Combining high-resolution remotely sensed data with local and Indigenous Knowledge to model the landscape suitability of culturally modified trees: biocultural stewardship in Kitasoo/Xai’xais Territory
Abstract
Environmental management and monitoring must reconcile social and cultural objectives with biodiversity stewardship to overcome political barriers to conservation. Suitability modelling offers a powerful tool for such “biocultural” approaches, but examples remain rare. Led by the Stewardship Authority of the Kitasoo/Xai’xais First Nation in coastal British Columbia, Canada, we developed a locally informed suitability model for a key biocultural indicator, culturally modified trees (CMTs). CMTs are trees bearing evidence of past cultural use that are valued as tangible markers of Indigenous heritage and protected under provincial law. Using a spatial multi-criteria evaluation framework to predict CMT suitability, we developed two cultural predictor variables informed by Kitasoo/Xai’xais cultural expertise and ethnographic data in addition to six biophysical variables derived from LiDAR and photo interpretation data. Both cultural predictor variables were highly influential in our model, revealing that proximity to known habitation sites and accessibility to harvesters (by canoe and foot) more strongly influenced suitability for CMTs compared with site-level conditions. Applying our model to commercial forestry governance, we found that high CMT suitability areas are 51% greater inside the timber harvesting land base than outside. This work highlights how locally led suitability modelling can improve the social and evidentiary dimensions of environmental management.
Introduction
Biological diversity is strongly linked to social, cultural, and economic resilience. Increasingly, there is a need to connect these dimensions in environmental management and monitoring (EMM) projects to reverse global declines in biological diversity and promote resilient cultures and communities (Lertzman 2009; De Groot et al. 2010; von der Porten et al. 2019; Zurba et al. 2019; Walker et al. 2020). Barriers to implementation can manifest when conservation oriented policies and decision-making processes are not locally informed or led (DeRoy et al. 2019). In cross-cultural biodiversity conservation or stewardship implementation, innovating new ways to support locally led stewardship initiatives is critical to meeting both local and global biodiversity conservation goals (Gavin et al. 2018). New strategies must directly include social and cultural values and support Indigenous rights and authority in EMM, which are integral to social–ecological systems (Sterling et al. 2017b; Artelle et al. 2018; Ban et al. 2018, 2020; Salomon et al. 2018; Pinkerton et al. 2019; Zurba et al. 2019; Caverley et al. 2020). “Biocultural approaches” can offer an efficacious method to guide EMM objectives towards achieving these outcomes, because they focus conservation goals and interventions on values that are important to local communities (Verschuuren 2012; Pungetti 2013; Gavin et al. 2015; Sterling et al. 2017a; DeRoy et al 2019).
Biocultural approaches seek to protect and steward linkages between humans and ecological systems by supporting locally led EMM objectives and by adopting a partnership-based approach to bridge social–ecological linkages across spatial and institutional scales (Caillon et al. 2017; Sterling et al. 2017a; Wali et al. 2017; Gavin et al. 2018; DeRoy et al 2019; von der Porten et al. 2019). Addressing biocultural EMM objectives requires monitoring processes that focus on locally important values, species, and the relationships among them (Gallagher and Josephs 2008; Verschuuren 2012; Artelle et al. 2018; DeRoy et al. 2019). Success in protecting and stewarding social–ecological linkages can be measured through diverse methodologies, ontologies, and knowledge systems (e.g., Indigenous methodologies, perceptions-based approaches, natural sciences, or empirical social sciences; Newing 2010; Bennett 2016; Caillon et al. 2017).
A range of these innovative cross-cultural approaches to EMM that explicitly adopt values that reflect local peoples and respect Indigenous rights and laws are emerging. For example, researchers in Australia have developed an environmental management framework in collaboration with two Indigenous groups in the Kimberly region—the Bardi Jawi and Nyul Nyul—that is guided by key Indigenous wetland stewardship principles (Pyke et al. 2018). This framework has helped transcend inter-cultural and inter-agency barriers and promote a guiding stewardship planning framework in which “wetlands need people” (Pyke et al. 2018). Similarly, the Omora Ethnobotanical Park in Tierra del Fuego, Chile, has incorporated 10 principles based on Yaghan (or Yámana) Indigenous Knowledge and stewardship practice to guide development of the Park’s mission and to align objectives among institutions involved, including the Yaghan People and Chilean government (Rozzi et al. 2006).
Beyond guiding principles and establishing frameworks, analytical approaches that support interdisciplinary, cross-cultural approaches to implementing biocultural EMM are required. Suitability modelling has been used in “functional ecological” approaches to EMM (i.e., those that do not include biocultural indicators) for decades (Rodríguez et al. 2007; Elith and Leathwick 2009; Franklin 2010; Guisan et al. 2013). Common suitability modelling methods that are used in functional ecological approaches are machine learning based, such as random forest models or maximum entropy (Guisan et al. 2017). However, suitability modelling in this context has had mixed success in achieving desired EMM outcomes. Failures can often be attributed to error propagation in the models (Heuvelink 1998; Store and Kangas 2001). This can be caused by use of ex situ occurrence data (i.e., observational data gathered outside the management area), or by inclusion of environmental predictor variables that do not strongly contribute to, or limit, the given habitat or distribution of focal species (Store and Kangas 2001; Franklin 2010). Therefore, suitability modelling is more likely to support outcomes when occurrence data used to evaluate the model and predictor influence are locally derived, and (or) the model predictor variables are locally informed (Polfus et al. 2014). Such approaches provide new opportunities to link local values with local data in EMM.
One method that is particularly well suited for the incorporation of local and expert knowledge in a suitability modelling framework is multi-criteria evaluation (MCE; Eastman 1999). MCE is a modelling process in which a suite of potential criteria or predictor variables that contribute to the suitability of a given outcome are empirically compared against one another to determine the relative influence of each predictor on the expected outcome (i.e., location of an important resource; Eastman 1999; Store and Jokimäki 2003). MCE methods have been used to incorporate local expert knowledge as part of suitability modelling frameworks in a diverse array of applications, including decision-making models and habitat suitability modelling (Store and Kangas 2001; Polfus et al. 2014, 2016). Local expertise can be especially important when prior empirical study is limited or nonexistent (Store and Kangas 2001). However, local guidance and place-based expertise are critical to any biocultural approach to suitability modelling or other EMM endeavours regardless of whether prior empirical study has occurred or not (Salomon et al. 2018).
The area now referred to as the Great Bear Rainforest (GBR) of British Columbia, Canada, is a globally significant biocultural diversity hotspot that is rich in place-based EMM expertise. The GBR is comprised of intertwined socio-cultural and marine-influenced terrestrial biogeoclimatic systems that have been heavily influenced by thousands of years of stewardship practice by First Nations Peoples (Lepofsky et al. 2017; Lepofsky and Armstrong 2018). Indeed, First Nations People in this region have been engaged in EMM directly with many species of cultural importance for over 14 000 years (Mclaren et al. 2015; Mackie et al. 2018). The Kitasoo/Xai’xais (KX) First Nation, among many others, are continuing this stewardship in a contemporary context by developing and applying their own approaches to stewardship informed by their Indigenous laws to manage both ecological and cultural values. Further, recent provincial legislation that applies to the GBR (Great Bear Rainforest Land Use Objectives Order (GBR LUO; British Columbia Ministry of Forests, Lands and Natural Resource Operations 2016)), was developed to recognize and support the implementation of Indigenous-led stewardship in a collaborative government-to-government structure. The GBR LUO includes specific protection targets (often percentage based) for ecological features such as ecotypes and critical habitat for endangered species with less-defined goals for culturally significant features in this region.
This legal process recognizes culturally modified trees (CMTs) as a priority cultural value included as an “Aboriginal Heritage Feature”. CMTs can be defined as trees, both living and dead, that bear evidence of traditional or cultural use by First Nations People (British Columbia Archaeology Branch 2001). Examples of CMTs in coastal British Columbia include bark removal, felling and removal of logs for wood products (such as canoes or building and carving materials), pitch collection, inner bark collection, and wood plank removal from live standing trees. Although empirical study on CMTs is limited in the GBR—especially for bark-stripped CMTs—this work builds off of recent work by others in the region that focused on another common type of CMT referred to as “aboriginally logged” features that were harvested for wood resources (Benner et al. 2019).
