suitable 1MR indicator and is of concern as shared habitats such as wastewater may permit transfer of AMR to clinically relevant bacterial species and possibly facilitate entrance of multidrug-resistant (MDR) organisms into the environment.
As this study did not include PCR analyses for all possible β-lactamase-related resistance genes, WGS was conducted for a subset of 46 isolates from each WWTP including isolates with and without PCR-identified resistance genes to confirm ARGs and to investigate additional bla genes that may have contributed to carbapenem tolerance. WGS analyses detected additional β-lactamase encoding genes including ampS, blaACT, blaCARB, blaCEPH, cphA, blaFOX, blaMOX, blaOXY, blaL1, and blaPAM (Fig. 2). No ARGs were detected in eleven of the sequenced isolates using ResFinder-2.1 or CARD-RGI (Fig. 2, isolates: OLC2865, 2866, 2868, 2691, 2871, 3144, 3145, 3147, 3634, 3635, and 3636). ResFinder also failed to detect any ARGs in both Pandoraea apista (Burkholderiaceae) isolates (OLC2679 and 3043); however, both had PCR positive results for blaOXA-10 and CARD-RGI detected blaOXA-153 at 99.6% identity (Fig. 2, Table S1). Lowering ResFinder-2.1 thresholds to 80% identity (ID) enabled detection of blaOXA-62 at 83.46%. This suggests the identity thresholds used with ResFinder may have been too stringent, and some ARG-alleles may not be included in the ResFinder database. Similarly, blastn analysis using blaL1, a chromosomal metallo-β-lactamase from Stenotrophomonas maltophilia (Accession: EF126059), resulted in positive hits for the S. maltophilia isolates OLC2691, OLC2871, OLC3147, and OLC3635 (Fig. 2). ResFinder analysis of the S. maltophilia isolates with the %ID threshold lowered to 80 resulted in positive hits for blaL1 at 89.57%, 88.69%, 89.70%, and 89.70%, respectively. In contrast, CARD-RGI did not detect the blaL1 gene in any of these isolates.
Likewise, ResFinder v2.1 and v3.2 with lower ID threshold reported blaACT-4 at 85.08% and blaACT-9 at 98.86%, respectively, in the Enterobacter asburiae isolate (OLC3633). Jousset et al. (2019) recently described an Enterobacter kobei lineage with weak carbapenemase activity due to the chromosomally encoded AmpC gene blaACT-28. CARD-RGI analysis of OLC3633 detected ACT-28 (accession: NG_048614), which is not present in the ResFinder database, at 98.95% identity. In contrast to Jousset et al. (2019) who found isolates were resistant or less sensitive to many β-lactams including ESBLs and expanded spectrum cephalosporins, but not imipenem and meropenem, the Enterobacter asburiae isolated in this study exhibited resistance to meropenem at 4 μg/mL. In addition to AmpCs and ESBLs, porin mutations or modified expression has also been found to confer resistance in Enterobacter species (Codjoe and Donkor 2018; Hao et al. 2018) and may have contributed to resistance in this isolate.
The remaining seven isolates with no identifiable β-lactam ARGs were either Pseudomonas fluorescens or Pseudomonas putida (Fig. 2). Neither CARD-RGI, ResFinder analysis with %ID and minimum length lowered to minimum values, nor blastn (BLAST with default settings) analyses of sequences to known Pseudomonas spp. metallo-β-lactamase genes (Thaller et al. 2011; Suzuki et al. 2014; Borgianni et al. 2015) found any identifiable carbapenem-resistance genes in these isolates. It is possible that these Pseudomonas isolates exhibit carbapenem-tolerance due to reduced outer membrane permeability and (or) increased efflux as has been described elsewhere (Livermore 2001, 2002; Tacão et al. 2015; Kittinger et al. 2016; Codjoe and Donkor 2018; Jousset et al. 2019).
Plasmid-encoded ARGs
MOB-recon predicted a combined 111 plasmids in 24 of the 46 sequenced isolates. Of the 111 plasmids 17 were predicted as conjugative, 34 were predicted as mobilizable, and 24 included contigs which encoded ARGs (Table S2). Plasmid incompatibility groups included ColRNAI (n = 5), IncC (n = 2), IncFIA (n = 3), IncFIB (n = 6), IncFII (n = 7), IncP (n = 8), IncR (n = 2), IncQ1 (n = 2), IncQ2 (n = 2), and IncX3 (n = 1) (Fig. 2, Table S2).
All five of the sequenced Klebsiella spp. contained plasmids encoding ARGs. In contrast, while each of the six sequenced Aeromonas spp. isolates encoded plasmids, only three of these Aeromonas isolates’ plasmids included contigs encoding ARGs (Table S2). However, short read sequences such as those generated in this study can be challenging to assemble, preventing complete plasmid sequences from being accurately reconstructed (Orlek et al. 2017). MOB suite is dependent on a plasmid database to provide accurate results, and the authors acknowledge that it will not perform well on novel plasmids lacking sequence similarity to those in the database (Robertson and Nash 2018). The database contents are biased towards plasmids from Enterobacteriaceae (Robertson and Nash 2018), presenting an additional obstacle. Although some of the resistance genes identified in this study are known to be chromosomal, it is likely that MOB suite was unable to accurately reconstruct all plasmids for these wastewater isolates as there may not have been matches in current public databases. To determine ARG location more accurately in these isolates (Table S2), additional work to isolate and transfer plasmid(s) and (or) long-read sequencing will need to be conducted.