We focus here on CMTs that have been harvested for bark—a material central to current and historical Indigenous cultural practice—in a way that typically allows the tree to heal and continue growing (and in some cases be harvested multiple times over many years; British Columbia Archaeology Branch 2001; Turner et al. 2009; Earnshaw 2017). Generally, trees suitable for harvesting bark need to have a bole surface free of knots and branches. Smaller diameter trees can be more suitable for pulling tapered bark strips (often on the upslope face), whereas larger diameter trees are more suitable for removing rectangular bark strips (British Columbia Archaeology Branch 2001). However, the practise of intergenerational stewardship provides sustained harvesting opportunities on the younger healing lobes that form after bark is pulled, thereby allowing bark to be pulled for hundreds of years on even the oldest and largest diameter cedar trees (Earnshaw 2017, 2019; Stafford 2017). We focus particularly on CMTs that are visible to field surveyors (i.e., not healed-over completely), which were likely culturally modified within the last ∼250 years. Importantly, CMTs provide evidence of Indigenous use spanning centuries and serve as biocultural indicators that can help identify culturally significant areas that may also be biologically rich (Garibaldi and Turner 2004; Turner et al. 2009; Sutherland et al. 2016; Benner et al. 2019; DeRoy et al. 2019).
Despite their value to Indigenous peoples, CMTs were not protected until relatively recently and at small spatial scales. They received protection as “archaeological sites” under the provincial Heritage Conservation Act (British Columbia Archaeology Branch 1996), but only for CMTs dating prior to 1846—an arbitrary colonial construct that limits heritage management protection status (Turner et al. 2009; Earnshaw 2017). The cumulative impacts that widespread commercial timber harvest imposed on CMTs prior to their initial protection in the 1980s is likely immense (Oliver 2007; Turner et al. 2009; Earnshaw 2017). Further, in other regions empirically informed estimates suggest that roughly half of all bark-stripped CMTs are not currently visible to archaeologists and CMT surveyors because the tree has completely healed over the scar, which can lead to continued undocumented removal of CMTs through commercial forestry even after their legislated protection (Earnshaw 2017, 2019). This undocumented removal of CMTs may impact the outcomes of Indigenous rights and title cases brought before Canadian courts, due to the removal of physical evidence of long-term occupation and use (Earnshaw 2017). Documented removal of CMTs can also occur via site alteration permits issued by the provincial Archaeology Branch, which typically require expressed support or approval by First Nations. The impacts from commercial forestry continue as old growth cedar including both western redcedar (Thuja plicata) and yellow-cedar (Cupressus nootkatensis) remain targets for commercial harvest in British Columbia. Against this background of historical and ongoing impacts and their value as markers of Indigenous heritage, occupation, and stewardship, as well as their legal protection, documenting remaining CMTs comprises a stewardship priority for many Indigenous Nations in western Canada.
Historically, colonial governments have largely excluded Indigenous governments from decision-making regarding biodiversity conservation and resource extraction in Canada and abroad. However, the political landscape is changing in Canada and abroad with the commitment to implement the United Nations General Assembly (2007) Declaration on the Rights of Indigenous Peoples and to reconcile the relationships between Indigenous and colonial governments. Although there is increasing interest in co-governance, approaches still commonly follow dominant western science paradigms to which Indigenous governments, communities, and knowledge holders are expected to conform.
For example, the GBR LUO takes a spatially explicit approach to managing commercial forestry in the region by allocating percentage-based retention targets for each landscape unit and ecotype to be protected from commercial forestry (British Columbia Ministry of Forests, Lands and Natural Resource Operations 2016). The province of British Columbia has invested decades of research and development with teams of spatial analysts to create spatially explicit, ecological inventory data sets that combine remotely sensed data with field observations to model the distribution of ecological features, like ecological communities and rare ecosystems, and economic features such as stand volume (British Columbia Ministry of Forests, Lands and Natural Resource Operations 2018). In parallel, but dating back millennia, many Indigenous governments also have abundant knowledge and data in the form of local and Indigenous Knowledge, oral histories and, more recently, traditional use studies. These forms of information and data, however, are not easily translated to interact with percentage-based targets for commercial timber harvest limits and, unlike ecological inventory data, most cultural heritage features have not been surveyed or inventoried.
The resulting political and practical barriers are manifold. For example in the context of spatial scale, data from traditional use studies often refer to specific points or culturally significant places with amorphous boundaries that cannot be divided in the same way as forest stand types. At a political and governance scale, the barriers are more hindering. Laws, regulations, and other colonial government systems are slow to adapt to new co-governance agreements. For example, the Chief Forester with the Province of British Columbia sets the Allowable Annual Cut for this region and many others based purely on timber supply modelling, which does not typically take into account Indigenous lifeways, practices, histories, or links to cultural well-being beyond excluding recorded archaeological sites from model outputs. Amendment to such colonial regulations, laws, and governance processes often requires the burden of proof or evidence on behalf of Indigenous governments. In the context of these political and data-oriented barriers, some First Nations Governments in the GBR are developing their own spatially explicit analyses based on ecological and cultural values that are important to their communities to support culturally relevant governance.
Given this context and focus, our team, which includes members from academia and the KX Stewardship Authority, used suitability modelling approaches that link local stewardship values and data. Specific research goals as identified by the KX Stewardship Authority for this project included: (i) gathering data from areas with low previous survey effort, (ii) evaluating potential biophysical and cultural predictor variables that might predict site suitability and distribution of CMTs, and (iii) providing directly relevant information to KX Lands Managers about where culturally modified trees are likely to occur, particularly in the context of commercial forestry. Towards these goals we developed CMT suitability models using records from field surveys in addition to archived records from the British Columbia Archaeology Branch to inform KX forest management and to support greater equity in spatial data and analysis available during co-governance.
Methods
Summary
We used a spatially explicit MCE modelling framework that used sensitivity analysis to assess the relative influence of cultural and biophysical predictor variables in predicting CMT occurrence. CMT data were sourced from recent 2018 field surveys as well as archived archaeological surveys (British Columbia Archaeology Branch 2018), which we georeferenced. We chose to use an inductive MCE modelling framework instead of empirical machine learning approaches because it provided a structured step-by-step approach that could be easily adapted to reflect expert opinion during all stages of model development and (Store and Kangas 2001).
Study area
The study area is located on what is now referred to as the Central Coast of British Columbia, Canada. Much of the Central Coast remains roadless, except for isolated networks of forestry roads. The study area covers ∼118 845 ha (Fig. 1) and is encompassed within a portion of KX Territory, spanning the temperate rainforests of the coastal mainland and a marine archipelago. We derived several of the predictor variables in the model from available LiDAR data, which set the maximum extent of the study region. The LiDAR extent covers much of the spatial area where commercial forestry is permitted to occur in KX Territory (i.e., outside of established protected areas).
Fig. 1.

CMT occurrence
We derived CMT data via georeferencing archived scanned archaeological sitemaps maps accessed through the British Columbia Remote Access to Archaeological Data (RAAD) system (n =126). We used a minimum of four registration points for each map using a projected ESRI world imagery basemap with NAD83 BC Environment Albers projection and datum. We used defined landscape features such as creeks, roads, shorelines, and cutblock boundaries to add registration points. We excluded six hand-drawn site form maps (out of a total of 132 maps) from our analysis due to imprecision. We added registration points until the map aligned with clearly defined landscape features and the scale of the georeferenced map was within ±5 m of the base map scale. We then added points for all CMTs that were depicted on the sitemaps.
The total number of CMTs that we georeferenced from archived archaeological sitemaps included tapered bark strip scars (n = 339) and rectangular bark strip scars (n = 172; Table 1). We excluded 232 CMTs (of 511; ∼45%) from the RAAD source that fell within cutblock boundaries where commercial forestry operations had either removed (via a site alteration permit) or left a small buffer of standing trees around the recorded CMTs. We excluded these cutblock observations because we used tree crown height as a variable in our model (below), and crown heights were altered by tree removal subsequent to initial documentation.
Table 1.
CMT subtype | Field survey (n) | RAAD (n) | Total |
---|---|---|---|
Taper strip | 184 | 339 | 523 |
Rectangular S\strip | 93 | 172 | 265 |
Sum of recorded CMTs | 277 | 511 | 788 |
CMTs included in model | 277 | 279 | 556 |
In 2018, we conducted over 110 km of field surveys for CMTs with KX Stewardship Technicians (Supplementary Material 1, S1). These surveys were conducted to increase survey coverage in areas that might potentially be underrepresented by previous archaeological survey effort and make up for observations that were excluded due to their proximity to cut blocks. Field survey data were recorded with electronic data recorders equipped with GPS signal boosters (Trimble Geospatial R1 Integrated GNSS Receiver, Sunnyvale, California) that increased spatial accuracy of recorded CMTs to within ≤10 m. CMT subtypes from our 2018 surveys include “tapered bark strip” (n = 184) scars and “rectangular bark strip” (n = 93) scars (Fig. 2; Table 1). We used CMT observations that were bark stripped because these CMT subtypes were the most common subtype observed (bark stripped CMTs n = 788, other CMT subtypes n = 103). Further, the site conditions and suitability for different CMT subtypes may vary, which could influence the inference of our results (Stryd and Eldridge 1993).
Fig. 2.

We then converted the remaining 556 CMTs from both data sets to a raster surface using the “Point to Raster” tool in ArcMap version 10.6 (Redlands, California). Most of these CMTs occur on western redcedar (hereafter redcedar, n = 526) and yellow-cedar (n = 30). Since some points fell within the same 25 m × 25 m raster cells, the resulting raster surface containing n = 413 cells served as the response variable used to evaluate the suitability models.
Suitability modelling approach
We assembled a suite of eight predictor variables (Elevation, a Cost-Distance Submodel, Distance from Known Habitation Sites (e.g., shell midden sites), a Crown Height Model, Slope, Aspect, and the percent Canopy Cover of yellow-cedar and redcedar) based on existing literature and Kitasoo/Xai’xais Stewardship Authority’s staff knowledge. These predictors were also selected based on the form and quality of data and knowledge available, which included LiDAR, a combination of photo interpretation and satellite imagery derived data and local and Indigenous Knowledge (Table 2). We applied scale transformation functions (linear, logistic growth, and symmetric linear) to variables, based on available information from both the literature and local and Indigenous Knowledge. The cell resolution was aggregated from 1 m2 to 25 m2 to reduce computational requirements for model processing and better match the spatial resolution of the photo interpretation and satellite imagery derived variables. We incorporated these predictor variables in a multi-criteria evaluation framework (Supplementary Material 1, S4) to evaluate the relative influence of each predictor variable on the presence of CMTs (response variable).
Table 2.
Variable category | Variable name | Source | Description of variable transformation | References |
---|---|---|---|---|
Cultural | Cost-Distance Submodel | Local and Indigenous Knowledge, LiDAR, Canadian Hydrographic Service | Submodel used canoe landing beaches as source cells and slope as cost surface, scaled linearly | Gustas and Supernant 2017; Kitasoo/Xai’xais 2018; Vernon Brown 2018* |
Cultural | Distance from Known Habitation Sites | Local and Indigenous Knowledge, Archaeological Record | Euclidean distance from known habitation sites in 1 km intervals, scaled linearly | Blake Evans 2018*; Kitasoo/Xai’xais 2018; Vernon Brown 2018* |
Biophysical | Elevation | LiDAR | Elevation reclassified in 100 m intervals; lower more suitable than higher, scaled linearly | Arcas Consulting 1999; Karpiak 2003 |
Biophysical | Crown Height Model | LiDAR | Rescaled by logistic growth function, trees <10 m not suitable | Arcas Consulting 1999; Brown and Brown 2009 |
Biophysical | Slope | LiDAR | Rescaled by exponential function such that more suitable slopes are <60° | Arcas Consulting 1999; Benner et al. 2019 |
Biophysical | Aspect | LiDAR | Rescaled by symmetric linear function to favour north-facing aspects | Stryd and Eldridge 1993; Vernon Brown 2018* |
Biophysical | Canopy Cover redcedar | BC VRI 2018 (Satellite Imagery) | Percent canopy cover of western redcedar; rescaled by logistic growth function, with greater proportion of canopy cover associated with higher suitability. | Stewart 1984; Stafford and Maxwell 2006; Turner et al. 2009 |
Biophysical | Canopy Cover yellow-cedar | BC VRI 2018 (Satellite Imagery) | Percent canopy cover of yellow-cedar; rescaled by logistic growth function, with greater proportion of canopy cover associated with higher suitability | Stewart 1984; Stafford and Maxwell 2006; Turner et al. 2009 |
Note
We applied scale transformation functions (linear, logistic, exponential and symmetric linear) to variables, based on available information from both the literature and local and Indigenous knowledge.
*
Personal communication.
MCE is the process of transforming, weighting, and combining variables resulting in an output that represents suitability based on desired attributes (Eastman 1999; Supplementary Material 1, S4). The transformations and weights assigned to variables can be informed in a variety of ways. Expert opinion, local knowledge, and Indigenous Knowledge are treated with equal value to empirical data when informing variable selection and transformation and can also serve a valuable role when other data are lacking. Further, MCE allows the researcher or resource manager to examine a wide range of weighting scenarios to gain insight into the predictive power and the relative sensitivity of each variable in reference to occurrence data as weights are manipulated. Our MCE process consisted of multiple steps: standardizing the variables, rescaling the variables, applying a range of weights to each variable to assess the sensitivity and predictive influence of each variable, and finally determining a best-fit weighting scheme to produce a principal weights CMT model (Fig. S1).
We used the Analytical Hierarchy Process (AHP) to assign a rank to each predictor based on the sensitivity analysis results and derived a model with best-fit weights (Saaty 1980; Store and Kangas 2001). Usually AHP is used to assign best-fit weights based on subjective ranks determined by expert opinion. Here, however, we use the relative importance rank that was determined in the MCE to inform the AHP ranks. We assigned a rank to each predictor variable based on Saaty’s (1980) scale of importance, a 1 being least important and 9 exceedingly important. We used the AHP (v2.0) extension for Arcmap, which implements Saaty’s (1980) standard pairwise comparison matrix and calculates the resulting principal weights for each variable. We used the resulting best-fit model to project suitability across the study area and gain insight into the distribution of different suitability score classes to inform forestry practices. We also evaluated the effect of predictor variables in a null model using random point locations instead of CMTs locations (Tables S1 and S2). Multicollinearity between all variables was low with all variance of inflation scores <1.6 (Table S2).
Remotely sensed biophysical variables
We used remote sensing products during the development of predictor variables that were incorporated in the models. We used LiDAR data that were collected and processed by Western Forest Products and shared via a data-use agreement. LiDAR data were collected using a full waveform sensor (model Riegl LMS-Q780, Horn, Austria) mounted on a fixed wing aircraft using a beam divergence of <0.25 mrad and a scan angle of ±30° and 55%–60% swath overlap with an absolute horizontal accuracy of 15 cm (95% confidence) and an absolute vertical accuracy of 10 cm (95% confidence). Raw LiDAR points were reclassified into a bare earth digital elevation model (hereafter Elevation) and a tree crown height model (CHM). Elevation was derived from the mean value of all ground points within 1 m2, and the CHM was derived from the highest point value compared with the lowest point value within each square metre cell. We also derived variables for Slope and Aspect from Elevation using the “Slope” tool and “Aspect” tool in Arcmap. We aggregated all the LiDAR-derived variables from 1 m2 to 25 m2 using the mean value for all but the CHM to capture the average geophysical site conditions within each cell. We used the median value for the CHM to capture the average dominant canopy height within each 25 m × 25 m cell to give better insight into the representative upper canopy conditions within each cell.