Use of indicator organisms for monitoring AMR in WWTPs
In addition to monitoring specific ARGs, AMR surveillance programs also recommend monitoring for the presence of bacterial indicators of the Gammaproteobacteria class as these are the most frequent ARG carriers (Berendonk et al. 2015). Pseudomonas aeruginosa and Aeromonas spp. have been proposed as indicators in environmental samples where fecal contamination is not expected. Bacterial indicators where fecal contamination is expected include Escherichia coli, Enterococcus faecalis, Enterococcus faecium, and Klebsiella spp. The recommended indicator organisms are ubiquitous as well as carriers of clinically relevant ARG(s) in multiple environments. A variety of different bacterial species were identified using MALDI-TOF (Fig. 1b) and WGS (Fig. 2). Suggested AMR-indicators of the genera Aeromonas and Klebsiella were isolated from both the Toronto and Ottawa samples tested (Fig. 1b, Table S1), which correlated with an increased number of carbapenem-resistance genes (Fig. 1a). Every Klebsiella and Aeromonas spp. isolated and identified during this study harboured multiple ARGs, some of which were located on plasmids.
Klebsiella spp. have been recommended as possible indicators to assess AMR status in environmental settings as they are considered early indicators of new AMR emergence (Berendonk et al. 2015; Navon-Venezia et al. 2017). We identified eight K. oxytoca and one K. pneumoniae in the Toronto and Ottawa samples (respectively) (Sievert et al. 2013; Moradigaravand et al. 2017). The K. pneumoniae isolated from Ottawa was notable for its MDR genotype. It was predicted to contain several plasmids and multiple ARGs including resistance to aminoglycosides, fluoroquinolones, macrolides, phenicols, sulphonamides, and trimethoprim, in addition to the β-lactamases blaTEM-1, blaSHV-11, blaKPC-3, blaCARB-2, and blaOXA-9 (OLC2685, Fig. 2). Netikul and Kiratisin (Netikul and Kiratisin 2015) found carbapenem-resistant K. pneumoniae isolated from a university hospital in Thailand were resistant to carbapenems at a higher ratio than other CREs, harboured multiple β-lactamase and ESBL genes, and (or) expressed altered porin expression, yet only two isolates (out of 36 they tested) encoded commonly associated carbapenemase genes (e.g., blaKPC), suggesting the need to investigate other mechanisms of carbapenem-resistance in isolates.
Other than Klebsiella spp. a number of isolates belonging to the AMR-indicator genus Aeromonas were also isolated from the Toronto and Ottawa sites (Figs. 1b and 2), although this genus is suggested as an indicator where fecal contamination is not expected. Members of the genus Aeromonas are ubiquitous opportunistic pathogens primarily found in aquatic environments but have also been isolated from clinical, soil, and animal environments (Varela et al. 2016; Chenia and Duma 2017). Several studies have investigated members of Aeromonas as a reservoir of AMR in aquatic environments (Figueira et al. 2011; Trudel et al. 2016; Varela et al. 2016). Trudel et al. (2016) found 37% of tested Canadian A. salmonicida isolates contained at least one resistance plasmid and could pose a potential risk for transfer of resistant genetic material to other waterborne bacteria (including Salmonella spp.). MALDI-TOF identified three isolates from each of the Toronto and Ottawa samples as Aeromonas spp. (Table S1). WGS analysis identified six Aeromonas isolates from Toronto (n = 4) and Ottawa (n = 2), four and three of which encoded blaGES and blaOXA β-lactamase genes, respectively. WGS detected β-lactamase ARGs blaFOX, ampS, blaOXA, blaGES, blaCEPH, cphA2, and blaMOX-6 in aeromonads isolated during this study (Fig. 2). Overall, 10 Aeromonas spp. isolates were identified using a combination of MALDI-TOF and WGS from Toronto (n = 5) and Ottawa (n = 5) (Table S1).
Species identification of Arnprior isolates, which had few known carbapenem or β-lactam resistance genes, found a large proportion of pseudomonads that were not P. aeruginosa (Figs. 1 and 2). AMR in P. aeruginosa has been attributed to expression of modifying enzymes, efflux pumps, lower membrane permeability, and chromosomal mutations (Moriyama et al. 2009; Chatterjee 2016). While P. aeruginosa has been well studied and is documented to be inherently resistant and exhibit decreased susceptibility to many antibiotics (Livermore 2002; Breidenstein et al. 2011), there are relatively few studies regarding AMR mechanisms in P. fluorescens and P. putida. It is possible that carbapenem resistance in the pseudomonads isolated from Arnprior is due to presence of chromosomal ampC genes and (or) altered membrane permeability as has been observed in other Pseudomonas species (Breidenstein et al. 2011; Molina et al. 2014; Suzuki et al. 2014; Borgianni et al. 2015).