In addition to the LiDAR data, aerial and satellite imagery data were used for the development of canopy cover measures of western redcedar and yellow-cedar. The Forest Inventory Branch of the Province of BC created the Vegetation and Resource Inventory (BC VRI) database using photo interpretation methods in combination with satellite imagery and regional ground truthing (British Columbia Ministry of Forests, Lands and Natural Resource Operations 2018). We utilized the processed BC VRI data to calculate the percent canopy cover (hereafter Canopy Cover) for both yellow-cedar and redcedar within each polygon and then converted these layers to raster using the “Polygon to Raster tool”.
Cultural variables
Until recently, archaeological predictive modelling has largely omitted the use of local and Indigenous Knowledge (Supernant 2017). As part of creating locally grounded models we wanted to incorporate cultural variables that reflected local and Indigenous Knowledge of cedar harvesting, an activity that has been practiced continually for thousands of years in the study area. Cultural variables are those that incorporate some aspect of local cultural practice or way of life into the design of the spatial predictor variable. Vernon Brown, fieldwork co-lead, cultural knowledge holder, and co-author, developed the conceptual framework for both cultural variables included in this analysis. Vernon Brown holds extensive local and Indigenous Knowledge of the Territory from lived experience on the land, work with archaeologists, and from conducting hundreds of hours of Traditional Use Study interviews with KX Elders and members.
Cost-Distance Submodel variable
Based on this local insight, we hypothesized that the distribution of currently visible CMTs (those made within the last ∼250 years) were more likely to occur in areas accessible from marine and terrestrial resource harvesting areas and travel corridors, which would involve a combination of canoe and foot travel. These principles informed the conceptual framework for our Cost-Distance Submodel, parameterized with a cost-distance surface (Supplementary Material 1, S3). To estimate harvester accessibility, we reclassified shoreline morphology data from the Canadian Hydrographic Service to convert shorelines that support ocean-going canoe accessibility (e.g., sandy banks, gravel, cobble, boulders and rocky shelves) to source cells from which accumulated cost could be calculated. We then used the LiDAR-derived slope layer as the cost surface to calculate cost distance from the source cells (canoe accessible shorelines). Trail networks and other infrastructure (e.g., lake canoes) would have likely improved the ease of travel to inland harvesting and stewardship areas. Accordingly, we reclassified lakes over 10 ha (on which canoes would likely be present) from “no data” (or barrier cells) to 0% slope to represent low energetic cost travel by lake canoe. The resulting variable offers increased heterogeneity compared to a standard “distance from shoreline” variable to capture a locally informed and fine-tuned picture of the past and present cultural landscape as it relates to cedar bark harvest.
Distance from Known Habitation Sites variable
We also included Distance from Known Habitation Sites as a predictor variable because we hypothesized that known habitation sites, camps, and long-term subsistence archaeological features indicated more extensive cultural activity (e.g., cedar bark harvest). Many of these sites are associated with marine resources. Further, KX Indigenous Knowledge suggested a need to appropriately represent the strong interactions between marine and terrestrial environment in regards to KX resource stewardship. This variable additionally pairs local and Indigenous Knowledge of landscape utilization with existing archaeological records. We combined spatial data from traditional use interviews with community members (conducted prior to this study; Kitasoo/Xai’xais 2018) with recorded archaeological sites in the provincial archaeological database that had at least one indicator of habitation or sustained cultural activity (e.g., halibut (Hippoglossus stenolepis) or herring (Clupea pallasii) harvesting camps, fish traps, shell midden, canoe runs, or house platforms, etc.). Although many of the habitation sites occur along the shoreline, this variable offers variation in the form of both distance along shore and distance inland from the nearest habitation site.
Colocation of CMT suitability and commercial forestry
To understand the utility of the best-fit model in the context of current forest management we compared the principal weights CMT model with the existing spatial area designated for commercial timber harvesting in the region (“timber harvesting land base”; THLB). The THLB refers to the spatial extent of areas that are likely to be suitable for commercial timber harvest and is represented by polygons also derived from the BC VRI database. These polygons were developed by the Forest Inventory Branch based on the economic viability of the timber and some area-based protections defined in the GBR LUO. To better understand the potential for future impacts on CMTs, we compared the spatial distribution of the THLB with values derived from our CMT suitability model. We expected there would be overlap between highly suitable CMT stands and those potentially allocated for commercial forestry, but quantifying this overlap (and identifying specific areas of overlap) was important for current and future forest management and land use planning. Accordingly, we conducted an overlay analysis to calculate the relative proportion of high (>7.5), moderate (5.5–7.5), and low (1.3–5.5) suitability areas (using equal breaks) that occur inside and outside the THLB.
Results
MCE
Predictor variables differed in their utility to predict CMT distribution in KX Territory. The MCE showed that the suitability predictions of known CMT locations had strong relationships with Elevation and cultural predictor variables—the Cost-Distance Submodel and Distance from Known Habitation Sites variables. Elevation had the highest mean suitability scores for each weighted scenario whereas the percent Canopy Cover of yellow-cedar variable had the lowest range of suitability scores (Table 3). We found that the CHM, Distance from Known Habitation Sites, the Cost-Distance Submodel and Elevation all display an increase in suitability score with increased weight, while Slope, Aspect, and Canopy Cover of yellow-cedar and redcedar display decreasing suitability scores with increased weights (Table 3). With increased weights, the suitability scores for Slope, Aspect, and Canopy Cover of redcedar and yellow-cedar all fall below the equal weights suitability score, suggesting these variables do not meaningfully influence the prediction of known CMT locations (Table 3).
Table 3.

Note
Suitability scores represent the sum of all predictors multiplied by their weights in each model run. The sum of the applied weight added to the equalized weights of remaining variables is equal to 1. Higher suitability scores (dark green) signify a prediction of higher suitability in cells containing observed CMTs by the predictor with an increased weight. Lower suitability scores (dark purple) represent lower means in CMT cells with increased weights. Results were rescaled from a scale of 0–1 to a scale of 1–10 for ease of interpretation.
Standard deviations lend further insight into the relative performance of predictor variables. Higher standard deviations suggest less consistency in the predictive influence of the weighted variable. The Cost-Distance Submodel had the lowest and most stable range of standard deviations compared to all other predictors. The standard deviations for Elevation and the Canopy Cover for yellow-cedar predictors also had relatively low ranges of standard deviations (Table 4).
Table 4.

Note
Darker shades of green are associated with lower standard deviation, and darker shades of purple are associated with higher. Higher standard deviation signifies that there is a higher degree of uncertainty in how the given layer represents suitability for CMTs; lower standard deviation signifies less uncertainty. Table cells correspond to the means in Table 3.
Null model comparison
The range of mean suitability scores was much lower in the null MCE compared with the CMT MCE (Tables S1 and 3). Mean scores also differed significantly (t = 7.95, df = 140.01, α = 0.05, p < 0.001). Therefore, the predictor variables selected and developed with support from local and Indigenous Knowledge and literature (Table 2) enabled us to generate suitability models that predicted the locations of CMTs significantly better than random locations.
Determining the optimal weighting scheme
The results from the AHP analysis allowed us to develop a principal weights CMT model. The principal weighting scheme calculated from the AHP pairwise comparison ranks (Table S1) with a Consistency Ratio < 0.1 assigned the highest weights to Elevation, Distance from Known Habitation Sites, and Cost-Distance Submodel variables and the lowest weights to the Canopy Cover of redcedar and yellow-cedar variables (Table 5). The resulting model showed a significantly higher mean suitability score in the cells containing CMTs than the mean suitability score of the study area (t = 48.8, df = 340, α = 0.05, p < 0.001) (Figs. 3 and 4). The model predicted high suitability for CMTs for ∼12 089 ha, or 10.2% of the study area, moderate suitability across ∼76 555 ha (64.4%) and low suitability over 30 607 ha (25.5%).
Table 5.
Layer name | Analytical hierarchy process rank | Principal weight (%) |
---|---|---|
Cost-Distance Submodel | 8 | 18.5 |
Elevation | 8 | 18.5 |
Distance from known habitation sites | 8 | 18.5 |
Crown Height Model | 7 | 16.4 |
Slope | 4 | 9.3 |
Aspect | 4 | 9.2 |
% Canopy Cover redcedar | 3 | 7.0 |
% Canopy Cover yellow-cedar | 1 | 2.4 |
Note
Ranks vary from 1 to 8, with 8 signifying greatest influence.