Conclusion
Wastewater is an important source of AMR where there is potential for widespread dissemination into the environment. As clinical, industrial, household, and agricultural practices all connect to wastewater systems, this also makes WWTPs useful sites to monitor the AMR burden within an environment and assess the risk of transfer to humans both directly and indirectly through food production. A large number of indicator genera (e.g., Klebsiella) and organisms harbouring AMR indicator resistance genes (e.g., blaKPC, blaTEM, blaVIM, etc.) were identified in both Toronto and Ottawa influent samples. Only a single Pandoraea sp. isolate purified from the smaller rural community of Arnprior harboured a β-lactamase resistance gene (blaOXA-153), which has been reported to cause elevated minimum inhibitory concentrations (MICs) to carbapenems (Schneider and Bauernfeind 2015). None of the isolates from Arnprior were identified as AMR indicator species.
Limitations of this study include the small sample size at each WWTP site, PCR analyses not being conducted for all known carbapenem-inactivating ARGs, that other β-lactamase enzymes may also hydrolyze carbapenems to some extent resulting carbapenem-tolerance, that efflux and porin mutations possibly conferring carbapenem tolerance were not investigated, and that the selection method used was not comprehensive for all bacteria found in wastewater. Future studies would ideally sample a number of times over multiple seasons, as well as test a larger number of isolates and account for clones within samples. While this study utilized PCR and WGS of bacteria isolated from samples, other molecular techniques including quantitative PCR, metagenomics, and 16S rRNA sequencing allow rapid sample analysis while avoiding cultivation and have previously been used to study wastewater (Böckelmann et al. 2009; Muurinen et al. 2017; Ng et al. 2017; Freeman et al. 2018; Rocha et al. 2019).
We found that although PCR is an economical high-throughput method for gene detection, when phenotypes could be the product of a multitude of genes including carbapenemases, cephalosporinases, and ESBLs, requiring multiple experiments and multiplex-PCRs, WGS may actually be more beneficial. These results highlight the value of WGS for AMR detection in bacterial isolates, especially for genera that are not routinely monitored. However, we did find both ResFinder-2.1 and CARD-RGI to be biased towards well-studied bacteria, such as members of Enterobacteriaceae, and suggest investigations of non-Enterobacteriaceae may benefit from using lower gene-identity thresholds or conducting analyses with a more comprehensive AMR-gene database. The inability to determine the cause of carbapenem tolerance in seven Pseudomonas spp. isolates is evidence additional, uncharacterized resistance mechanisms still exist.
Funding
This study has received funding from the Government of Canada interdepartmental Genomic Research Development Initiative (GRDI-AMR program).
Acknowledgements
We gratefully acknowledge technical assistance from Paul Manninger, Martine Dixon, Mylène Deschênes, Susan van Zanten, Adam Koziol, Andrew Low, Julie Shay, and Pam Auchterlonie, as well as Rudy Jones for his help and collaboration.
References
Aarestrup FM, and Woolhouse MEJ. 2020. Using sewage for surveillance of antimicrobial resistance. Science, 367: 630–632.
Almeida ACS, Cavalcanti FLS, Martins WMB, Vilela MA, Gales AC, Morais MA Jr, et al. 2013. First description of KPC-2-producing Klebsiella oxytoca in Brazil. Antimicrobial Agents and Chemotherapy, 57: 4077–4078.
Anderson KL, Whitlock JE, and Harwood VJ. 2005. Persistence and differential survival of fecal indicator bacteria in subtropical waters and sediments. Applied and Environmental Microbiology, 71: 3041–3048.
Andrews S. 2010. FastQC: a quality control tool for high throughput sequence data [online]: Available from bioinformatics.babraham.ac.uk/projects/fastqc/.
Avison MB, Higgins CS, von Heldreich CJ, Bennett PM, and Walsh TR. 2001. Plasmid location and molecular heterogeneity of the L1 and L2 β-lactamase genes of Stenotrophomonas maltophilia. Antimicrobial Agents and Chemotherapy, 45: 413–419.
Bartolini A, Frasson I, Cavallaro A, Richter SN, and Palù G. 2014. Comparison of phenotypic methods for the detection of carbapenem non-susceptible Enterobacteriaceae. Gut Pathogens, 6: 13.
Ben-David D, Kordevani R, Keller N, Tal I, Marzel A, Gal-Mor O, et al. 2012. Outcome of carbapenem resistant Klebsiella pneumoniae bloodstream infections. Clinical Microbiology and Infection, 18: 54–60.
Berendonk TU, Manaia CM, Merlin C, Fatta-Kassinos D, Cytryn E, Walsh F, et al. 2015. Tackling antibiotic resistance: the environmental framework. Nature Reviews Microbiology, 13: 310.
Böckelmann U, Dörries H-H, Ayuso-Gabella MN, de Marçay MS, Tandoi V, Levantesi C, et al. 2009. Quantitative PCR monitoring of antibiotic resistance genes and bacterial pathogens in three European artificial groundwater recharge systems. Applied and Environmental Microbiology, 75: 154–163.