Fig. 3.

Fig. 4.

Colocation of CMT suitability and commercial forestry
There is a high degree of spatial overlap between high suitability CMT stands and the spatial area that is likely to contain commercial timber harvesting in the study area (Table 6). We note that commercial harvesting of trees—and potentially CMTs—can also occur outside the THLB. We found that high suitability locations for CMTs make up ∼13% of the spatial area inside the THLB, which is 51% greater than the area of high suitability locations for CMTs outside the THLB (∼9%; Table 6). Moderate suitability cultural cedar stands comprise ∼74% of the area inside the THLB and ∼61% outside. Low suitability CMT stands account for ∼12% of area inside the THLB and ∼30% outside.
Table 6.
Suitability score range | Suitability class | Total no. of cells | Proportion of study area | Total hectares | Relative proportion inside THLB | Relative proportion outside THLB |
---|---|---|---|---|---|---|
>7.5 | High | 193 416 | 10.2% | 12 089 | 13.4% | 8.9% |
5.5–7.5 | Moderate | 1 224 880 | 64.4% | 76 555 | 74.3% | 60.8% |
1.4–5.5 | Low | 489 717 | 25.8% | 30 607 | 12.3% | 30.3% |
Note
Model values were reclassified into high, moderate, and low suitability classes. THLB, timber harvesting land base.
Discussion
We developed a unique suitability modelling approach to not only support Kitasoo/Xai’xais governance of their Territory but also as a generalizable framework that could be used by other Nations in the context of co-governance or Indigenous-led governance. This approach draws from a diverse range inputs including, field survey, archived archaeological site reports, local and Indigenous Knowledge, remotely sensed satellite and LiDAR data to model landscape suitability for CMTs, a key biocultural indicator. We found that Elevation and the cultural variables are among the most powerful predictors for CMT distribution in KX Territory. In particular, the Cost-Distance Submodel is more influential than Slope alone, which indicates that cumulative impediments (costs) to harvesters to reach a site in the form of slope are more likely to affect the suitability of a given area for bark harvesting compared to specific site (cell) level conditions (Table 3). LiDAR-derived Elevation and CHM were also important contributors to predict CMT suitability. Slope, Aspect, Canopy Cover of both yellow-cedar and redcedar, however, were not highly influential in predicting CMT suitability. Our final model based on best-fit (principal) weights provides a continuous heatmap of CMT suitability throughout the study area (Fig. 3), and performed well to identify known CMT occurrence from both recent surveys and from RAAD (Fig. 4). Finally, by comparing our principal weights CMT model with the commercial timber harvesting landbase, we find that the proportion of high suitability areas is much greater within the THLB than outside.
The model provides a vehicle to combine local and Indigenous knowledge with biophysical data to collectively provide evidence-based predictions for the occurrence of culturally important features. Accordingly, the model illustrates how biocultural stewardship can draw on both ancient knowledge and contemporary computing approaches. We also acknowledge several limitations and trade-offs. The development of the KX field surveys employed a nonrandom sampling strategy, owing to logistical constraints and the difficulty in conducting grid- or census-based methods in this largely roadless landscape. Clearly, if financial and time resources are abundant, census-based surveys provide a better approach for many suitability modelling endeavours (Muir and Moon 2000; Store and Jokimäki 2003). Additionally, although the contributions of existing data from previous archaeological survey efforts greatly increased our sample size and provided valuable occurrence data for regions that were difficult to access during our field season, the accuracy of the georeferenced points was unknown. Confidence in the models could be improved by ground-truthing the spatial accuracy of a subset of the georeferenced CMT locations from RAAD as well as information derived from satellite imagery. Lastly, incorporating community-based interviews that more explicitly informed the models on harvester preferences and laws might improve model performance.
The predictive power of the cultural variables in our model demonstrates the importance of incorporating local perspectives in suitability modelling of biocultural indicators. For example, other CMT potential models often use a “distance from shoreline” variable, where our work benefitted from Indigenous Knowledge and local perspectives to create a finer-tuned Cost-Distance Submodel. The design of both the Cost-Distance Submodel and the Distance from Known Habitation Sites variables were led and informed by local and Indigenous Knowledge to ensure that our approach offered a more detailed and culturally resonate perspective than the simple “distance from shoreline” variable. The somewhat lower standard deviation for our Cost-Distance Submodel suggests its use also provided less uncertainty than Elevation. Importantly these variables reflect the long history of KX biocultural stewardship and offered key information to predict the suitability of CMT locations.
Although we expected that the biophysical variables would have strong predictive utility for CMTs, variables such as Canopy Cover of yellow-cedar and redcedar performed relatively poorly. The poor performance of the redcedar variable could be attributed to a possible correlation between CMTs and mixed species stand composition or inaccuracy in the satellite-derived species composition data (Fleming et al. 2004). The low sample size of yellow-cedar CMT observations (n = 33) used in the CMT–MCE, paired with the relatively small amount of area with dominant yellow-cedar canopy, could have contributed to the minimal predictive utility of yellow-cedar in modelling CMT suitability. The low sample size of yellow-cedar CMTs could be due to challenges in surveying higher elevation yellow-cedar stands. Moreover, most CMT observations from RAAD in our study area come from surveys conducted below 250 m. Although we used a helicopter during our field surveys to access higher elevation areas (conducting ∼15 km of survey above 300 m) to address this potential bias, we did not locate any CMTs above 400 m. Future studies may benefit from modelling yellow-cedar CMTs separately from redcedar CMTs due to their potential divergence in suitable site conditions (Stafford 2017).
Other than Elevation, biophysical variables had modest predictive utility. The CHM had the most predictive power of these variables, indicating that although taller trees are increasingly suitable (Table 3), a variety of tree heights can provide adequate conditions for CMTs. Further, a diversity of canopy layers (which might lower the median crown height per 25 m2 cell) likely creates suitable conditions for trees with fewer branches below the crown, thus offering a bole surface relatively unobstructed by limbs from which to pull bark (Sutherland et al. 2016; Benner et al. 2019). Additionally, the mean suitability scores for Slope and Aspect fell below that of the equal weights mean suitability score, indicating their modest predictive power (Table 3). The standard deviations for Slope and Aspect were among the highest in the models, indicating that CMT suitability occurs across a wide range of slopes and aspects. This latter observation is often a reason for omitting CMT survey efforts in steep terrain in other regions, which is not supported by this model.
We quantified important spatial patterns between CMT suitability and the commercial THLB polygons, the latter comprising ∼27% of the study area. We found that moderate and high suitability cells account for more than 87% of the area within the THLB (Table 6). Further, the relative proportion of high suitability cells within the THLB (13%) is 51% greater than the amount outside the THLB (9%; Table 6). These patterns, evident at fine to large scales, illustrate the utility of this model (Fig. 3) in mitigating potential impacts to existing and future CMTs from commercial timber harvest. For example, forestry planning can identify “hotspots” of CMT suitability (at multiple scales) before onsite surveying and engineering is considered. We caution, too, that our (and any) model cannot replace the utility of ground-based surveys. Additionally, despite archaeological surveys within a cutblock prior to cutting, the undocumented removal of CMTs can still occur because old CMT scars can completely heal over making them difficult to identify prior to harvest (Earnshaw 2017, 2019). Given the role of CMTs as biocultural indicators the principal weights model could also be used as a tool for improving archaeological predictive survey for these healed-over CMTS and other archaeological site types (Gallagher and Josephs 2008; Verhagen and Whitley 2012; Hesse 2013).
Although suitability models cannot replace ground-based surveys, they offer insight into how impacts to culturally significant features and areas can be minimized as part of forest and other natural resource management. One potential method for applying our suitability model would be to overlay proposed cutblock polygons (smaller spatial units compared to the THLB polygons) to evaluate the mean, maximum, and range of suitability scores within the boundaries. In the context of Indigenous governments like the KX, this could provide Lands Managers and the communities they serve valuable information about the potential risks of developing an area before plans proceed, and investments are made into cutblock engineering, etc. For example, if development proceeds in areas of high suitability, proposed blocks can be surveyed intensively (at 100% survey coverage) prior to development and followed by a post harvest assessment to identify completely healed-over CMT scars (Earnshaw 2016, 2019). This model can also be applied during the Landscape Reserve Design process, which is a planning exercise to implement the goals and regulations set out in the GBR LUO for both biodiversity and culturally significant resources. Further, in the GBR and abroad, modelling biocultural indicators may also help support the design and implementation of Indigenous Protected Areas (Murray and King 2012).