Bogaerts P, de Castro RR, de Mendonça R, Huang T-D, Denis O, and Glupczynski Y. 2013. Validation of carbapenemase and extended-spectrum β-lactamase multiplex endpoint PCR assays according to ISO 15189. Journal of Antimicrobial Chemotherapy, 68: 1576–1582.
Bonnet R. 2004. Growing group of extended-spectrum β-lactamases: the CTX-M enzymes. Antimicrobial Agents and Chemotherapy, 48: 1–14.
Bonnin RA, Nordmann P, Potron A, Lecuyer H, Zahar J-R, and Poirel L. 2011. Carbapenem-hydrolyzing GES-type extended-spectrum β-lactamase in Acinetobacter baumannii. Antimicrobial Agents and Chemotherapy, 55: 349–354.
Borgianni L, Luca FD, Thaller MC, Chong Y, Rossolini GM, and Docquier J-D. 2015. Biochemical characterization of the POM-1 metallo-β-lactamase from Pseudomonas otitidis. Antimicrobial Agents and Chemotherapy, 59: 1755–1758.
Boyd DA, Mataseje LF, Davidson R, Delport JA, Fuller J, Hoang L, et al. 2017. Enterobacter cloacae complex isolates harboring blaNMC-A or blaIMI-type class A carbapenemase genes on novel chromosomal integrative elements and plasmids. Antimicrobial Agents and Chemotherapy, 61: e02578-16.
Breidenstein EBM, de la Fuente-Núñez C, and Hancock REW. 2011. Pseudomonas aeruginosa: all roads lead to resistance. Trends in Microbiology, 19: 419–426.
Bush K. 1999. beta-Lactamases of increasing clinical importance. Current Pharmaceutical Design, 5: 839–845.
Bush K. 2010. Bench-to-bedside review: the role of β-lactamases in antibiotic-resistant Gram-negative infections. Critical Care, 14: 224.
Bush K, and Jacoby GA. 2010. Updated functional classification of β-lactamases. Antimicrobial Agents and Chemotherapy, 54: 969–976.
Bush K, Jacoby GA, and Medeiros AA. 1995. A functional classification scheme for beta-lactamases and its correlation with molecular structure. Antimicrobial Agents and Chemotherapy, 39: 1211–1233.
Bushnell B. 2014. BBMap: a fast, accurate, splice-aware aligner [online]: Available from sourceforge.net/projects/bbmap/.
Chang H-J, Hsu P-C, Yang C-C, Kuo A-J, Chia J-H, Wu T-L, et al. 2011. Risk factors and outcomes of carbapenem-nonsusceptible Escherichia coli bacteremia: a matched case-control study. Journal of Microbiology, Immunology and Infection, 44: 125–130.
Chatterjee M. 2016. Antibiotic resistance in Pseudomonas aeruginosa and alternative therapeutic options. International Journal of Medical Microbiology, 306: 48–58.
Chenia HY, and Duma S. 2017. Characterization of virulence, cell surface characteristics and biofilm-forming ability of Aeromonas spp. isolates from fish and sea water. Journal of Fish Diseases, 40: 339–350.
Clinical and Laboratory Standards Institute (CLSI). 2011. Performance standards for antimicrobial susceptibility testing; 19th informational supplement. Document M100-S21.
Clinical and Laboratory Standards Institute (CLSI). 2020. Performance standards for antimicrobial susceptibility testing. 30th edition. Document M100-ED30:2020.
Codjoe FS, and Donkor ES. 2018. Carbapenem resistance: a review. Medical Sciences, 6: 1.
Davison J. 1999. Genetic exchange between bacteria in the environment. Plasmid, 42: 73–91.
Diene SM, and Rolain J-M. 2014. Carbapenemase genes and genetic platforms in Gram-negative bacilli: Enterobacteriaceae, Pseudomonas and Acinetobacter species. Clinical Microbiology and Infection, 20: 831–838.
Esterly JS, Wagner J, McLaughlin MM, Postelnick MJ, Qi C, and Scheetz MH. 2012. Evaluation of clinical outcomes in patients with bloodstream infections due to Gram-negative bacteria according to carbapenem MIC stratification. Antimicrobial Agents and Chemotherapy, 56: 4885–4890.
Fevre C, Jbel M, Passet V, Weill F-X, Grimont PAD, and Brisse S. 2005. Six groups of the OXY β-lactamase evolved over millions of years in Klebsiella oxytoca. Antimicrobial Agents and Chemotherapy, 49: 3453–3462.
Figueira V, Vaz-Moreira I, Silva M, and Manaia CM. 2011. Diversity and antibiotic resistance of Aeromonas spp. in drinking and waste water treatment plants. Water Research, 45: 5599–5611.
Freeman CN, Scriver L, Neudorf KD, Hansen LT, Jamieson RC, and Yost CK. 2018. Antimicrobial resistance gene surveillance in the receiving waters of an upgraded wastewater treatment plant. FACETS, 3: 128–138.