Beyond the GBR, locally informed models such as ours could be used to help identify biocultural diversity hotspots or facilitate bringing cultural values into national or global strategies for biodiversity conservation and protection in the context of reconciliation (Diggon et al. 2020; Supernant 2020; Wong et al. 2020). Suitability models of other biocultural indicators and culturally significant species or values could also be incorporated as part of community harvest management plans to coordinate contemporary resource use, protected area designation, and management or comprehensive assessments for nonextractive industries such as tourism (e.g., Lemelin et al. 2015; Kitasoo/Xai’xais 2019). Scaling up, multiple biocultural suitability models could be combined in a biocultural ecosystem services modelling framework (Nelson et al. 2009; Klain and Chan 2012; Pert et al. 2015). In this way, stewardship managers can identify areas with a high diversity of cultural ecosystem services (e.g., heritage and marine/terrestrial resources). Further, this biocultural modelling approach could also be incorporated into species-at-risk management planning so that conservation efforts can have compounding benefits for both biodiversity and the Indigenous communities that have strong place-based ties to key habitat areas and species (Westwood et al. 2019).
Importantly this model is the first locally informed continuous spatial representation of historical and contemporary landscape use (cedar bark harvesting) to be applied in the context commercial forestry in Kitasoo/Xai’xais Territory. Further, we provide a unique example of how local and Indigenous Knowledge can be incorporated both in the form of input data as well as throughout the spatial modelling process. Our unique approach that blends Indigenous and place-based knowledge with archaeological inventory data to model aspects of the cultural landscape provides one path to weave Indigenous-led approaches with western science approaches to EMM. By linking people and place to a biocultural indicator, our model has facilitated the implementation of Indigenous-led stewardship in the context of co-governance. In doing so, our approach provides a potentially generalizable strategy to confront—and overcome—common political barriers to implementation of biodiversity conservation projects, such as the lack of recognition and meaningful incorporation of Indigenous cultural values during planning and monitoring.
Conclusion
Biocultural EMM offers an emerging domain that couples local values and scientific approaches to overcome common political barriers to biodiversity conservation by protecting enduring linkages between people and nature. Biocultural indicators, such as culturally modified trees, offer a focal point where multiple knowledge sources, institutions, and agencies can engage to design and implement EMM effectively. Our case study illustrates how a locally led suitability modelling approach guided and enabled the incorporation of locally informed input predictor variables. Accordingly, we demonstrate how local and Indigenous Knowledge and values can direct both the development and application of science as well as guide the implementation of biocultural EMM decisions in co-governance scenarios. However, we also highlight the need to re-evaluate the equity of co-governance agreements to better recognize, uphold, and apply Indigenous ways of knowing in ways we do here and beyond (Reid et al. 2020). Respecting and actively supporting Indigenous authority, laws, institutions, values, protocols, and stewardship practice are critical to achieving biodiversity conservation and sustainable development goals in Canada and abroad.
Acknowledgements
We thank the KX Resource Stewardship Authority and specifically Douglas Neasloss and Evan Loveless for their support and guidance of this research. We also thank our colleagues in the field including Stephen Neasloss, Sarein Basi-Primeau, Chantal Pronteau, John May, Mitch Robinson, Santana Edgar, Krista Duncan, Sam Harrison, and Rosie Child. We thank Andra Forney, Nick Reynolds, Blake Evans, and Richard Hebda for their guidance and training. We also thank Jacob Earnshaw, Robert Gustas, Jordan Benner, and Ken Lertzman for their guidance and input that greatly improved this work. Finally, we thank Diana Cooper with the British Columbia Archaeology Branch for her assistance with the RAAD database. B.C. DeRoy was supported by Raincoast Conservation Foundation Graduate Fellowship, Vernon Brown and C.N. Service by the KX Stewardship Authority and Spirit Bear Research Foundation, M. Leclerc by Raincoast Conservation Foundation and Fonds de Recherche Nature et Technologies (FRQNT #255026), I. McKechnie by Hakai Institute and NSERC-DG (#531246), and C.T Darimont by Raincoast Conservation and SkyeMikko Foundations as well as the National Science and Engineering Research Council (NSERC) Discovery Grant (#435683). We also thank the anonymous reviewers who considerably improved this manuscript.
References
Arcas Consulting. 1999. The archaeological overview assessment of the Central Coast of British Columbia. Report on file with the Archaeology Branch, Victoria, British Columbia.
Artelle K, Stephenson J, Bragg C, Housty J, Housty W, Kawharu M, et al. 2018. Values-led management: the guidance of place-based values in environmental relationships of the past, present, and future. Ecology and Society, 23(3): 35.
Ban NC, Frid A, Reid M, Edgar B, Shaw D, and Siwallace P. 2018. Incorporate Indigenous perspectives for impactful research and effective management. Nature Ecology & Evolution, 2(11): 1680–1683.
Ban NC, Wilson E, and Neasloss D. 2020. Historical and contemporary indigenous marine conservation strategies in the North Pacific. Conservation Biology, 34(1): 5–14.
Benner J, Knudb A, Nielsen J, Krawchuk M, and Lertzman K. 2019. Combining data from field surveys and archaeological records to predict the distribution of culturally important trees. Diversity and Distributions, 25(9): 1375–1387.
Bennett NJ. 2016. Using perceptions as evidence to improve conservation and environmental management. Conservation Biology, 30(3): 582–592.
British Columbia Archaeology Branch. 1996. Heritage Conservation Act. c. 187 [online]: Available from bclaws.ca/civix/document/id/complete/statreg/96187_01.
British Columbia Archaeology Branch. 2001. Culturally modified trees of British Columbia: a handbook to the identification and recording of culturally modified trees. B.C. Ministry of Forests, Queens Printer, Victoria, British Columbia (version 2.0). On File at the British Columbia Archaeology Branch, Victoria, British Columbia.
British Columbia Archaeology Branch. 2018. Remote access to archaeological data (RAAD) [online]: Available from for.gov.bc.ca/archaeology/archaeology_professionals/resources.htm.
British Columbia Ministry of Forests, Lands and Natural Resource Operations. 2016. Great Bear Rainforest order. British Columbia Ministry of Forests, Lands and Natural Resource Operations, Victoria, British Columbia [online]: Available from www2.gov.bc.ca/assets/gov/farming-natural-resources-and-industry/forestry/timber-pricing/coast-timber-pricing/maps-and-graphics/great_bear_rainforest_order_-_jan_21_2016.pdf.
British Columbia Ministry of Forests, Lands and Natural Resource Operations. 2018. VRI 1 Forest Vegetation Composite Polygons and Rank 1 Layer [online]: Available from catalogue.data.gov.bc.ca/dataset/vri-forest-vegetation-composite-polygons-and-rank-1-layer.
Brown F, and Brown K. 2009. Staying the course, staying alive—coastal First Nations fundamental truths: biodiversity, stewardship and sustainability. Biodiversity BC, Victoria, British Columbia [online]: Available from biodiversitybc.org/assets/Default/BBC_Staying_the_Course_Web.pdf.
Caillon S, Cullman G, Verschuuren B, and Sterling EJ. 2017. Moving beyond the human-nature dichotomy through biocultural approaches: including ecological well-being in resilience indicators. Ecology and Society, 22(4): 27.
Caverley N, Lyall A, Pizzirani S, and Bulkan J. 2020. Articulating Indigenous rights within the inclusive development framework: an assessment of forest stewardship policies and practices in British Columbia, Canada. Society & Natural Resources, 33(1): 25–45.
De Groot RS, Alkemade R, Braat L, Hein L, and Willemen L. 2010. Challenges in integrating the concept of ecosystem services and values in landscape planning, management and decision making. Ecological Complexity, 7(3): 260–272.
DeRoy BC, Darimont CT, and Service CN. 2019. Biocultural indicators to support locally led environmental management and monitoring. Ecology and Society, 24(4): 21.
Diggon S, Bones J, Short CJ, Smith JL, Dickinson M, Wozniak K, et al. 2020. The Marine Plan Partnership for the North Pacific Coast—MaPP: a collaborative and co-led marine planning process in British Columbia. Marine Policy, 104065, in press, corrected proof, available online 29 October 2020.
Earnshaw JK. 2017. “The trees you do not need…”: culturally modified forests and the Tsilhqot’in ruling. The Midden, 47(3): 2–11.
Earnshaw JK. 2019. Cultural forests in cross section: clear-cuts reveal 1,100 years of bark harvesting on Vancouver Island, British Columbia. American Antiquity, 84(3): 1–15.
Earnshaw JTK. 2016. Cultural forests of the Southern Nuu-chah-nulth: historical ecology and salvage archaeology on Vancouver Island’s West Coast. M.A. thesis, University of Victoria, Victoria, British Columbia. 257 p.
Eastman JR. 1999. Multi-criteria evaluation and GIS. Geographical Information Systems, 1(1): 493–502.
Elith J, and Leathwick JR. 2009. Species distribution models: ecological explanation and prediction across space and time. Annual Review of Ecology, Evolution, and Systematics, 40: 677–697.
Fleming KK, Didier KA, Miranda BR, and Porter WF. 2004. Sensitivity of a white‐tailed deer habitat‐suitability index model to error in satellite land‐cover data: implications for wildlife habitat‐suitability studies. Wildlife Society Bulletin, 32(1): 158–168.
Franklin J. 2010. Moving beyond static species distribution models in support of conservation biogeography. Diversity and Distributions, 16(3): 321–330.
Gallagher JM, and Josephs RL. 2008. Using LiDAR to detect cultural resources in a forested environment: an example from Isle Royale National Park, Michigan, USA. Archaeological Prospection, 15(3): 187–206.
Garibaldi A, and Turner N. 2004. Cultural keystone species: implications for ecological conservation and restoration. Ecology and Society, 9(3): 1 [online]: Available from jstor.org/stable/10.2307/26267680.
Garrick D. 1998. Shaped cedars and cedar shaping. Western Canada Wilderness Committee, Vancouver, British Columbia.
Gavin MC, McCarter J, Mead A, Berkes F, Stepp JR, Peterson D, et al. 2015. Defining biocultural approaches to conservation. Trends in Ecology & Evolution, 30(3): 140–145.
Gavin MC, McCarter J, Berkes F, Mead A, Sterling EJ, Tang R, et al. 2018. Effective biodiversity conservation requires dynamic, pluralistic, partnership-based approaches. Sustainability, 10(6): 1846.
Guisan A, Tingley R, Baumgartner JB, Naujokaitis-Lewis I, Sutcliffe PR, Tulloch AI, et al. 2013. Predicting species distributions for conservation decisions. Ecology Letters, 16(12): 1424–1435.
Guisan A, Thuiller W, and Zimmermann NE. 2017. Habitat suitability and distribution models: with applications in R. Cambridge University Press.
Gustas R, and Supernant K. 2017. Least cost path analysis of early maritime movement on the Pacific Northwest Coast. Journal of Archaeological Science, 78: 40–56.
Hesse R. 2013. The changing picture of archaeological landscapes: lidar prospection over very large areas as part of a cultural heritage strategy. In Interpreting archaeological topography: 3D data, visualisation and observation. Occasional Publication of the AARG. Vol. 5. Edited by RS Opitz and DC Cowley. Oxbow Books, Oxford, England. pp. 171–183.
Heuvelink GB. 1998. Error propagation in environmental modelling with GIS. CRC Press.
Karpiak M. 2003. Modelling Nuu-chah-nulth land use: the cultural landscape of Clayoquot Sound. M.A. thesis, Simon Fraser University, Burnaby, British Columbia. 152 p.
Kitasoo/Xai’xais. 2018. Kitasoo/Xai’xais Cultural Heritage Database. On file with Kitasoo/Xai’xais Stewardship Authority, Klemtu, British Columbia.
Kitasoo/Xai’xais. 2019. Cultural Feature Inventory Standards Protocol. Kitasoo/Xai’xais Stewardship Authority, Klemtu, British Columbia [online]: Available from klemtu.com/stewardship/land-use-planning-management.
Klain SC, and Chan KM. 2012. Navigating coastal values: participatory mapping of ecosystem services for spatial planning. Ecological Economics, 82: 104–113.
Lemelin RH, Koster R, and Youroukos N. 2015. Tangible and intangible indicators of successful aboriginal tourism initiatives: a case study of two successful aboriginal tourism lodges in Northern Canada. Tourism Management, 47: 318–328.
Lepofsky D, and Armstrong CG. 2018. Foraging new ground: documenting ancient resource and environmental management in Canadian Archaeology. Canadian Journal of Archaeology, 42: 57–73.
Lepofsky D, Armstrong CG, Greening S, Jackley J, Carpenter J, Guernsey B, et al. 2017. Historical ecology of cultural keystone places of the Northwest Coast. American Anthropologist, 119(3): 448–463.
Lertzman K. 2009. The paradigm of management, management systems, and resource stewardship. Journal of Ethnobiology, 29(2): 339–359.
Mackie Q, Fedje D, and McLaren D. 2018. Archaeology and sea level change on the British Columbia coast. Canadian Journal of Archaeology, 42: 74–91.
McLaren D, Rahemtulla F, and Fedje D. 2015. Prerogatives, sea level, and the strength of persistent places: archaeological evidence for long-term occupation of the Central Coast of British Columbia. BC Studies: The British Columbian Quarterly, No. 187: 155–191.
Muir RJ, and Moon H. 2000. Sampling culturally modified tree sites. Report prepared for The Ministry of Forests, Aboriginal Affairs Branch. pp. 1–43 [online]: Available from for.gov.bc.ca/ftp/archaeology/external/!publish/web/sampling_culturally_modified_trees.pdf.
Murray G, and King L. 2012. First Nations values in protected area governance: Tla-o-qui-aht tribal parks and Pacific Rim National Park Reserve. Human Ecology, 40: 385–395.
Nelson E, Mendoza G, Regetz J, Polasky S, Tallis H, Cameron D, et al. 2009. Modeling multiple ecosystem services, biodiversity conservation, commodity production, and tradeoffs at landscape scales. Frontiers in Ecology and the Environment, 7(1): 4–11.
Newing H. 2010. Bridging the gap: interdisciplinarity, biocultural diversity and conservation. In Nature and culture: rebuilding lost connections. Edited by S Pilgrim and J Pretty. Routledge. pp. 23–40.
Oliver J. 2007. Beyond the water’s edge: towards a social archaeology of landscape on the Northwest Coast. Canadian Journal of Archaeology, 31(1): 1–27 [online]: Available from jstor.org/stable/41103280.
Pert PL, Hill R, Maclean K, Dale A, Rist P, Schmider J, et al. 2015. Mapping cultural ecosystem services with rainforest aboriginal peoples: integrating biocultural diversity, governance and social variation. Ecosystem Services, 13: 41–56.
Pinkerton E, Salomon AK, and Dragon F. 2019. Reconciling social justice and ecosystem-based management in the wake of a successful predator reintroduction. Canadian Journal of Fisheries and Aquatic Sciences, 76(6): 1031–1039.
Polfus JL, Heinemeyer K, Hebblewhite M, and Taku River Tlingit First Nation. 2014. Comparing traditional ecological knowledge and western science woodland caribou habitat models. The Journal of Wildlife Management, 78(1): 112–121.
Polfus JL, Manseau M, Simmons D, Neyelle M, Bayha W, Andrew F, et al. 2016. Łeghágots’ enetę (learning together): the importance of indigenous perspectives in the identification of biological variation. Ecology and Society, 21(2): 18.
Pungetti G. 2013. Biocultural diversity for sustainable ecological, cultural and sacred landscapes: the biocultural landscape approach. In Landscape ecology for sustainable environment and culture. Edited by B Fu and BK Jones. Springer, Dordrecht, the Netherlands. pp. 55–76.