García-Alcalde F, Okonechnikov K, Carbonell J, Cruz LM, Götz S, Tarazona S, et al. 2012. Qualimap: evaluating next-generation sequencing alignment data. Bioinformatics, 28: 2678–2679.
Girlich D, Poirel L, and Nordmann P. 2012. Value of the modified Hodge test for detection of emerging carbapenemases in Enterobacteriaceae. Journal of Clinical Microbiology, 50: 477–479.
Gonzalez Leiza M, Perez-Diaz JC, Ayala J, Casellas JM, Martinez-Beltran J, Bush K, et al. 1994. Gene sequence and biochemical characterization of FOX-1 from Klebsiella pneumoniae, a new AmpC-type plasmid-mediated beta-lactamase with two molecular variants. Antimicrobial Agents and Chemotherapy, 38: 2150–2157.
Hao M, Ye M, Shen Z, Hu F, Yang Y, Wu S, et al. 2018. Porin deficiency in carbapenem-resistant Enterobacter aerogenes strains. Microbial Drug Resistance, 24: 1277–1283.
Hembach N, Schmid F, Alexander J, Hiller C, Rogall ET, and Schwartz T. 2017. Occurrence of the mcr-1 colistin resistance gene and other clinically relevant antibiotic resistance genes in microbial populations at different municipal wastewater treatment plants in Germany. Frontiers in Microbiology, 8: 1282.
Högenauer C, Langner C, Beubler E, Lippe IT, Schicho R, Gorkiewicz G, et al. 2006. Klebsiella oxytoca as a causative organism of antibiotic-associated hemorrhagic colitis. New England Journal of Medicine, 355: 2418–2426.
Hutinel M, Huijbers PMC, Fick J, Åhrén C, Larsson DGJ, and Flach C-F. 2019. Population-level surveillance of antibiotic resistance in Escherichia coli through sewage analysis. Eurosurveillance, 24: 1800497.
Jacoby GA. 2009. AmpC β-lactamases. Clinical Microbiology Reviews, 22: 161–182.
Jacoby GA, Mills DM, and Chow N. 2004. Role of beta-lactamases and porins in resistance to ertapenem and other beta-lactams in Klebsiella pneumoniae. Antimicrobial Agents and Chemotherapy, 48: 3203–3206.
Jousset AB, Oueslati S, Bernabeu S, Takissian J, Creton E, Vogel A, et al. 2019. False-positive carbapenem-hydrolyzing confirmatory tests due to ACT-28, a chromosomally encoded AmpC with weak carbapenemase activity from Enterobacter kobei. Antimicrobial Agents and Chemotherapy, 63: e02388-18.
Kittinger C, Lipp M, Baumert R, Folli B, Koraimann G, Toplitsch D, et al. 2016. Antibiotic resistance patterns of Pseudomonas spp. isolated from the River Danube. Frontiers in Microbiology, 7: 586.
Krahn T, Wibberg D, Maus I, Winkler A, Bontron S, Sczyrba A, et al. 2016. Intraspecies transfer of the chromosomal Acinetobacter baumannii blaNDM-1 carbapenemase gene. Antimicrobial Agents and Chemotherapy, 60: 3032–3040.
Larsen MV, Cosentino S, Rasmussen S, Friis C, Hasman H, Marvig RL, et al. 2012. Multilocus sequence typing of total-genome-sequenced bacteria. Journal of Clinical Microbiology, 50: 1355–1361.
Li B, Yang Y, Ma L, Ju F, Guo F, Tiedje JM, et al. 2015. Metagenomic and network analysis reveal wide distribution and co-occurrence of environmental antibiotic resistance genes. The ISME Journal, 9: 2490–2502.
Liu CM, Aziz M, Kachur S, Hsueh P-R, Huang Y-T, Keim P, et al. 2012. BactQuant: an enhanced broad-coverage bacterial quantitative real-time PCR assay. BMC Microbiology, 12: 56.
Livermore DM. 2001. Of Pseudomonas, porins, pumps and carbapenems. Journal of Antimicrobial Chemotherapy, 47: 247–250.
Livermore DM. 2002. Multiple mechanisms of antimicrobial resistance in Pseudomonas aeruginosa: our worst nightmare? Clinical Infectious Diseases, 34: 634–640.
Low AJ, Koziol AG, Manninger PA, Blais BW, and Carrillo CD. 2019. ConFindr: rapid detection of intraspecies and cross-species contamination in bacterial whole-genome sequence data. PeerJ, 7: e6995.
McArthur AG, Waglechner N, Nizam F, Yan A, Azad MA, Baylay AJ, et al. 2013. The comprehensive antibiotic resistance database. Antimicrobial Agents and Chemotherapy, 57: 3348–3357.
Molina L, Udaondo Z, Duque E, Fernández M, Molina-Santiago C, Roca A, et al. 2014. Antibiotic resistance determinants in a Pseudomonas putida strain isolated from a hospital. PLoS ONE, 9: e81604.