Pyke M, Toussaint S, Close P, Dobbs R, Davey I, George K, et al. 2018. Wetlands need people: a framework for understanding and promoting Australian indigenous wetland management. Ecology and Society, 23(3): 43.
Reid AJ, Eckert LE, Lane JF, Young N, Hinch SG, Darimont CT, et al. 2020. “Two‐eyed seeing”: an Indigenous framework to transform fisheries research and management. Fish and Fisheries, in press, corrected proof, available online 29 October 2020.
Rodríguez JP, Brotons L, Bustamante J, and Seoane J. 2007. The application of predictive modelling of species distribution to biodiversity conservation. Diversity and Distributions, 13(3): 243–251.
Rozzi R, Massardo F, Anderson CB, Heidinger K, and Silander JA Jr. 2006. Ten principles for biocultural conservation at the southern tip of the Americas: the approach of the Omora Ethnobotanical Park. Ecology and Society, 11(1): 43.
Saaty TL. 1980. The analytic hierarchy process. McGraw-Hill, New York, New York. 324 p.
Salomon AK, Lertzman K, Brown K, Wilson KB, Secord D, and McKechnie I. 2018. Democratizing conservation science and practice. Ecology and Society, 23(1): 44.
Stafford J, and Maxwell J. 2006. The Text Is in the Trees: Incorporating Indigenous Forest Practices into the Archaeological Landscape of the Northwest Coast. Paper presented at the Annual Meeting of the Canadian Archaeological Association, Toronto, Ontario.
Stafford J. 2017. Taphonomy of culturally modified forests in coastal BC: notes on identification and recording of bark harvested cedar trees. The Midden, 47: 41–52.
Sterling EJ, Filardi C, Toomey A, Sigouin A, Betley E, Gazit N, et al. 2017a. Biocultural approaches to well-being and sustainability indicators across scales. Nature Ecology & Evolution, 1(12): 1798–1806.
Sterling EJ, Ticktin T, Morgan TKK, Cullman G, Alvira D, Andrade P, et al. 2017b. Culturally grounded indicators of resilience in social-ecological systems. Environment and Society, 8(1): 63–95.
Stewart H. 1984. Tree of Life to the Northwest Coast Indians. Douglas and McIntyre, Vancouver, British Columbia.
Store R, and Jokimäki J. 2003. A GIS-based multi-scale approach to habitat suitability modeling. Ecological Modelling, 169(1): 1–15.
Store R, and Kangas J. 2001. Integrating spatial multi-criteria evaluation and expert knowledge for GIS-based habitat suitability modelling. Landscape and Urban Planning, 55(2): 79–93.
Stryd AH, and Eldridge M. 1993. CMT archaeology in British Columbia: the Meares Island studies. BC Studies: the British Columbian Quarterly, 99: 184–234.
Supernant K. 2017. Modeling Métis mobility? Evaluating least cost paths and indigenous landscapes in the Canadian west. Journal of Archaeological Science, 84: 63–73.
Supernant K. 2020. Grand Challenges No. 1—truth and reconciliation: archaeological pedagogy, Indigenous histories, and reconciliation in Canada. Journal of Archaeology and Education, 4: 1–23 [online]: Available from digitalcommons.library.umaine.edu/jae/vol4/iss3/2.
Sutherland IJ, Gergel SE, and Bennett EM. 2016. Seeing the forest for its multiple ecosystem services: indicators for cultural services in heterogeneous forests. Ecological Indicators, 71: 123–133.
Turner NJ, Ari Y, Berkes F, Davidson-Hunt I, Ertug ZF, and Miller A. 2009. Cultural management of living trees: an international perspective. Journal of Ethnobiology, 29(2): 237–270.
United Nations General Assembly. 2007. Declaration on the rights of Indigenous peoples. United Nations General Assembly, New York, New York [online]: Available from un.org/development/desa/indigenouspeoples/declaration-on-the-rights-of-indigenous-peoples.html.
Verhagen P, and Whitley TG. 2012. Integrating archaeological theory and predictive modeling: a live report from the scene. Journal of Archaeological Method and Theory, 19(1): 49–100.
Verschuuren B. 2012. Integrating biocultural values in nature conservation: perceptions of culturally significant sites and species in adaptive management. In Sacred species and sites: advances in biocultural conservation. Edited by G Pungetti, G Oviedo, and D Hooke. Cambridge University Press. pp. 231–246.
von der Porten S, Ota Y, Cisneros-Montemayor A, and Pictou S. 2019. The role of Indigenous resurgence in marine conservation. Coastal Management, 47(6): 527–547.
Wali A, Alvira D, Tallman D, Ravikumar A, and Macedo M. 2017. A new approach to conservation: using community empowerment for sustainable well-being. Ecology and Society, 22(4): 6.
Walker WS, Gorelik SR, Baccini A, Aragon-Osejo JL, Josse C, Meyer C, et al. 2020. The role of forest conversion, degradation, and disturbance in the carbon dynamics of Amazon indigenous territories and protected areas. Proceedings of the National Academy of Sciences of the United States of America, 117(6): 3015–3025.
Westwood AR, Otto SP, Mooers A, Darimont C, Hodges KE, Johnson C, et al. 2019. Protecting biodiversity in British Columbia: recommendations for developing species at risk legislation. FACETS, 4(1): 136–160.
Wong C, Ballegooyen K, Ignace L, Johnson M, and Swanson H. 2020. Towards reconciliation: 10 Calls to Action to natural scientists working in Canada. FACETS, 5(1): 769–783.
Zurba M, Beazley KF, English E, and Buchmann-Duck J. 2019. Indigenous Protected and Conserved Areas (IPCAs), Aichi Target 11 and Canada’s Pathway to Target 1: focusing conservation on reconciliation. Land, 8(1): 10.
Supplementary material
Supplementary Material 1 (DOCX / 739 KB)
- Download
- 739.58 KB
Information & Authors
Information
Published In
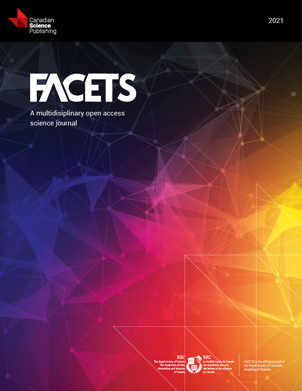
FACETS
Volume 6 • Number 1 • January 2021
Pages: 465 - 489
Editor: Karen Beazley
History
Received: 11 June 2020
Accepted: 14 December 2020
Version of record online: 6 April 2021
Notes
This paper is part of a collection titled “Conservation in Canada: identifying and overcoming barriers”.
Copyright
© 2021 DeRoy et al. This work is licensed under a Creative Commons Attribution 4.0 International License (CC BY 4.0), which permits unrestricted use, distribution, and reproduction in any medium, provided the original author(s) and source are credited.
Data Availability Statement
All relevant data are within the paper and in the Supplementary Material.
Key Words
Sections
Subjects
Authors
Author Contributions
BCD, VB, CNS, CB, IM, and CTD conceived and designed the study.
BCD, CNS, and CTD secured funding.
BCD, VB, and CNS performed the experiments/collected the data.
BCD, ML, and CB analyzed and interpreted the data.
All drafted or revised the manuscript.
Competing Interests
The authors have declared that no competing interests exist.
Metrics & Citations
Metrics
Other Metrics
Citations
Cite As
Bryant C. DeRoy, Vernon Brown, Christina N. Service, Martin Leclerc, Christopher Bone, Iain McKechnie, and Chris T. Darimont. 2021. Combining high-resolution remotely sensed data with local and Indigenous Knowledge to model the landscape suitability of culturally modified trees: biocultural stewardship in Kitasoo/Xai’xais Territory. FACETS.
6(): 465-489. https://doi.org/10.1139/facets-2020-0047
Export Citations
If you have the appropriate software installed, you can download article citation data to the citation manager of your choice. Simply select your manager software from the list below and click Download.
Cited by
1. Transforming conservation in Canada: shifting policies and paradigms