Monstein H-J, Östholm-Balkhed Å, Nilsson MV, Nilsson M, Dornbusch K, and Nilsson LE. 2007. Multiplex PCR amplification assay for the detection of blaSHV, blaTEM and blaCTX-M genes in Enterobacteriaceae. APMIS, 115: 1400–1408.
Moradigaravand D, Martin V, Peacock SJ, and Parkhill J. 2017. Population structure of multidrug-resistant Klebsiella oxytoca within hospitals across the United Kingdom and Ireland identifies sharing of virulence and resistance genes with K. pneumoniae. Genome Biology and Evolution, 9: 574–584.
Moriyama K, Wiener-Kronish JP, and Sawa T. 2009. Protective effects of affinity-purified antibody and truncated vaccines against Pseudomonas aeruginosa V-antigen in neutropenic mice. Microbiology and Immunology, 53: 587–594.
Mulvey MR, Soule G, Boyd D, Demczuk W, Ahmed R, and Multi-provincial Salmonella Typhimurium Case Control Study Group. 2003. Characterization of the first extended-spectrum beta-lactamase-producing Salmonella isolate identified in Canada. Journal of Clinical Microbiology, 41: 460–462.
Muurinen J, Karkman A, and Virta M. 2017. High throughput method for analyzing antibiotic resistance genes in wastewater treatment plants. In Antimicrobial resistance in wastewater treatment processes. Edited by PL Keen and R Fugère. John Wiley & Sons, Ltd. pp. 253–262.
Navon-Venezia S, Kondratyeva K, and Carattoli A. 2017. Klebsiella pneumoniae: a major worldwide source and shuttle for antibiotic resistance. FEMS Microbiology Reviews, 41: 252–275.
Netikul T, and Kiratisin P. 2015. Genetic characterization of carbapenem-resistant Enterobacteriaceae and the spread of carbapenem-resistant Klebsiella pneumonia ST340 at a university hospital in Thailand. PLoS ONE, 10: e0139116.
Ng C, Tay M, Tan B, Le T-H, Haller L, Chen H, et al. 2017. Characterization of metagenomes in urban aquatic compartments reveals high prevalence of clinically relevant antibiotic resistance genes in wastewaters. Frontiers in Microbiology, 8: 2200.
Noble WC, Virani Z, and Cree RG. 1992. Co-transfer of vancomycin and other resistance genes from Enterococcus faecalis NCTC 12201 to Staphylococcus aureus. FEMS Microbiology Letters, 93: 195–198.
Nordmann P, Naas T, and Poirel L. 2011. Global spread of carbapenemase-producing Enterobacteriaceae. Emerging Infectious Diseases, 17: 1791–1798.
Okonechnikov K, Conesa A, and García-Alcalde F. 2016. Qualimap 2: advanced multi-sample quality control for high-throughput sequencing data. Bioinformatics, 32: 292–294.
Orlek A, Stoesser N, Anjum MF, Doumith M, Ellington MJ, Peto T, et al. 2017. Plasmid classification in an era of whole-genome sequencing: application in studies of antibiotic resistance epidemiology. Frontiers in Microbiology, 8: 182.
Pärnänen KMM, Narciso-da-Rocha C, Kneis D, Berendonk TU, Cacace D, Do TT, et al. 2019. Antibiotic resistance in European wastewater treatment plants mirrors the pattern of clinical antibiotic resistance prevalence. Science Advances, 5: eaau9124.
Paterson DL, Hujer KM, Hujer AM, Yeiser B, Bonomo MD, Rice LB, et al. 2003. Extended-spectrum β-lactamases in Klebsiella pneumoniae bloodstream isolates from seven countries: dominance and widespread prevalence of SHV- and CTX-M-type β-lactamases. Antimicrobial Agents and Chemotherapy, 47: 3554–3560.
Philippon A, Slama P, Dény P, and Labia R. 2016. A structure-based classification of class A β-lactamases, a broadly diverse family of enzymes. Clinical Microbiology Reviews, 29: 29–57.
Pierce VM, Simner PJ, Lonsway DR, Roe-Carpenter DE, Johnson JK, Brasso WB, et al. 2017. Modified carbapenem inactivation method for phenotypic detection of carbapenemase production among Enterobacteriaceae. Journal of Clinical Microbiology, 55: 2321–2333.
Poirel L, Dortet L, Bernabeu S, and Nordmann P. 2011. Genetic features of blaNDM-1-positive Enterobacteriaceae. Antimicrobial Agents and Chemotherapy, 55: 5403–5407.
Queenan AM, and Bush K. 2007. Carbapenemases: the versatile β-lactamases. Clinical Microbiology Reviews, 20: 440–458.
Robertson J, and Nash JHE. 2018. MOB-suite: software tools for clustering, reconstruction and typing of plasmids from draft assemblies. Microbial Genomics, 4: e000206.
Rocha J, Fernandes T, Riquelme MV, Zhu N, Pruden A, and Manaia CM. 2019. Comparison of culture- and quantitative PCR-based indicators of antibiotic resistance in wastewater, recycled water, and tap water. International Journal of Environmental Research and Public Health, 16: 4217.
Rossolini GM, Zanchi A, Chiesurin A, Amicosante G, Satta G, and Guglielmetti P. 1995. Distribution of cphA or related carbapenemase-encoding genes and production of carbapenemase activity in members of the genus Aeromonas. Antimicrobial Agents and Chemotherapy, 39: 346–349.
Saavedra MJ, Peixe L, Sousa JC, Henriques I, Alves A, and Correia A. 2003. Sfh-I, a subclass B2 metallo-β-lactamase from a Serratia fonticola environmental isolate. Antimicrobial Agents and Chemotherapy, 47: 2330–2333.
Schneider I, and Bauernfeind A. 2015. Intrinsic carbapenem-hydrolyzing oxacillinases from members of the genus Pandoraea. Antimicrobial Agents and Chemotherapy, 59: 7136–7141.
Segatore B, Massidda O, Satta G, Setacci D, and Amicosante G. 1993. High specificity of cphA-encoded metallo-beta-lactamase from Aeromonas hydrophila AE036 for carbapenems and its contribution to beta-lactam resistance. Antimicrobial Agents and Chemotherapy, 37: 1324–1328.
Shaikh S, Fatima J, Shakil S, Rizvi SMD, and Kamal MA. 2015. Antibiotic resistance and extended spectrum beta-lactamases: types, epidemiology and treatment. Saudi Journal of Biological Sciences, 22: 90–101.
Sievert DM, Ricks P, Edwards JR, Schneider A, Patel J, Srinivasan A, et al. 2013. Antimicrobial-resistant pathogens associated with healthcare-associated infections: summary of data reported to the National Healthcare Safety Network at the Centers for Disease Control and Prevention, 2009–2010. Infection Control and Hospital Epidemiology, 34: 1–14.
Sims N, and Kasprzyk-Hordern B. 2020. Future perspectives of wastewater-based epidemiology: monitoring infectious disease spread and resistance to the community level. Environment International, 139: 105689.
Somboro AM, Sekyere JO, Amoako DG, Essack SY, and Bester LA. 2018. Diversity and proliferation of metallo-β-lactamases: a clarion call for clinically effective metallo-β-lactamase inhibitors. Applied and Environmental Microbiology, 84: e00698-18.
Souvorov A, Agarwala R, and Lipman DJ. 2018. SKESA: strategic k-mer extension for scrupulous assemblies. Genome Biology, 19: 153.
Suzuki M, Suzuki S, Matsui M, Hiraki Y, Kawano F, and Shibayama K. 2014. A subclass B3 metallo-β-lactamase found in Pseudomonas alcaligenes. Journal of Antimicrobial Chemotherapy, 69: 1430–1432.
Tacão M, Correia A, and Henriques IS. 2015. Low prevalence of carbapenem-resistant bacteria in river water: resistance is mostly related to intrinsic mechanisms. Microbial Drug Resistance, 21: 497–506.
Tamma PD, Opene BNA, Gluck A, Chambers KK, Carroll KC, and Simner PJ. 2017. Comparison of 11 phenotypic assays for accurate detection of carbapenemase-producing Enterobacteriaceae. Journal of Clinical Microbiology, 55: 1046–1055.
Thaller MC, Borgianni L, Di Lallo G, Chong Y, Lee K, Dajcs J, et al. 2011. Metallo-β-lactamase production by Pseudomonas otitidis: a species-related trait. Antimicrobial Agents and Chemotherapy, 55: 118–123.
Trudel MV, Vincent AT, Attéré SA, Labbé M, Derome N, Culley AI, et al. 2016. Diversity of antibiotic-resistance genes in Canadian isolates of Aeromonas salmonicida subsp. salmonicida: dominance of pSN254b and discovery of pAsa8. Scientific Reports, 6: 35617.
Tzouvelekis LS, Tzelepi E, Tassios PT, and Legakis NJ. 2000. CTX-M-type β-lactamases: an emerging group of extended-spectrum enzymes. International Journal of Antimicrobial Agents, 14: 137–142.
van Duin D, and Doi Y. 2017. The global epidemiology of carbapenemase-producing Enterobacteriaceae. Virulence, 8: 460–469.
Varela AR, Nunes OC, and Manaia CM. 2016. Quinolone resistant Aeromonas spp. as carriers and potential tracers of acquired antibiotic resistance in hospital and municipal wastewater. Science of the Total Environment, 542: 665–671.
von Wintersdorff CJH, Penders J, van Niekerk JM, Mills ND, Majumder S, van Alphen LB, et al. 2016. Dissemination of antimicrobial resistance in microbial ecosystems through horizontal gene transfer. Frontiers in Microbiology, 7: 173.
Walker BJ, Abeel T, Shea T, Priest M, Abouelliel A, Sakthikumar S, et al. 2014. Pilon: an integrated tool for comprehensive microbial variant detection and genome assembly improvement. PLoS ONE, 9: e112963.
Walther-Rasmussen J, and Høiby N. 2007. Class A carbapenemases. Journal of Antimicrobial Chemotherapy, 60: 470–482.
Ye J, Coulouris G, Zaretskaya I, Cutcutache I, Rozen S, and Madden TL. 2012. Primer-BLAST: a tool to design target-specific primers for polymerase chain reaction. BMC Bioinformatics, 13: 134.
Yigit H, Queenan AM, Rasheed JK, Biddle JW, Domenech-Sanchez A, Alberti S, et al. 2003. Carbapenem-resistant strain of Klebsiella oxytoca harboring carbapenem-hydrolyzing β-lactamase KPC-2. Antimicrobial Agents and Chemotherapy, 47: 3881–3889.
Zankari E, Hasman H, Cosentino S, Vestergaard M, Rasmussen S, Lund O, et al. 2012. Identification of acquired antimicrobial resistance genes. Journal of Antimicrobial Chemotherapy, 67: 2640–2644.
Zhan Z, Hu L, Jiang X, Zeng L, Feng J, Wu W, et al. 2018. Plasmid and chromosomal integration of four novel blaIMP-carrying transposons from Pseudomonas aeruginosa, Klebsiella pneumoniae and an Enterobacter sp. Journal of Antimicrobial Chemotherapy, 73: 3005–3015.
Zurfluh K, Hächler H, Nüesch-Inderbinen M, and Stephan R. 2013. Characteristics of extended-spectrum β-lactamase- and carbapenemase-producing Enterobacteriaceae isolates from rivers and lakes in Switzerland. Applied and Environmental Microbiology, 79: 3021–3026.
Supplementary materials
Supplementary Material 1, Table S1 (XLSX / 25.6 KB)
- Download
- 25.70 KB
Supplementary Material 2, Table S2 (XLSX / 19.8 KB)
- Download
- 19.83 KB
Supplementary Material 3, Fig. S1 (JPG / 3.52 MB)
- Download
- 3.52 MB
Information & Authors
Information
Published In
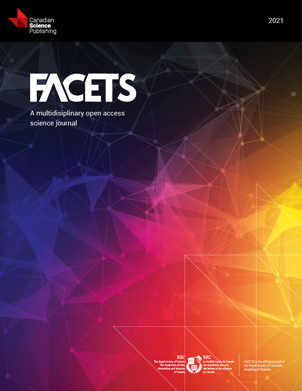
FACETS
Volume 6 • Number 1 • January 2021
Pages: 569 - 591
Editor: Elena P. Ivanova
History
Received: 5 November 2020
Accepted: 18 January 2021
Version of record online: 22 April 2021
Copyright
© 2021 Cooper et al. This work is licensed under a Creative Commons Attribution 4.0 International License (CC BY 4.0), which permits unrestricted use, distribution, and reproduction in any medium, provided the original author(s) and source are credited.
Data Availability Statement
All relevant data are within the paper and in the Supplementary Material.
Key Words
Sections
Subjects
Authors
Author Contributions
ALC, CDC, and BWB conceived and designed the study.
ALC, CC, HM, MW, and PS performed the experiments/collected the data.
ALC, CC, HM, MW, PS, and ST analyzed and interpreted the data.
ST, AW, CDC, and BWB contributed resources.
All drafted or revised the manuscript.
Competing Interests
The authors have declared that no competing interests exist.
Metrics & Citations
Metrics
Other Metrics
Citations
Cite As
Ashley L. Cooper, Cassandra Carter, Hana McLeod, Marie Wright, Prithika Sritharan, Sandeep Tamber, Alex Wong, Catherine D. Carrillo, and Burton W. Blais. 2021. Detection of carbapenem-resistance genes in bacteria isolated from wastewater in Ontario. FACETS.
6(): 569-591. https://doi.org/10.1139/facets-2020-0101
Export Citations
If you have the appropriate software installed, you can download article citation data to the citation manager of your choice. Simply select your manager software from the list below and click Download.
Cited by
1. A Review of the Dissemination of Antibiotic Resistance through Wastewater Treatment Plants: Current Situation in Sri Lanka and Future Perspectives
2. Presence and Persistence of ESKAPEE Bacteria before and after Hospital Wastewater Treatment
3. Antibiotic resistance determinants among carbapenemase producing bacteria isolated from wastewaters of Kathmandu, Nepal
4. Diversity of
bla
POM in carbapenem-resistant opportunistic pathogenic
Pseudomonas otitidis
in municipal wastewater
5. Rapid detection of multiple resistance genes to last-resort antibiotics in Enterobacteriaceae pathogens by recombinase polymerase amplification combined with lateral flow dipstick
6. Clinically Relevant β-Lactam Resistance Genes in Wastewater Treatment Plants
7. A resistome survey across hundreds of freshwater bacterial communities reveals the impacts of veterinary and human antibiotics use
8. Genomic Analysis of Carbapenem-Resistant
Comamonas
in Water Matrices: Implications for Public Health and Wastewater Treatments
9. Simultaneous gut colonization by Klebsiella grimontii and Escherichia coli co-possessing the blaKPC-3-carrying pQil plasmid
10. Carbapenem Resistance among Marine Bacteria—An Emerging Threat to the Global Health Sector